Introduction
The antioxidant activity of foods is important for both their shelf life and protection from oxidative damage in the human body. Increased oxidative stress is involved in the onset of most age/diet-related chronic diseases( Reference Benzie 1 , Reference Ames, Shigenaga and Hagen 2 ). Therefore, antioxidant activity has been considered an essential nutritional property( Reference Serafini and Peluso 3 ). Its expression within the human body depends on the structure of the food matrix and density of food antioxidants, which both depend on the processing conditions, ultimately having an impact on the antioxidant bioavailability. Many in vitro assays have been proposed to estimate the antioxidant capacity of food. However, each method is based on different oxidoreduction reactions, yielding heterogeneous antioxidant potentials, as observed with the method used for milk( Reference Cloetens, Panee and Akesson 4 ).
Overall, the antioxidant potential has been more widely studied in plant-based foods than in animal-based foods( Reference Wu, Beecher and Holden 5 , Reference Carlsen, Halvorsen and Holte 6 ). Probably the main reason for this is the lower average antioxidant potential of animal-based foods as compared with plant-based foods, i.e. on average 64-fold less( Reference Carlsen, Halvorsen and Holte 6 ). Thus, although being an important part of various diets worldwide, the antioxidant potential of dairy products has rarely been systematically measured( Reference Carlsen, Halvorsen and Holte 6 , Reference Grażyna, Hanna and Adam 7 ). The most comprehensive study is that by Carlsen et al.( Reference Carlsen, Halvorsen and Holte 6 ) who measured antioxidant potential of eighty-six dairy products among more than 3100 foods, beverages, spices, herbs and supplements used worldwide. In addition, in epidemiological studies, the antioxidant potential of dairy products has not been investigated as the primary explanation for some of their protective effects, for example, against weight gain, type 2 diabetes, some CVD and some cancers( Reference Fardet and Boirie 8 ).
Dairy products contain antioxidant compounds in varying proportions depending on the matrix type (i.e. milks, yoghurts, fermented milks and cheeses) and processing (i.e. mechanical, thermal and fermentative). These compounds include both lipophilic and hydrophilic antioxidants: proteins (especially casein), peptides, antioxidant enzymes (i.e. superoxide dismutase (SOD), catalase and glutathione peroxidase), conjugated linoleic acid (CLA), coenzyme Q10, lactoferrin, vitamins (C, E, A and D3), carotenoids, some minerals and some trace elements( Reference Grażyna, Hanna and Adam 7 , Reference Lindmark-Månsson and Åkesson 9 ) (Fig. 1). According to Grażyna et al.( Reference Grażyna, Hanna and Adam 7 ), the antioxidants in milk act in synergy by forming an antioxidant network, imparting high antioxidant potential to milk and effectively protecting milk fat against oxidation. In addition, although this is not a direct focus of the present review, the effect of the animal origin, genetic type (i.e. breed)( Reference Perna, Intaglietta and Simonetti 10 ), feed (for example, composition, quantity, and complementation, such as the fat quality) and pasture conditions (for example, organic v. conventional, seasonal variations) on the antioxidant potential of milk has been repeatedly studied( Reference Perna, Intaglietta and Simonetti 10 – Reference Perna, Intaglietta and Simonetti 23 ).

Fig. 1 Main milk antioxidants.
Overall, the antioxidant potential of dairy products has been mostly studied in vitro, with very few in vivo animal and human studies. However, this is also true for other foods such as cereals, whose antioxidant potential has been extensively studied in vitro but rarely studied directly in human subjects( Reference Fardet, Rock and Rémésy 24 ). The main issue is the important difference between in vitro and in vivo antioxidant potentials of foods, unless the in vitro potential is directly measured in digestive extracts, as demonstrated for cereal-based products( Reference Fardet, Rock and Rémésy 24 , Reference Pérez-Jiménez and Saura-Calixto 25 ).
The objectives of the present narrative review were therefore to synthesise data on the antioxidant potential of dairy products, both in vitro and in animals and humans, as well as for each main dairy product (i.e. milks, yoghurts, fermented milks and cheeses), and to describe, when possible, the influence of processing, especially skimming, thermal treatment, fermentation, probiotic supplementation and storage, on the antioxidant potential of these foods. We chose to organise the literature results into three parts according to in vitro, animal and human studies because there is generally a relevant difference between what is measured in vitro using the typical antioxidant assays and the real in vivo antioxidant impact, which does not necessarily reflect the in vitro measurements, as we previously discussed for cereal-based foods( Reference Fardet, Rock and Rémésy 24 ).
In vitro studies
Dairy products (among food groups)
The literature indicates that dairy products may be a source of antioxidants( Reference Carlsen, Halvorsen and Holte 6 ), notably via their protein fraction( Reference Grażyna, Hanna and Adam 7 ). Comparing the results of one study with those of another on the basis of the same antioxidant assay used to measure the total antioxidant capacity per g of solid food( Reference Wu, Beecher and Holden 5 ) and ml of milk( Reference Cloetens, Panee and Akesson 4 ) can lead to some interesting comparisons, such as milk exhibiting a total antioxidant capacity of the same magnitude as those of grain products, fruit and vegetable juices, and some solid fruit and vegetable foods (Table 1). For example, the antioxidant capacity of commercial ultra-high temperature (UHT) cows’ milk is approximately 13 μmol Trolox equivalents (TE)/ml (oxygen radical absorbance capacity (ORAC) assay)( Reference Cloetens, Panee and Akesson 4 ), while that of plant-based foods, except dried fruits and spices, is in the range of 1·2–179·4 μmol TE/g (ORAC assay), with vegetables and fruits globally showing a wider range of antioxidant potentials than dairy products for a similar water content( Reference Wu, Beecher and Holden 5 ). Others reported that dairy products exhibit the lowest antioxidant content, with eggs and seafood, among twenty-four food categories. For example, fruits and fruits juice – except berries – have 9-fold higher antioxidant content than dairy products( Reference Carlsen, Halvorsen and Holte 6 ).
Table 1 Antioxidant potential of milk compared with that of plant-based food groupsFootnote * (Ranges)

UHT, ultra-high temperature; ORAC, oxygen radical absorbance capacity.
* Determined from the ORAC assay. Adapted from Cloetens et al.( Reference Cloetens, Panee and Akesson 4 ) for milk products and Wu et al.( Reference Wu, Beecher and Holden 5 ) for other food groups.
† In μmol Trolox equivalents/ml.
Among dairy products Unal( Reference Unal 26 ) compared the antioxidant activity of different types of milk (n 14, including infant formula), yoghurt (n 14, including a probiotic yoghurt), fresh cream cheese (n 12) and kefir (n 6) purchased from a local Turkish supermarket based on the 2,2-diphenyl-1-picrylhydrazyl (DPPH) assay and Fe-chelating activity. Concerning milk, the highest antioxidant activity was observed for regular whole UHT milk, which has the highest content of fat, and semi-skimmed UHT milk enriched with polyunsaturated n-3 fatty acids. According to the author, this could be due to the reactivity of fat-soluble antioxidants and to the fat globule membrane proteins( Reference Lindmark-Månsson and Åkesson 9 ). However, three milks with the same fat content (3 %, whole, UHT) had significantly different antioxidant activities, an effect probably attributable to the various conditions of the thermal processes used by manufacturers, as stated by the authors. But other factors could have been at play, such as the season of milk production or milk source.
Semi-skimmed yoghurt exhibited significantly greater antioxidant activity than skimmed yoghurt. As expected, strawberry yoghurt has significantly higher antioxidant activity than plain yoghurt – both from the same manufacturer – which can be attributed to both the strawberries and probiotics. Indeed, an increase in antioxidant activity was detected after the addition of bifidobacteria to lactic acid bacteria in a fermented milk( Reference Wang, Yu and Chou 27 ). Creamy fresh cheeses generally have higher antioxidant activity, which can be attributed to their high levels of protein on a fresh-weight basis compared with other dairy products. Concerning the different samples of kefir, plain kefirs were different from each other in terms of antioxidant activity, which could be caused by their different compositions and processing conditions used by different manufacturers.
Milk
Antioxidants in milk
Studies on whey and deproteinised milk have shown that the major contributor to the total antioxidant capacity of whole milk is the casein fraction and that albumin is the major contributor to the total antioxidant capacity of whey protein( Reference Zulueta, Maurizi and Frigola 28 ). Another study compared the trapping of free radicals in milk, milk serum (obtained by centrifugation) and whey (obtained by acidification of skimmed milk) after subjecting the samples to size exclusion chromatography( Reference Clausen, Skibsted and Stagsted 29 ). As shown previously( Reference Chen, Lindmark-Mansson and Gorton 30 ), caseins contributed the most to the antioxidant activities measured by 2,2’-azino-bis(3-ethylbenzothiazoline-6-sulfonic acid) (ABTS) and ORAC assays (89 %). β-Lactoglobulin and α-lactalbumin contributed much less to the total antioxidant capacity, and in the low-molecular-weight fractions, urate and ascorbate were identified as antioxidants. This last result was confirmed by Cloetens et al. ( Reference Cloetens, Panee and Akesson 4 ) who published a review of the antioxidant capacity of milk (breast, animal and infant milks) with a focus on measurement methods. The major antioxidant in the low-molecular-weight fractions was identified as uric acid, an important milk antioxidant, which is also in agreement with previous data( Reference Østdal, Andersen and Nielsen 31 ). Uric acid contributes 60 % of the human plasma antioxidant capacity (ferric-reducing ability of plasma (FRAP) assay)( Reference Benzie and Strain 32 ). In milk, uric acid is formed from the ruminal breakdown of microbial nucleotides( Reference Giesecke, Ehrentreich and Stangassinger 33 , Reference Johnson, Harrison and Riley 34 ). Uric acid has both reducing and free radical-scavenging properties, which protect milk compounds from oxidative damage, and its antioxidative effect in milk is similar to that of ascorbate( Reference Østdal, Andersen and Nielsen 31 ). Other experiments have shown that the antioxidant activity of casein is mediated by its constitutive amino acids, while the antioxidant activity in whey is largely dependent on denaturation or partial hydrolysis and dominated by the free thiol groups of β-lactoglobulin( Reference Clausen, Skibsted and Stagsted 29 ). Clausen et al.( Reference Clausen, Skibsted and Stagsted 29 ) also concluded that the number of major contributors in milk to the free radical-scavenging activity (ABTS and ORAC assays) is relatively low.
The study of Cekic et al.( Reference Cekic, Demir and Baskan 35 ) took a similar approach: the antioxidant capacity of milk was measured using two assays, cupric-reducing antioxidant capacity (CUPRAC) and ABTS, because only these assays can assess the contribution of protein to the observed total antioxidant capacity. Different types of milk were tested: organic milk; pasteurised milk; UHT-processed fatty milk (3·0 % milk fat), low-fat milk (1·5 %) and skimmed milk (0·1 %); and homogenised milk in commercial packaging. For each milk type, three samples were analysed: defatted whole milk, a solution of milk protein and whey. Comparing the total antioxidant capacities of skimmed milk, casein, β-lactoglobulin, α-lactalbumin, and different protein-milk mixtures confirmed that milk proteins are responsible for a large part of the total antioxidant capacity of milk( Reference Cekic, Demir and Baskan 35 ).
Otherwise, β-lactoglobulin-depleted milk has been shown to possess approximately 50 % less antioxidant activity than raw skimmed milk( Reference Liu, Chen and Mao 36 ). Therefore, since β-lactoglobulin is very sensitive to thermal denaturation, dairy products should not be overheated to potentially maintain their antioxidant potential( Reference Liu, Chen and Mao 36 ). Although β-lactoglobulin is generally considered a mild antioxidant, the authors hypothesised that ‘it is possible that the free thiol groups in β-lactoglobulin or in other milk proteins act as a reducing agent to regenerate other antioxidants in the raw milk’( Reference Liu, Chen and Mao 36 ). Concerning the mechanisms, Liu et al.( Reference Liu, Chen and Mao 36 ) showed that β-lactoglobulin is a mild antioxidant (<vitamin E) but the conversion of β-lactoglobulin monomers into dimers is partly responsible for the in vitro LDL protection against oxidation induced by Cu. In addition, β-lactoglobulin substantially loses its antioxidant activity through crosslinking the thiol groups due to heating (100°C for 2 min) or through carboxymethylation to block the thiol groups, with Cys-121 playing an essential role.
Cervato et al.( Reference Cervato, Cazzola and Cestaro 37 ) evaluated the antioxidant properties of milk casein subunits (α-, β- and κ-caseins) in liposome models. All casein subunits can inhibit the peroxidation of arachidonic acid inserted in multilamellar liposomes of dipalmitoylphosphatidylcholine, but α-casein appears to have the greatest effect. This observation could be attributed to the Fe-chelation ability of phosphoserine residues, for which ten, five and one are present in α-, β- and κ-casein, respectively. Caseins appear to favour self-oxidation of Fe and thus inhibit lipid peroxidation( Reference Maubois and Leonil 38 ). However, these authors believe that mechanisms other than simple Fe chelation are involved in the antioxidant activity of casein, indicating that caseins exhibit a variety of mechanisms in their inhibition of peroxidation, involving both the specific properties of other proteins and characteristics of caseins. Finally, milk proteins and digested peptides might contribute to protecting the endothelial cells of the digestive tract against oxidative injury by free radicals.
Power et al.( Reference Power, Jakeman and FitzGerald 39 ) recently summarised laboratory results on antioxidant peptides, particularly those derived from milk, including their antioxidant potential measured in vitro and evaluated in vivo, mechanisms of action, bioavailability, potential applications and health benefits with a particular focus on ageing populations and regulatory requirements. One of the main important points is that these peptides, released during enzymic hydrolysis, are inactive when included in the ‘parent’ protein before digestion. The released peptides were shown to have the ability to scavenge free radicals, chelate metal ions, and/or inhibit lipid peroxidation. However, as explained by Power et al. ( Reference Power, Jakeman and FitzGerald 39 ), because of the variety of methods used to assess their antioxidant activity, data comparison from one study to another is difficult. Otherwise, the mechanisms of action of the antioxidant peptides derived from whey and casein have not been fully elucidated. Preliminary studies suggested that these mechanisms are related to the amino acid composition, sequence/structure and hydrophobicity, i.e. physico-chemical properties of the amino acids, of the peptides( Reference Chen, Muramoto and Yamauchi 40 ). In some cases, peptides derived from milk proteins have in vitro antioxidant activity similar to or even higher than that of chemical antioxidants (for example, butylated hydroxytoluene or butylated hydroxyanisole)( Reference Power, Jakeman and FitzGerald 39 ). Further, the authors gave an overview of the antioxidative peptides derived from cows’ and human milk protein following enzyme hydrolysis, including their amino acid sequences and the nature of the corresponding fragments. The authors concluded that such antioxidant peptides could find appropriate applications for specific populations such as the elderly because of the increased risk of sarcopenia and oxidative stress with ageing( Reference Power, Jakeman and FitzGerald 39 ).
The study by Woo et al.( Reference Woo, Jhoo and Kim 41 ) confirmed that hydrolysis of milk proteins is effective at increasing their antioxidant activity (ABTS assay). Specifically, the tryptic digest of casein showed the highest radical-scavenging activity, and the casein hydrolysates that passed through a membrane with a threshold of 3 kDa exhibited the highest antioxidant activity. In addition, Şanlidere Aloğlu( Reference Şanlidere Aloğlu 42 ) determined the antioxidant capacity of raw, pasteurised and sterilised milk before and after in vitro enzymic gastrointestinal digestion. The average values before digestion were 4·02, 4·47 and 4·18 mmol TE/g for raw, pasteurised and sterilised milk, respectively (ABTS assay), which significantly increased at the end of digestion to 11·13, 12·33 and 11·88 mmol TE/g, respectively. The main reason for this effect is that the antioxidant properties of casein and whey proteins were increased via hydrolysis in the presence of many digestive enzymes and that certain peptides released after the digestion of milk possessed an antioxidant capacity higher than that of other peptides( Reference Hernández-Ledesma, Dávalos and Bartolomé 43 – Reference Mandalari, Adel-Patient and Barkholt 45 ). In this study, the reported values obtained via the ABTS assay were mostly higher than those previously reported by Cloetens et al.( Reference Cloetens, Panee and Akesson 4 ) obtained via the ORAC assay, emphasising the importance of using similar assays for relevant comparisons. While the ORAC assay is based on H atom transfer mechanisms and measures the oxidative degradation of a fluorescent molecule after being mixed with free radical generators, the ABTS assay measures the electron- or H-donating properties of antioxidants, both hydrophilic and lipophilic, and is based on the conversion of ABTS to its radical cation, which is reactive towards most antioxidants. However, as most studies have been conducted in vitro, the extension to the real antioxidant potential of hydrolysed peptides in vivo will require appropriate studies in human volunteers.
If the protein fraction is the most powerful hydrophilic antioxidant in milk, the most active lipophilic antioxidant is CLA, which is uniquely found in milk, ruminant meat and eggs from chickens that have been fed CLA and is characterised by conjugated double bonds( Reference Grażyna, Hanna and Adam 7 ). In vitro, CLA was shown to inhibit maize oil lipid peroxidation induced by Cu( Reference Badr El-Din and Omaye 46 ) and to quench the stable radical DPPH•( Reference Fagali and Catala 47 ). In rats, CLA also protects structural lipids against free radicals and reactive oxygen species( Reference Palacios, Piergiacomi and Catala 48 , Reference Kim, Kim and Ahn 49 ). According to Dhiman et al. ( Reference Dhiman, Nam and Ure 50 ) the total CLA in dairy products and milk varies from 0·12 to 0·68 % of the total fat. Milk from organic farms has a higher CLA content than conventional milk due to the higher content of PUFA in cows’ diets in organic farming( Reference Bergamo, Fedele and Iannibelli 51 , Reference Rehberger, Bisig and Eberhard 52 ), and Ferreiro et al.( Reference Ferreiro, Gayoso and Rodriguez-Otero 53 ) report differences of +9·5 to 14·9 % in favour of organic milks. Lipophilic milk antioxidants also include vitamins A, D3 and E, but milk is not a very rich source of vitamins E or D3 ( Reference Grażyna, Hanna and Adam 7 ).
In addition to a protein fraction, antioxidant peptides, uric acid, CLA and lipophilic vitamins, milk also contains bioactive secondary phenolic compounds – such as equol, a metabolite of daidzein – which are formed from plant phenolics by the bacterial flora of the cow intestine( Reference Mustonen, Tuori and Saastamoinen 54 ). Tsen et al.( Reference Tsen, Siew and Lau 55 ) analysed five commercial whole milk, four commercial semi-skimmed milk and three commercial skimmed milk samples purchased from local supermarkets in Singapore and found that the antioxidant capacity and the ability to inhibit the formation of F2-isoprostanes increased with the concentrations of phenolic compounds and equol. However, differences in the antioxidant potential were not as marked as the differences in the equol concentration, indicating that other compounds contributed to the antioxidant potential. In addition, the equol and total phenolic concentrations were positively correlated with the milk fat concentration. While fresh full-cream milk contained 47·0 ng/ml equol and 0·70 μg gallic acid/g total phenolic compounds, fresh non-fat milk contained only 1·3 ng/ml and 0·04 μg gallic acid/g, respectively. These compounds are present in higher concentrations in the lipid fraction than in the aqueous fraction of cows’ milk; thus, the milk fat fraction of non-homogenised milk contained significantly higher amounts of phenolic compounds (2·42 v. 0·20 μg gallic acid/g) and equol (2·27 v. 0·13 ng/ml; P<0·05) than the corresponding aqueous fraction, respectively. Therefore, skimming may potentially reduce the levels of the original equol and phenolic compounds that otherwise may depend on the feeding practice of the cow.
Influence of animal species, fat content, thermal treatments and storage
The antioxidant capacity of milk differs according to the animal source: values of 1·0, 1·7, 1·7, 2·1 and 2·8 μmol TE/g (ABTS assay) were reported for human, cows’, horse, buffalo and goats’ milks, respectively( Reference Oner, Sanlidere-Aloglu and Dedebaş 56 ). The authors concluded that goats’ milk contains the highest concentrations of free radical scavengers than the others without giving further explanation. However, there is no doubt that the difference in antioxidant capacity according to animal origin is mainly due to the different composition of antioxidant compounds and/or the interaction of these compounds with the matrix to attenuate (by counteracting ion oxidation processes) or increase this potential. A higher antioxidant potential of goats’ milk compared with cows’ and donkey milk was also calculated by Simos et al.( Reference Simos, Metsios and Verginadis 57 ). These authors explained that the high antioxidant capacity of goats’ milk can be attributed to its richness in antioxidants, as well as the particular combination of compounds or their greater bioavailability.
According to the CUPRAC and ABTS assays, the highest total antioxidant capacity has been observed for fatty milks( Reference Cekic, Demir and Baskan 35 ). Skimmed milk has a 21 % (CUPRAC) and 6 % (ABTS) lower total antioxidant capacity than fatty milk. According to the authors, since fat has no antioxidant activity, total antioxidant activity of milk fat can be considered to be due to antioxidants entrapped in fat during milk homogenisation before UHT sterilisation.
Further, in the study by Zulueta et al. ( Reference Zulueta, Maurizi and Frigola 28 ), the fat content of the milk was not correlated with the total antioxidant capacity in UHT milk, while the total antioxidant capacity of low-fat pasteurised milk was significantly lower (13624–13984 μmol TE/l; ORAC assay) than that of milk with a higher fat content (14124–14216 μmol TE/l). Chen et al.( Reference Chen, Lindmark-Mansson and Gorton 30 ) also observed that the total antioxidant capacity (ABTS assay) in commercial cows’ milk (3 % fat) was significantly higher than in cows’ milk with 0·5–1·5 % fat and skimmed milk. Such results suggested that interference with lipids and the reactivity of the lipophilic antioxidants and the fat globule membrane proteins may influence the total antioxidant capacity.
In addition to animal species and skimming, thermal treatments may also influence the milk antioxidant potential. For example, changes in potential antioxidants and pro-oxidants in pasteurised and heated skimmed milk in an oil bath at 80, 90 and 120°C for up to 24 h were measured( Reference Calligaris, Manzocco and Anese 58 ). At each heating temperature, the milk samples showed an initial increase in the pro-oxidant activity until approximately 5 h of thermal treatment, followed by a decrease in this activity until 24 h; the decrease was associated with an increase in the milk antioxidant activity, especially at 90°C and 120°C. Such changes were explained by both the thermal degradation of natural antioxidants and the formation of novel oxidised molecules in the early stages of the Maillard reaction. Therefore, short heat treatments may be responsible for the depletion of the overall antioxidant properties of milk. However, severe heat treatments provide recovery and even a possible increase in the antioxidant properties of milk due to the formation of brown melanoidins (Maillard reaction products). These results emphasise the important role played by the time–temperature combination of heat treatments, which could explain the apparent discrepancy with other studies showing no significant effect of temperature on the antioxidant capacity of milk.
In the previously mentioned study by Şanlidere Aloğlu( Reference Şanlidere Aloğlu 42 ), the average values for raw, pasteurised (72–75°C for 15–30 s) and sterilised (121°C for 15 min) milks were 4·02, 4·47 and 4·18mmol TE/g (ABTS assay), respectively, with no significant difference between these values. In a study of Zulueta et al. ( Reference Zulueta, Maurizi and Frigola 28 ) the total antioxidant capacity of commercial UHT milks (ORAC assay) varied from 12·0 (sd0·5) to 14·5 (sd0·4) μmol TE/ml, and the capacity of pasteurised commercial milk ranged from 13·6 (sd0·7) to 14·2 (sd1·1) μmol TE/ml (ORAC assay), with no overall significant difference between the UHT and pasteurised milks. Similarly, heating the milk to 63°C for 1 h did not affect the total antioxidant capacity( Reference Chen, Lindmark-Mansson and Gorton 30 ). Therefore, Maillard reaction products resulting from heating appear to compensate for the heat-induced loss of organic antioxidant compounds.
Contrary to human breast milk, the influence of storage time on the antioxidant capacity of cows’ milk has rarely been studied. The most significant study measured the antioxidant capacity (FRAP assay) of six raw cows’ milk samples collected directly from a dairy and immediately cooled to 4°C for up to 48 h (i.e. refrigerated storage)( Reference Amamcharla and Metzger 59 ). The FRAP values of the raw milk significantly decreased with refrigerated storage time, i.e. –27 % upon 48 h of storage. According to the authors, this reduction in the total antioxidant capacity of milk can be attributed to constant and undefined interactions involving many pro- and antioxidants, but further details were not given.
Conclusions
Whole milk contains both hydrophilic (i.e. proteins and peptides, lactoferrin and antioxidant enzymes) and lipophilic antioxidants (i.e. lipophilic vitamins and CLA), and its overall total antioxidant activity will notably depend on the fat content and time–temperature combination applied (for example, Maillard products) (Table 2).
Table 2 Summary of the in vitro antioxidant capacity of the main dairy products
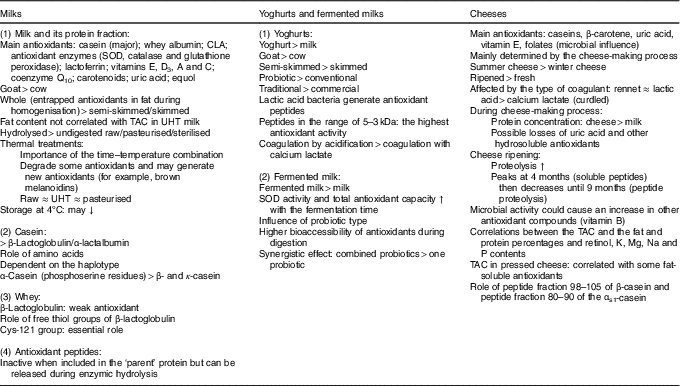
CLA, conjugated linoleic acid; SOD, superoxide dismutase; TAC, total antioxidant capacity; UHT, ultra-high temperature; ↓, decrease; ↑, increase.
Yoghurts and other fermented milks
Antioxidant potential
The antioxidant properties of naturally fermented milk result from the presence of casein, whey proteins, peptides, amino acids, uric acid, vitamins C, E, D and A, β-carotene, and enzymic systems (SOD, catalase, and glutathione peroxidase), as well as the presence of CLA and coenzyme Q10 and the antioxidant activity of the lactic acid bacteria or kefir culture( Reference Zulueta, Maurizi and Frigola 28 , Reference Jiménez, Antonia Murcia and Parras 60 – Reference Pihlanto 62 ). Fermented milks may therefore exhibit very different antioxidant potentials according to the origin of the milk and to the nature of the bacterial strain. Among different plain fermented products marketed in Poland (i.e. twelve yoghurts, twelve kefirs, two buttermilks, two cultured milks and a Turkish drink yoghurt called ayran), yoghurt and kefir were shown to have the highest antioxidant activity: the mean FRAP values were 1·19 (yoghurts), 1·03 (kefirs), 0·66 (cultured milks), 0·48 (ayran) and 0·45 (buttermilks) μmol Fe2+/g( Reference Najgebauer-Lejko and Sady 63 ). The ability to scavenge DPPH radicals was 0·19mmol TE/kg for the yoghurts and 0·17 μmol TE/g for the kefirs, followed by the buttermilks, cultured milks and ayran, for which the mean antiradical power was 0·14, 0·11 and 0·09 μmol TE/g (DPPH assay), respectively. The authors attributed this effect to the fortification of the yoghurt and kefir products with milk powder and/or milk proteins. In addition to a high protein content, the presence of additional probiotic strains of Lactobacillus casei also positively affected the values obtained from the FRAP and DPPH assays in this study.
Influence of the mammal species
The study by Balakrishnan & Agrawal( Reference Balakrishnan and Agrawal 64 ) included the preparation of a fermented milk (with Pediococcus pentosaceus from cheese) from various sources such as cows’, goats’ and camel milk. The scavenging activity of milk (DPPH assay, %) after 24 h of fermentation was the highest with goats’ milk (93 v. 54 % before fermentation), followed by camel milk (84 v. 64 % before fermentation) and cows’ milk (78 v. 39 % before fermentation), illustrating an important increase of up to +39 % upon fermentation. A higher antioxidant activity (Trolox equivalent antioxidant capacity-ABTS assay) was also observed with camel milk fermented with Lactobacillus rhamnosus PTCC 1637 compared with cows’ milk( Reference Moslehishad, Ehsani and Salami 65 ). This was attributed to a higher proline content in the primary structure of camel milk caseins.
Influence of casein polymorphism
Perna et al.( Reference Perna, Intaglietta and Simonetti 66 ) evaluated the antioxidant activity (FRAP and ABTS assays) of a yoghurt made from milk characterised by different casein haplotypes (αs1-, β- and κ-caseins) and fortified or not with chestnut and sulla honey. A haplotype is a group of alleles of different loci located on the same chromosome and usually transmitted together. The antioxidant activity of the control yoghurt was 204·43(sd57·81) μg ascorbic acid equivalents/ml based on the ABTS assay and 116·74(sd29·30) μg ascorbic acid equivalents/ml based on the FRAP assay. The high antioxidant capacity of the yoghurt was attributed to several parameters, including the presence of different bioactive peptides from the proteolysis of milk proteins by lactic acid bacteria, the fermentation and post-acidification during storage that produce organic acids, and the possible peptide aggregation that occurs during the enzymic hydrolysis of whey protein and casein. Haplotypes BB-A2A1-AA and BB-A2A1-AB had the highest values in both antioxidant assays compared with the control yoghurt, clearly showing that milk protein polymorphism influences the antioxidant activity of yoghurt.
Influence of fermentation and storage
When comparing a skimmed milk with the corresponding fermented milk, Jiménez et al.( Reference Jiménez, Antonia Murcia and Parras 60 ) observed that the fermented milk (46 %) was less effective than the skimmed milk (65 %) at inhibiting lipid peroxidation. However, both products trapped approximately 85 % of free hydroxyl radicals (•OH). Moreover, based on the ABTS assay (Trolox equivalent antioxidant capacity), fermented milk had a significantly lower total antioxidant capacity of approximately 48 % relative to the skimmed milk after 6 min of measurement but had a capacity of +62 % after 24 h of measurement. No explanation was proposed for this unexpected effect.
The proteolytic systems of lactic acid bacteria are the main way to generate bioactive peptides, including both free radical-scavenging antioxidant activity and angiotensin-converting enzyme-inhibiting activity, during fermentation of the milk( Reference Gobbetti, Minervini and Rizzello 67 ). Evaluating the antioxidant properties of a skimmed milk fermented with Lactobacillus delbrueckii ssp. bulgaricus LB340 revealed that among four peptide fractions of 10–5, 5–3, 3–1 and <1·0 kDa, those in the range of 5–3 kDa exhibited the highest antioxidant activity (66 %, DPPH assay), which was not significantly different from that of the 3–1 kDa peptide fraction (64 %)( Reference Qian, Xing and Cui 68 ). The DPPH free radical-scavenging effect of the <1·0 kDa peptide fraction reached 55 %. Furthermore, the antioxidant activity was correlated with the molecular weight from 0 to 5 kDa. However, considering the mucus layer in the small intestine, which has a thickness of 100–150 μm, molecular filters with an exclusion molecular weight of 0·6–0·8 kDa were used, and due to the peptidase in the intestinal epithelial cells, the authors suggested that ‘the antioxidant activity of the peptide fraction less than 1·0 kDa might represent that of fermentation better in vivo ( Reference Cao and Zhang 69 )’( Reference Qian, Xing and Cui 68 ). Qian et al.( Reference Qian, Xing and Cui 68 ) reported results that agreed with those of Peng et al.( Reference Peng, Xiong and Kong 70 ) showing that the 0·1–2·8 kDa fraction from whey protein isolation had the strongest free radical-scavenging capacity.
In a commercial fermented milk, researchers tried to identify the peptides included in the most active (angiotensin-converting enzyme and antioxidant activities) protein fractions isolated by reverse-phase HPLC-MS( Reference Hernández-Ledesma, Miralles and Amigo 71 ). Among several potentially active peptides, the β-casein peptide KVLPVPQ may possess antioxidant activity. Of the isolated fractions, the highest antioxidant activity was found in a fraction where seven of the eight peptides identified contained at least one proline residue, and six of them had more than two proline residues. Therefore, according to the authors, ‘the high content of proline peptides could determine the antioxidant activity found in this fraction’( Reference Hernández-Ledesma, Miralles and Amigo 71 ).
In a study by Aloğlu & Oner( Reference Aloğlu and Oner 72 ), the physico-chemical and microbiological properties of commercial and traditional yoghurts were determined during 4 weeks of storage, as well as the proteolytic activity and antioxidant activities (DPPH and ABTS assays) of peptides derived from hydrolysis. The antioxidant activity of water-soluble extracts of traditional yoghurt was higher than that of commercial yoghurt, reflecting differences in the chemical and microbiological properties. The physico-chemical and microbiological properties of traditional yoghurts generally do not meet the commercial standards. During storage for up to 4 weeks (4°C), the average values increased from 7·7 to 8·7 mmol TE/g for the commercial yoghurts and from 10·1 to 13·2mmol/g for the traditional yoghurt (ABTS assay). The presence and position of the amino acids tryptophan, tyrosine and methionine in the peptides were believed to be responsible for the antioxidant activity. Finally, if the initial total antioxidant activity of the yoghurts was low, the activity of the peptides released from the HPLC fractions was 10- to 200-fold higher.
Concerning the influence of cold storage (4°C), an increased antioxidant activity of fermented cows’ and camel milks was observed for up to 21 d due to an increase in proteolytic activity, but the increase primarily occurred between 14 and 21 d( Reference Moslehishad, Ehsani and Salami 65 ). Thus, the increase in proteolysis during storage increases the release of antioxidant peptides even more than fermentation.
Influence of the probiotic strain
The influence of the strain of probiotic on the antioxidant potential of fermented milks has been studied much more than the previous parameters. Most of these studies were based on the release of antioxidant peptides by different probiotics.
Studies have coherently demonstrated a correlation between an increased degree of proteolysis and the antioxidant potential. In the most recent study, Solieri et al.( Reference Solieri, Rutella and Tagliazucchi 73 ) isolated non-starter lactobacilli from different cheeses because of their proteolytic activity and their ability to produce fermented milk enriched with angiotensin-converting enzyme and antioxidant peptides. Thirty-four strains of Lactobacillus from Parmesan cheese and five strains from Pecorino Siciliano (or Canestrato) were screened for their ability to hydrolyse milk proteins, and two strains from Parmesan, Lactobacillus casei PRA205 and Lactobacillus rhamnosus PRA331, were selected to inoculate sterile cows’ milk. The milk fermented with Lactobacillus casei PRA205 had a higher radical-scavenging activity (1·2(sd0·0) mmol TE/ml) than the milk fermented with Lactobacillus rhamnosus (0·9(sd0·1) mmol TE/ml) (ABTS assay). In addition to this effect, the increases in proteolytic and radical-scavenging activities measured during the fermentation of milk for 120 h were clearly correlated, confirming the important role of peptides in the antioxidant activity of fermented milks. Ramesh et al.( Reference Ramesh, Kumar and Singh 74 ) studied the influence of different lactobacilli strains (n 19) from ten different species on the antioxidant capacity of the whey fraction of skimmed milk. The radical-trapping antioxidant activity ranged from 0·12 to 0·49 mmol TE/l (ABTS assay), confirming the low antioxidant capacity of the whey fraction. In agreement with previous results, the antioxidant and proteolytic activities of seven strains (i.e. Lactobacillus delbrueckii ssp. bulgaricus NCDC08, Lactobacillus plantarum NCDC25, Lactobacillus casei ssp. casei NCDC17, Lactobacillus rhamnosus NCDC24, Lactobacillus paracasei ssp. paracasei NCDC63, Lactobacillus fermentum NCDC141 and Lactobacillus helveticus NCDC288) were positively correlated. After the hydrolysis of casein with cell-free extracts from these seven strains, a significant increase in antioxidant activity was observed for Lactobacillus rhamnosus NCDC24, Lactobacillus casei ssp. casei NCDC17 and Lactobacillus paracasei ssp. paracasei NCDC63. For these three strains, low-molecular-weight peptides (<3·0 kDa) represented 60–70 % of the total antioxidant activity. Virtanen et al.( Reference Virtanen, Pihlanto and Akkanen 75 ) studied the production of antioxidant activity (ABTS assay and lipid peroxidation) during fermentation with commonly used lactic acid ferments. Sterilised skimmed milk (120°C for 20 min) was fermented with twenty-five different strains of lactic acid bacteria, and the six with the highest antioxidant activity were selected. The whey fraction was isolated from each fermented milk for measurement of its antioxidant capacity. In both assays, the strains Leuconostoc mesenteroides ssp. cremoris, Lactobacillus jensenii (American Type Culture Collection (ATCC) 25258) and Lactobacillus acidophilus (ATCC 4356) exhibited the highest antioxidant activity. The development of antioxidant activity was correlated with proteolysis for four strains. Additionally, the highest hydrophobic amino acid content and proportion of peptides in the molecular mass range of 4–20 kDa were found within fermentates with the highest antioxidant capacity, while the other fermentates mostly contained large polypeptides and compounds below 4 kDa. The development of antioxidant activity was therefore clearly specific for the strain. Interestingly, the authors concluded that ‘The development of radical scavengers was more connected to the simultaneous development of proteolysis whereas lipid peroxidation inhibitory activity was related to bacterial growth’( Reference Virtanen, Pihlanto and Akkanen 75 ). Similarly, Sah et al.( Reference Sah, Vasijevic and McKechnie 76 ) evaluated the effect of the probiotics Lactobacillus acidophilus (ATCC 4356), Lactobacillus casei (ATCC 393) and Lactobacillus paracasei ssp. paracasei (ATCC BAA52) on the antioxidant and anti-mutagenic activity of peptides extracted from yoghurt. Again, the degree of proteolysis by these probiotics was highly correlated with the antioxidant and anti-mutagenic activities of peptides, and the released peptides showed high antioxidant activity in the DPPH assay. The yoghurt containing the three probiotic strains together had the highest degree of hydrolysis and the highest antioxidant activity, emphasising a potential synergistic effect.
Other studies have generally compared the respective antioxidant potential of adding different probiotic strains and emphasised the synergistic action of combined strains compared with only one strain. A sterilised milk (unspecified thermal conditions) with 3·2 % fat was fermented with different microbial cultures, including a symbiotic culture of Lactobacillus delbrueckii ssp. bulgaricus and Streptococcus thermophilus and monocultures of Lactobacillus acidophilus, Lactobacillus casei and Bifidobacterium bifidus ( Reference Gjorgievski, Tomovska and Dimitrovska 77 ). For all cultures, the antioxidant activity of the fermented products was increased compared with non-fermented milk. The highest antioxidant activity was observed with Lactobacillus acidophilus, which neutralised 55 % of the free radicals, and the lowest activity was observed with Lactobacillus delbrueckii ssp. bulgaricus and Streptococcus thermophilus (45 %). The authors suggested that the increased antioxidant activity was not only due to released peptides but also to free amino acids, enzymes and various other compounds. Similarly, Lim( Reference Lim 78 ) evaluated the antioxidant properties of yoghurt fermented with lactic acid bacteria from pickled cabbage. Yoghurt fermented with Lactobacillus acidophilus PC16 had the highest antioxidant activity (DPPH assay) compared with yoghurt fermented with Lactobacillus casei PC05. Conversely, the ability to scavenge the superoxide anion and the activity of the enzyme SOD were significantly higher in yoghurt fermented with Lactobacillus casei PC05 compared with yoghurt fermented with Lactobacillus acidophilus PC16. Upon cold storage, there was no significant difference in the antioxidant activities of these yoghurts. In the study by Aleksandrova et al. ( Reference Aleksandrova, Chikov and Velikova 79 ) four strains of Lactobacillus delbrueckii were selected based on their high proteolytic activity. All of the strains belonged to the subspecies lactis and bulgaricus and were used in combination with a commercial strain for yoghurt LBB BY D4 (LB Bulgaricum plc) to produce a yoghurt-type dairy product (from skimmed milk). The in vivo antioxidant activity caused by the peptides obtained during the fermentation of milk proteins was analysed in living yeast cells. The main results showed that the antioxidant capacity towards reactive oxygen species in the yeast cells was higher with the combination of strains than with only the commercial strain and that the different strains exhibited different antioxidant capacities. Osuntoki & Korie( Reference Osuntoki and Korie 80 ) isolated lactobacilli from five native Nigerian fermented foods to ferment whey fractions from skimmed milk and measure their antioxidant activity (DPPH assay and lipid peroxidation inhibition assay). All the whey fractions had antioxidant activity, and the five isolates of lactobacilli with the highest activity were selected (Lactobacillus brevis, Lactobacillus fermentum, Lactobacillus plantarum, Lactobacillus casei and Lactobacillus delbrueckii). The whey fractions were then fermented for 24 h with the selected micro-organisms. The antioxidant activity increased during the 24 h in all cases, but differently according to the strain; however, with Lactobacillus delbrueckii, the activity increased less and plateaued at 4 h. The authors further concluded that the fermented milk may serve as a vehicle for probiotic lactobacilli from non-dairy sources. Finally, Parrella et al.( Reference Parrella, Caterino and Cangiano 81 ) studied the antioxidant capacity (ORAC and FRAP assays) of nine different samples of fresh skimmed (derived directly from the udder of the cow), pasteurised and UHT milks before and after fermentation with several lactobacilli, individually, and in combination with the yeast Saccharomyces boulardii. As expected, all fermented milk samples had greater antioxidant activity than the unfermented milk, with the effect being significant only for fresh milk. The co-incubation of the lactobacilli with yeast led to greater antioxidant activity, especially for fresh milk.
Specific case of kefir
Kefir is a fermented milk drink prepared by inoculating cows’, goats’ or sheep’s milk with kefir grains. The kefir grains initiating the fermentation are a combination of lactic acid bacteria and yeasts in a matrix of proteins, lipids and sugars. This symbiotic culture forms ‘grains’ that resemble cauliflower. Lactobacillus species are always present in kefir grains. Kefir appeared to have higher antioxidant potential but reduced glutathione peroxidase activity compared with the milk from which it has been fermented( Reference Liu, Chen and Lin 82 ). In addition, the free radical-scavenging activity of the plain kefir increased by 10 % with prolonged fermentation from 16 to over 24 h( Reference Liu, Chen and Lin 82 ). However, no difference was observed in the ferrous ion-chelating ability or SOD activity. Liu et al.( Reference Liu, Lin and Chen 61 ) explained that ‘The antioxidative activity of kefirs may be attributed to their proton-donating ability, their reducing power and SOD-like activity as evidenced through DPPH and superoxide radical scavenging and lipid peroxidative inhibition results’. Concerning the different types of kefir, Kesenkas et al.( Reference Kesenkaş, Dinkçi and Seçkin 83 ) compared full-fat and skimmed milk-based kefir inoculated with grains with a commercial culture using four different antioxidant assays and found that the fat content and the nature of the fermentation did not affect the antioxidant properties of the kefir. These results suggest that kefirs may be relevant candidates for useful and natural antioxidant supplements in the human diet.
Influence of in vitro digestion
Gagnon et al.( Reference Gagnon, Savard and Rivière 84 ) evaluated the bioaccessibility of antioxidants during the in vitro digestion of a reconstituted skimmed milk that had been fermented with five selected strains of Bifidobacterium longum ssp. longum. Contrary to previously mentioned studies, the milk fermented with these five strains did not have an initial antioxidant capacity (ORAC assay) higher than unfermented milk. However, higher bioaccessibility of the antioxidants in the fermented milk was observed during digestion. Surprisingly, strains with a low survival rate – compared with the strain showing the best survival rate – in fermented milk were shown to deliver more bioaccessible antioxidants during in vitro dynamic digestion. Therefore, the milk fermented with various strains of Bifidobacterium longum ssp. longum provided bioaccessible antioxidants during digestion, notably at the colonic level, that could prevent the excessive generation of oxidative compounds (i.e. free radicals by microbiota) within the digestive tract( Reference Azcarate-Peril, Sikes and Bruno-Barcena 85 ).
Conclusions
Yoghurt exhibits higher antioxidant potential than milk, notably due to the presence of probiotics generating antioxidant peptides. This is also true for other fermented milks but antioxidant activity greatly depends on the type and number of added probiotics (Table 2).
Cheeses
Influence of cheese processing and composition
The most recent study dealt with the factors affecting the antioxidant capacity (ABTS assay) of 224 different cheese samples (of known composition) prepared using sixteen varied mixtures of milk from cows, ewes and goats during two manufacturing seasons (winter and summer) and over 6 months of ripening( Reference Revilla, Gonzalez-Martin and Vivar-Quintana 86 ). Several correlation analyses were performed. First, the total antioxidant capacity was significantly correlated with the seasonality of manufacturing and the time of ripening but not with the animal species providing the milk. The antioxidant capacity was higher in summer than in winter because of the higher Trolox equivalent antioxidant capacity values observed at month 0 (except for winter goats’ cheese) and mainly at 1 and 2 months in the summer cheeses. This difference resulted from the lower hydrophobic:hydrophilic peptide ratios in summer than in winter cheeses, especially after the first month of ripening, indicating strong proteolysis. Second, significant correlations between the total antioxidant capacity and the retinol content (r 0·399), fat percentage (r 0·308), protein percentage (r 0·366), K content (r 0·385), Mg content (r 0·312), Na content (r 0·432) and P content (r 0·272) were reported, in agreement with previous results( Reference Lucas, Andueza and Rock 87 ). However, the positive correlations between minerals and the total antioxidant capacity are probably due to the significant positive correlation between the protein content (because the total antioxidant capacity is primarily associated with caseins) and these minerals. Based on these results, the authors generated a model to predict the antioxidant capacity in unknown cheeses of different compositions and ripening times( Reference Revilla, Gonzalez-Martin and Vivar-Quintana 86 ).
Therefore, because cheeses exhibit a very high diversity of composition and structure (matrix characteristics), their antioxidant potential could be modulated. Lucas et al.( Reference Lucas, Rock and Chamba 88 ) studied the respective effects of the composition of milk and processing on the antioxidant potential of five varieties of French cheese, i.e. Abondance (n 10 cheeses, including both winter and summer cheeses), Tomme de Savoie (n 10, including both winter and summer cheeses), Cantalet (n 12, only winter cheeses) and Salers (n 6, only summer cheeses) from cows’ milk, and Rocamadour (n 15, including both winter and summer cheeses) from goats’ milk. The compositional variability of the cheese in terms of fatty acids, β-carotene, xanthophylls and vitamin E depended mainly on the animal species composition of the milk, i.e. goat v. cow. The total antioxidant capacity of the cheeses ranged from 6·35 to 24·3 mmol Fe2+/g DM (FRAP assay). As expected, a higher total antioxidant capacity was measured in goats’ milk. In this study, statistical analyses showed no significant correlation between the milk composition and total antioxidant capacity. Furthermore, none of the antioxidant compounds analysed in this study was found to be positively or negatively correlated with the total antioxidant capacity, which can be explained either by the synergistic action of several compounds or by the action of antioxidant compounds not analysed in this study.
Pattorn & Hongsprabhas( Reference Pattorn and Hongsprabhas 89 ) studied the influence of coagulation methods – commonly used to prepare milk protein curds from skimmed milk – on the antioxidant activity of milk protein curds and powders. Precipitation by calcium lactate (curd), coagulation by lactic acid and rennetting of skimmed milk proteins were tested. Curdled milk from precipitation had the densest network (as observed via scanning electron microscopy) and the lowest antioxidant capacity, i.e. 0·27 (ORAC assay) and 0·05 (ABTS assay) μmol TE/mg protein, compared with the other dairy preparations (0·52–0·57 and 0·42–0·44 μmol TE/mg protein, respectively; P<0·05). Furthermore, trypsin hydrolysis for 30 min increased the antioxidant capacity of all products to 0·85–1·11 (ORAC assay) and 1·17–1·19 (ABTS assay) μmol TE/mg protein, regardless of the coagulant used. In terms of practical applications, these results showed that the milk powder precipitated by calcium lactate could be used as a dietary protein source that would provide antioxidant peptides during tryptic digestion. As written by the authors, the ‘study also suggests that the hydrolysates of milk proteins with reasonable antioxidant capacity could be achieved with milk powder prepared from mixed protein coagulated by calcium lactate, lactic acid or rennet, and then hydrolyzed by trypsin’, adding that ‘this is important for developing countries with majority of the population suffering from lactose intolerance, i.e., having milk surplus, yet lacking the capacity of fractionation of milk proteins into individual proteins such as casein β, casein κ, β-lactoglobulin and α-lactalbumin’( Reference Pattorn and Hongsprabhas 89 ).
Gupta et al.( Reference Gupta, Mann and Kumar 90 ) aimed to isolate and characterise antioxidant peptides (<3 kDa, isolated by reverse-phase HPLC and analysed by liquid chromatography-tandem MS, ABTS assay) in Cheddar-type cheese inoculated with Lactobacillus casei ssp. casei 300. The authors identified two peptides from milk proteins: VKEAMAPK (m/z 872·5048), corresponding to the 98–105 fraction of β-casein, and HIQKEDVPSER (m/z 1336·7034), corresponding to the 80–90 fraction of αs1-casein (i.e. protein fragment composed of amino acids initially at positions 98–105 and 80–90 of the linear protein sequence). Interestingly, the antioxidant activity of the VKEAMAPK peptide was comparable with that of commercial antioxidants such as butylated hydroxyanisole, tert-butylhydroquinone and ferulic acid, but the activity of the HIQKEDVPSER peptide was lower. Therefore, this proteolytic strain of lactic acid bacteria can be used to further improve the antioxidant potential of Cheddar cheese via the release of antioxidant peptides.
Influence of ripening time
A recent study investigated the proteolysis and total antioxidant capacity of proteins from white brined cheese prepared from overheated goats’ milk and ripened for 50 d( Reference Barac, Pesic and Zilic 91 ). Proteolysis after cheese ripening induced increases in the antioxidant capacity of both the water-soluble and water-insoluble protein fractions. For example, after 30 d of ripening, the total antioxidant capacity of the water-soluble fraction increased by 12 %. After that period, a more intensive increase was observed, and cheese ripened for 50 d had a total antioxidant capacity that was 56 % higher than that of fresh cheese. This study showed that both the content and nature of N components are essential for the antioxidant capacity. Similarly, Parvin et al. ( Reference Parvin, Rahman and Shimazaki 92 ) showed that the antioxidant activity of Bangladesh Gouda and Dhaka cheeses increases with ripening time (up to 2 months).
Gupta et al.( Reference Gupta, Mann and Kumar 93 ) evaluated changes in the antioxidant properties (ABTS and DPPH assays, scavenging activity and superoxide anion) of aqueous extracts of Cheddar cheese (inoculated with Lactobacillus casei ssp. casei 300 and Lactobacillus paracasei subsp. paracasei 22) at different stages of ripening but on a longer time scale than in previous studies. As previously reported, the antioxidant activity first increased to a peak at 4 months and then decreased until 9 months of ripening. The changes in the antioxidant activity were linked to the rate of formation of soluble antioxidant peptides upon proteolysis until 4 months. The decrease observed after 4–5 months of ripening indicates that the antioxidant peptides were not resistant to the continuing proteolysis.
The antioxidant capacity (ABTS assay) of water-soluble extracts of Parmigiano Reggiano cheese at different ageing times (up to 41 months) was also studied (ABTS assay)( Reference Bottesini, Paolella and Lambertini 94 ). The antioxidant capacity was determined after simulated in vitro gastrointestinal digestion of these extracts. Contrary to previous studies, the antioxidant capacity in water extracts of Parmigiano Reggiano cheese was unaffected by the ripening time and gastrointestinal digestion. Using liquid chromatography-electrospray ionisation-MS, the authors also showed that the antioxidant capacity of the water-soluble extracts was mostly due to free amino acids, mainly tyrosine, methionine and tryptophan, and secondarily to antioxidant peptides, but only minimally.
It is difficult to make generalisations from these studies but it seems that the antioxidant capacity of cheese increases upon ripening to an optimum due to peptide release then decreases due to peptide hydrolysis; this effect and the optimum antioxidant capacity probably depend on cheese type and ripening time.
Influence of in vitro digestion
In the study by Abadia-Garcia et al. ( Reference Abadia-Garcia, Cardador and del Campo 95 ), the viability of probiotic micro-organisms added to cottage cheese in simulated gastrointestinal digestion, the release of peptides with potential antioxidant activity (DPPH and ABTS assays) and their antimicrobial effect on Listeria monocytogenes were evaluated. Cottage cheese was prepared with Lactobacillus casei, Lactobacillus rhamnosus GG, and the commercial mix YO-MIX™ 205 incorporated, in addition to a control without added probiotics. Higher numbers of potentially bioactive peptides were observed in the cheeses with added probiotics. During storage for 28 d (8°C), the antioxidant activity of the soluble cheese extracts determined by the DPPH assay increased, but the one determined by the ABTS assay did not (no difference between the control and the cheeses containing probiotics). Based on the DPPH assay, the increase was strongest with the commercial mix and lower with the control and probiotic Lactobacillus rhamnosus GG. This increase was attributed to the proteolytic activity of the micro-organisms that released antioxidant bioactive peptides. The different behaviour depending on the antioxidant assay used may be due to the different structure of the radical, which can react differently depending on the peptides present in the aqueous extract. According to the authors, these results indicated that the ‘probiotic microorganisms exhibited a significant level of survival in simulated gastric juice when delivered in a cottage cheese’( Reference Abadia-Garcia, Cardador and del Campo 95 ). In contrast, ‘environmental simulated conditions of the duodenum, where bile salts and pancreatic juices are secreted, had more influence on bacterial viability’( Reference Abadia-Garcia, Cardador and del Campo 95 ).
Conclusions
As with yoghurt and fermented milks, cheese also contains antioxidants, especially casein, but with less variety resulting from losses during the cheese-making process. Its total antioxidant activity is mainly determined by the process used, notably that which concentrates the protein fraction leading to higher antioxidant potential than milk. During ripening, proteolysis combined with possible microbial activity may increase this potential (Table 2).
Animal studies
Milk and milk proteins
The influence of milk and its derived proteins on several physiological functions has been studied in either rats or mice. However, the designs and objectives of each study have greatly differed.
In the most recent study, male control and anaemic weaned rats were administered for 30 and 50 d cows’ or goats’ skimmed milk-based diets with either a normal (45 mg/kg) or overloaded (450 mg/kg) Fe content to induce chronic Fe overload( Reference Díaz-Castro, Pérez-Sánchez and Ramírez López-Frías 96 ). Fe overload is notably known for potentially increasing oxidative stress. Anaemia by Fe deficiency had no effect on the antioxidant enzymes (catalase and glutathione peroxidase) or lipid peroxidation in the liver, brain and erythrocytes. During chronic Fe repletion, the activity of SOD was largely higher in the group fed cows’ milk compared with the group consuming goats’ milk. Otherwise, the catalase and glutathione peroxidase activities were only slightly changed in rats fed a cows’ milk-based diet, suggesting that the level of both enzymes are sufficient to neutralise the expected high production of free radicals. In addition, a cows’ milk-based diet led to higher levels of lipid peroxidation (thiobarbituric acid-reactive substances; TBARS) compared with a goats’ milk-based diet, which was directly correlated with the increase in the SOD activity. The increase in lipid peroxidation reflects the neutralisation of the hydroxyl radical (•OH), a very reactive free radical, derived from dismutation of the superoxide anion (O2 •–). Finally, because goats’ milk had a positive effect on antioxidant defence, even in conditions of Fe overload, and limited lipid peroxidation, the authors proposed that goats’ milk should be recommended for those affected by nutritional Fe-deficiency anaemia, especially for individuals consuming oral Fe supplements. Another study was also based on the fact that Fe supplementation may induce lipid peroxidation and then contribute to the induction of intestinal lesions( Reference Zunquin, Rouleau and Bouhallab 97 ). This mechanism is notably observed in intense sports, where athletes frequently use a higher complementation of Fe with unknown effects. Since milk proteins have in vitro antioxidant properties, Zunquin et al.( Reference Zunquin, Rouleau and Bouhallab 97 ) believed that they could thwart potential secondary effects of Fe on oxidative stress in rats. After 30 d, compared with untrained rats, all exercise-trained rats exhibited an increase in antioxidant enzyme activities (SOD and glutathione peroxidase) and in Fe concentrations in their colonic mucosa and liver. All these parameters were significantly reduced by supplementation with casein milk protein. In addition to these effects, free casein and casein bound to Fe were found to significantly reduce Fe-induced lipid peroxidation (TBARS formation). Therefore, regarding anaemia and the consumption of oral Fe supplements, dietary milk protein supplementation could partly prevent the occurrence of deleterious effects to tissue induced by Fe overload.
Two other studies evaluated the influence of dairy products on the antioxidant status of obese rats. Indeed, angiotensin-converting enzyme inhibitors in dairy products may suppress the oxidative and inflammatory stress associated with obesity. To test this hypothesis, Zemel & Sun( Reference Zemel and Sun 98 ) carried out a study for 3 weeks in adipocyte fatty acid-binding protein-agouti transgenic mice fed obesogenic diets, including a diet rich in Ca and a diet rich in dairy products (skimmed milk powder: 400 g/kg). Compared with the basal diet, the production of reactive oxygen species in adipose tissue and the levels of NADPH oxidase mRNA and plasma malondialdehydes were reduced with the Ca-rich diet (P<0·05). The effect was even more pronounced with the dairy-rich diet (P<0·001). These results led the authors to conclude that dietary Ca and dairy products inhibit oxidative stress in a mouse model of diet-induced obesity and oxidative stress. They proposed that dietary Ca-induced suppression of circulating 1α,25-dihydroxycholecalciferol may be responsible for the Ca-induced suppression of oxidative stress. This hypothesis is based on the ability of 1α,25-dihydroxycholecalciferol to stimulate inflammatory cytokine production, as shown in an adipocyte and macrophage co-culture( Reference Sun and Zemel 99 ). Concerning milk proteins, casein and, more particularly, whey peptides were shown to reduce plasma, brain, heart and liver lipoperoxide contents and to increase liver glutathione and ATP contents in rats fed an obesogenic diet for 4 weeks and then casein- or hydrolysed milk-serum-restricted diets( Reference Broccali, Berti and Pistolesi 100 ). The authors proposed potential mechanisms for the higher efficacy of a whey peptide-based diet compared with casein in terms of an improved antioxidant status: (1) the smaller whey peptides are easily digested and absorbed, better promoting protein turnover for the synthesis of antioxidant enzymes; and (2) whey peptides supply β-alanine, which is fundamental for the synthesis of carnosine, an efficient antioxidant, as well as taurine, another antioxidant.
Considering the protective role of dietary Ca and other dairy components against oxidative and inflammatory stress, which otherwise accelerate the process of ageing, Bruckbauer & Zemel( Reference Bruckbauer and Zemel 101 ) evaluated the life-long effects of dietary Ca from both non-dairy and dairy (milk, non-fat, dry) sources on the lifespan of aP2-agouti transgenic (model of diet-induced obesity) and wild-type mice and on lifespan-related biomarkers, including some antioxidant biomarkers, until death. The age-related increase in reactive oxygen species production was significantly blunted in the high-Ca and milk-diet groups compared with the control group, with the effect being significant for the milk diet only after 78 weeks. Concerning SOD3 gene expression in the soleus, the wild-type mice showed increased levels in the milk-diet group, while no diet effect was found in the transgenic mice. Concerning SOD3 gene expression and enzyme activity in the liver, an overall increase in enzyme activity in the liver of mice in the milk-diet group compared with those in the high-Ca and control groups was observed, with no significant difference in gene expression. Therefore, although these effects had no influence on the maximum lifespan, they suppressed early mortality( Reference Bruckbauer and Zemel 101 ).
Finally, because diets containing evaporated milk or skimmed milk powder are particularly efficient at inducing Cu deficiency( Reference Jenkinson, Lawrence and Burk 102 , Reference Balevska, Russanov and Kassabova 103 ), the study by Lynch & Strain( Reference Lynch and Strain 104 ) investigated the effects of different diets based on skimmed milk powder, casein or whey on the Cu status of control and Cu-deficient rats. The tissue Cu levels, Cu-Zn SOD activity and cytochrome c oxidase activity were significantly lower in control animals fed skimmed milk powder compared with those fed casein, while lactoserum-fed rats showed intermediate values for these measurements linked to Cu status. In addition, the hepatic activities of glutathione reductase and Se-dependent glutathione peroxidase were significantly higher in rats fed the skimmed milk powder diet. However, all of the diets had significant effects on the hepatic levels of TBARS.
Fermented milks
In vitro fermented milks globally exhibit higher antioxidant potential than unfermented milks due to the release of antioxidant peptides. Only three studies have investigated this potential effect in either ageing mice( Reference Rohit, Rajeev and Meena 105 ), weanling rats( Reference Bay, Lee and Tan 106 ) or healthy normal rats( Reference Zommara, Tachibana and Sakono 107 ).
According to Rohit et al. ( Reference Rohit, Rajeev and Meena 105 ) the role of probiotics in the modulation of redox homeostasis during ageing remains unclear. Their study was conducted in male mice aged 16 months consuming a basic diet, supplemented or not with skimmed milk or milk fermented with probiotic Lactobacillus fermentum MTCC 5898. After 2 months on these diets, the antioxidant enzymes (SOD, catalase and glutathione peroxidase) in the probiotic group had significantly increased activities in the liver compared with those in the unfermented milk group. In erythrocytes, significantly increased activity was only observed for glutathione peroxidase. No significant difference between the control and unfermented skimmed milk groups was observed. Overall, these results clearly indicated an improved free radical clearance system following Lactobacillus fermentum MTCC 5898 administration.
In the following study, the effects of skimmed milk and cultured milk supplementation on peroxidative stress in the brains of weanling rats were investigated( Reference Bay, Lee and Tan 106 ). First, compared with the control brains, the TBARS concentrations were reduced in milk-supplemented animals. In addition, the brain SOD levels were significantly lower in the milk-supplemented animals, and the TBARS concentrations were positively correlated with SOD activity in the control animal brains. Otherwise, no significant differences in the brain glutathione S-transferase levels between the control, milk and cultured milk groups were reported. Therefore, both skimmed milk and cultured milk have antiperoxidative actions in vivo. In conclusion, the authors proposed that the milk protein rather than the lactobacilli was responsible for reducing lipid peroxidative stress in rat brains.
This next study examining the effect of whey from skimmed cows’ milk fermented with bifidobacteria and lactic acid bacteria on the antioxidant enzymes in rats for 6 weeks essentially confirmed previous results( Reference Zommara, Tachibana and Sakono 107 ). The rats consumed a reference control diet, a diet with whey from unfermented milk and a diet with whey from skimmed milk inoculated with Bifidobacterium longum, Lactobacillus acidophilus or Streptococcus salivarius ssp. thermophilus. The superoxide oxidase activity of blood cells and the liver catalase activity were increased by the whey from fermented milk, regardless of the nature of the strains, compared with the control diet. In addition, the activity of glutathione peroxidase in blood cells was higher with whey fermented with Lactobacillus acidophilus compared with the control diet. However, although no significant differences were observed between any of the diets in terms of plasma levels of TBARS and α-tocopherol, the lipoprotein fractions with a density less than 1·063 g/ml and prepared from rats fed the whey fermented with Bifidobacterium longum and Lactobacillus acidophilus were resistant to oxidative stress compared with the control diet. Overall, although some of the peroxidative changes induced by the cultured products were also observed with the non-fermented whey, the latter was less effective at increasing the activity of antioxidant enzymes than the cultured whey-based diets.
Cheeses
The effect of cheeses on the antioxidant status has been investigated only in rats. In the first study, thirty different Italian cheeses from three groups, i.e. ‘furmai da mut’, ‘caprino’ and ‘stracchino’ (n 10/group, different producer for all thirty cheeses), were added to the diets of thirty groups of four normal rats( Reference Cornelli, Bondiolotti and Battelli 108 ). Some of the cheeses from each group either significantly improved or deteriorated the oxidative balance, as measured through an oxidative index (plasma hydroperoxides/total cholesterol plasma level) and oxidative balance risk index ((oxidative index x total cholesterol plasma level)/plasma antioxidant ratio), but there was no significant effect when considering the pooled effect of the ten cheeses within each group.
The other study aimed at evaluating the effects of unripened cheese supplements on the antioxidant status in hypercholesterolaemic rats fed a high-cholesterol diet with or without 5 % unripened cheese for 6 weeks( Reference Bo-Young, Spengler and Roempp 109 ). The unripened cheese-based diet led to increases in plasma retinol and α-tocopherol of 40·5 and 39·2 %, respectively, and to an increased resistance to oxidative stress of leucocyte DNA of 52·3 % compared with the hypercholesterolaemic group without cheese. Furthermore, the significant increase in the peroxidation of plasma lipids in the hypercholesterolaemic group was reversed to its basal level in the presence of unripened cheese. The authors concluded that unripened cheese supplementation could exert significant health benefits in hypercholesterolaemic subjects, including improved antioxidant effects.
Conclusions
Animal studies investigating the antioxidant potential of dairy products are quite rare, and all have been carried out in different murine models. Goats’ milk, casein milk proteins, a dairy-rich diet, whey peptides, a milk-based diet and skimmed milk powder were all shown to reduce various markers of oxidative stress in different models of increased oxidative stress.
Concerning fermented milks, they all exhibited antioxidant activities in murine models, probably with a higher potential than unfermented milk, but this has to be confirmed. Finally, for cheese, results are inconclusive and paradoxical.
Human studies
In 2013, Cloetens et al.( Reference Cloetens, Panee and Akesson 4 ) reported that in adults, many intervention studies have been conducted on the effect of dairy products in the diet but very few have focused on the effect of milk on antioxidant systems. Indeed, only twelve relevant studies were identified, and among them one epidemiological study hypothesising the pro-oxidative effect of galactose.
Dairy products
Two studies by Zemel and collaborators( Reference Zemel, Sun and Sobhani 110 , Reference Stancliffe, Thorpe and Zemel 111 ) investigated the effect of dairy products or a dairy-based diet on the antioxidant status in overweight and obese subjects. The basis of their first study was that oxidative and inflammatory stress are elevated in obesity and are further augmented in the metabolic syndrome( Reference Stancliffe, Thorpe and Zemel 111 ). Therefore, forty overweight and obese adults with the metabolic syndrome were administered for 12 weeks a low-dairy (<0·5 dairy servings/d and <600 mg Ca/d) or adequate-dairy (>3·5 dairy product servings/d, ≥1200 mg Ca/d) weight-maintenance diet. The low-dairy diet had no effect on oxidative markers, i.e. plasma malondialdehyde (nmol/l) and oxidised LDL (ng/ml). Conversely, the adequate-dairy diet decreased malondialdehyde and oxidised LDL on day 7 (35 and 11 %, respectively; P<0·01), with further decreases by the twelfth week. Otherwise, the adequate-dairy diet exerted significantly greater effects on oxidative biomarkers in the obese subjects compared with the overweight subjects. These results showed that adequate intake of dairy products significantly attenuates oxidative as well as inflammatory stress in the metabolic syndrome, partly explaining the protective effect of dairy products against this metabolic dysregulation( Reference Abedini, Falahi and Roosta 112 – Reference Dugan, Barona and Fernandez 114 ).
Zemel et al.( Reference Zemel, Sun and Sobhani 110 ), 1 year before, compared dairy products with soya in the form of smoothies on oxidative and inflammatory stress in overweight and obese subjects. Smoothies were administered three times per d throughout a 28 d treatment period according to a blinded, randomised, cross-over design comparing dairy product- with soya-supplemented isoenergetic diets. The dairy product-based diet led to a significant reduction of oxidative stress, with a reduction of 22 % plasma malondialdehyde and 12 % plasma 8-isoprostane-F2α (P<0·0005). The soya-based diet had no effect. Higher effects were reported at 28 d than at 7 d. However, contrary to their above-mentioned study, there were no significant differences between the overweight and obese subjects.
Milk and milk-derived proteins
Studies concerning milk and its derived protein fraction also greatly differ in their designs and objectives.
The most recent study was an epidemiological cohort study carried out in Swedish women and men( Reference Michaëlsson, Wolk and Melhus 115 ). Compared with a low milk intake (<1 glass/d) and a high intake of fruit/vegetables (≥5 servings/d), the risk (hazard ratio, adjusted) for all-cause mortality was 2·79 (95 % CI 2·42, 3·21) in women consuming at least three glasses of milk/d and less than one serving/d of fruit/vegetables and 1·60 (95 % CI 1·40, 1·82) in women consuming the same amount of milk but at least five servings/d of fruit/vegetables. In men, the same comparisons resulted in risks of 1·31 (95 % CI 1·14, 1·51) and 1·07 (95 % CI 0·97, 1·18), respectively. Based on studies carried out on murine models, the authors hypothesised that the milk pro-oxidant galactose (component of lactose) may be one of the main causes of these observed effects( Reference Cui, Zuo and Zhang 116 – Reference Hao, Huang and Gao 118 ), with the increase in oxidative stress specifically associated with a lower life expectancy in animals( Reference Cui, Wang and Zuo 119 ) and with chronic diseases in humans( Reference Utkualp and Ozdemir 120 , Reference Moylan and Reid 121 ). Therefore, this study did not support a protective antioxidant effect of milk, as previously shown by the same researchers reporting higher concentrations of oxidative stress in human urine (levels of 8-iso-PGF2α) associated with a high consumption of milk( Reference Michaelsson, Wolk and Langenskiold 122 ). However, milk is a complex matrix containing not only lactose but also numerous antioxidant constituents that rebalance the overall antioxidant potential (for example, casein, lactoferrin, bioactive peptides released during digestion, and antioxidant enzymes). The above-mentioned studies in animals attest to the antioxidant protective effect of milk and dairy products in general. Excess simple sugars promote an increase in oxidative stress( Reference Prasad and Dhar 123 ). Therefore, one can regret that Michaelsson et al.( Reference Michaelsson, Wolk and Langenskiold 122 ) did not adjust the hazard ratio on total or added simple sugars, which often reflect an adherence to a Western (energy-rich and low level of fruits and vegetables) diet also rich in pro-oxidants. This may also means that heavy milk consumers more generally adhere to a Western pro-oxidant diet. Therefore, the comparison of ‘milk (<1 glass/d)+fruits/vegetables (≥5 servings/d)’ with ‘milk (≥3 glasses/d)+fruits/vegetables (<1 serving/d)’ is not the most common scenario, and the hazard ratio was no more significant when comparing, for example, ‘milk (1–1·9 glasses/d)+fruits/vegetables (2–4·9 servings/d)’ with ‘milk (<1 glass/d)+fruits/vegetables (≥5 servings/d)’. In the same study, beyond milk the increased consumption of fermented milk or yoghurts per d was shown to be associated with an improved antioxidant status in humans. More generally, the study by Michaëlsson et al.( Reference Michaelsson, Wolk and Langenskiold 122 ) showed the importance of a global approach to studying milk consumption in relation to the type of complex diet in which it is consumed. The pro-oxidative effect is probably attributed to the complex diet rather than to a single food product within the diet, for example, in a Western energy-rich diet.
Based on the fact that cows’ milk proteins may be a novel source of antioxidants that could be used in a nutritional strategy to maintain muscle mass during ageing, Power-Grant et al.( Reference Power-Grant, McCormack and De Cap 124 ) studied the antioxidant potential of different milk-based protein matrices (i.e. intact milk protein concentrate and two hydrolysate whey protein concentrates with 32 and 45 % degrees of hydrolysis) before and after simulated gastrointestinal digestion and determined whether the plasma antioxidant capacity was similarly modified in vivo following acute ingestion (after 3 h) of these matrices in healthy 50- to 70-year-old women. The in vitro digestion increased the antioxidant capacity by approximately 35 % for all matrices (from 24365 to 42592 μmol TE/100 g dry weight, P<0·05; ORAC assay). In vivo milk-based proteins increased the fasting plasma antioxidant capacity by approximately 23 % for all matrices (from 10952 to 13519 μmol TE/100 g dry weight, P<0·05; ORAC assay). Thus, there was an association between the changes in the ORAC-based measurement of the antioxidant capacities both in vitro and in vivo.
In the study by Hunter et al. ( Reference Hunter, Brown and Green 125 ) forty-two healthy smokers consumed lemonade or control milk twice daily for 2 or 6 weeks, respectively. Based on several antioxidant biomarkers (serum uric acid and plasma ORAC, FRAP, protein carbonyls and vitamin C), milk appeared neutral with no significant differences compared with lemonade.
Zavorsky et al.( Reference Zavorsky, Kubow and Grey 126 ) studied the consumption by healthy subjects of whey protein-based bars that had undergone high-pressure treatment. High-pressure treatment of whey protein may increase the digestibility and bioavailability of cysteine. Each group (n 3) ingested 15, 30 or 45 g/d of pressurised whey protein in the morning in bar format for 14 d. The significantly increased level of glutathione, a major cellular antioxidant in humans, in cells was linearly related with the amount of consumed whey protein. Thus, pressurised whey protein supplementation of 45 g/d for 2 weeks increased lymphocyte glutathione by 24 %.
Campbell et al.( Reference Campbell, Brown and Dufner 127 ) also studied the effect of milk proteins in healthy adult men (double-blind cross-over meal-challenge study). Based on the anti-atherogenic potential of soya isoflavones, their main objective was to test whether soya (85 mg of aglycone isoflavones, treatment) or 40 g of milk protein (0 mg of aglycone isoflavones, control) in combination with a high-fat meal can modify postprandial, atherogenic-associated events and biomarkers of oxidative stress (i.e. ex vivo Cu-induced LDL oxidation), inflammation and thrombosis. There was no significant difference between the treatments, suggesting an equivalent effect of soya isoflavones and milk proteins on LDL oxidation.
Önning et al.( Reference Önning, Akesson and Oste 128 ) studied the effects of different milks (oat, soya and cows’) on the antioxidant status (ABTS assay), among other variables, in twenty-four healthy males and females. Half of the subjects consumed 0·75–1 litres/d of oat and soya milk for 4 weeks each, and the other half consumed oat and cows’ milk during two periods of 4 weeks. Again, no consistent change in the antioxidant status was observed after consumption, regardless of the diet followed.
Finally, milk may be a relevant source of Se, which is a potential antioxidant and an antioxidant enzyme cofactor. To further explore the effects of Se in milk, Ravn-Haren et al.( Reference Ravn-Haren, Bugel and Krath 129 ) carried out a randomised, double-blind, cross-over study with a 4 x 1-week design, in which young healthy men supplemented their usual diet with either Se-enriched milk, Se-enriched yeast, selenate or placebo (non-enriched milk). The milk was prepared by giving cows a high dose of selenomethionine( Reference Hoac, Stagsted and Lundh 130 ), and most of the Se in milk was protein bound. All sources of Se increased the serum Se levels after supplementation for 1 week, but the effect of the two organic forms was more pronounced than that of selenate. Conversely, thrombocyte glutathione peroxidase was increased during periods in which the subjects were supplemented with selenate but not during those in which they received Se-enriched yeast or milk. No effect was found on the resistance of plasma lipids to oxidation or on the activities of glutathione peroxidase, glutathione reductase and glutathione S-transferase as measured in erythrocytes. Several other variables measured in plasma or leucocytes were also not affected. No specific effects of milk enriched with Se were demonstrated with respect to the Se-enriched yeast, leading the authors to conclude that insufficient information was available to form solid conclusions regarding the in vivo antioxidant potential of milk.
Fermented milk and probiotic yoghurt
As we previously reported in the present review, fermented milk and probiotics may repress oxidative stress, notably due to the production of antioxidant peptides. Several studies have tested the effect of fermented and probiotic milk and yoghurt on the antioxidant status in diabetic or healthy young/adult subjects and in pregnant women.
Ejtahed et al.( Reference Ejtahed, Mohtadi-Nia and Homayouni-Rad 131 ) assessed the effects of conventional v. probiotic yoghurt on the antioxidant status of sixty-four patients with diabetes (type 2, aged 30–60 years). For 6 weeks, the test patients consumed 300 g/d of probiotic yoghurt containing Lactobacillus acidophilus La5 and Bifidobacterium lactis Bb12, and the control patients consumed 300 g/d of plain yoghurt. The probiotic yoghurt significantly increased erythrocyte SOD and blood glutathione peroxidase activities and the total antioxidant status compared with the control plain yoghurt. In addition, in the two groups, the serum concentration of malondialdehydes significantly decreased compared with baseline. However, erythrocyte catalase activity did not change compared with the basal value in the two groups. According to the authors, probiotic yoghurt is a functional food that can exert antioxidant effects.
This next study was based on the observation that pregnancy is associated with elevated levels of oxidative stress and was therefore designed (randomised, single-blind, controlled) to determine the effects of daily consumption of probiotic yoghurt on oxidative stress among Iranian adult pregnant women (18–30 years)( Reference Asemi, Jazayeri and Najafi 132 ). The study was carried out for 9 weeks with subjects consuming 200 g/d of either conventional yoghurt or probiotic yoghurt, using several antioxidant biomarkers. Contrary to plain conventional yoghurt, probiotic-containing yoghurt led to significant changes in the plasma glutathione level (+67·9 μmol/l), serum 8-oxo-7,8-dihydroguanine level (–74·3 ng/ml), erythrocyte glutathione peroxidase activity (+163 nmol/min per ml) and glutathione reductase (+323 nmol/min per ml) activity. However, while conventional yoghurt decreased the total antioxidant capacity, probiotic yoghurt had no significant effect. Despite such differences, between-group differences were only significant for erythrocyte glutathione reductase activity.
The following studies were all carried out in healthy subjects. The effects of probiotic and conventional yoghurts on antioxidant parameters were tested in young healthy women (22–29 years old) consuming 100 g/d of these yoghurts for 2 weeks, followed by 200 g/d for an additional 2 weeks( Reference Fabian and Elmadfa 133 ). The consumption of each yoghurt led to significant decrease in the average antioxidant concentrations (vitamin E, lycopene and zeaxanthin) during the 4 weeks. However, the average concentrations of lutein, β-carotene, albumin, uric acid and bilirubin decreased significantly only in the probiotic group. Such alterations consequently led to a significant reduction in the average total antioxidant capacity during the 4 weeks for both yoghurts. Additionally, the mean plasma levels of malondialdehyde and conjugated dienes increased significantly in the probiotic and control groups. The SOD activity remained essentially constant in both groups throughout the study, and the mean activities of glutathione peroxidase and catalase significantly decreased only in the probiotic group. However, although several parameters changed significantly during the study, no significant differences were observed between the two investigated groups.
The authors suggested that regular intake of lactic acid bacteria results in antioxidant depletion, antioxidant enzyme modulation, and enhanced oxidative stress and cautiously concluded that these results indicate a possible negative influence of probiotics and conventional yoghurt on plasma levels of antioxidants and antioxidant parameters. The significant increase in the concentrations of malondialdehyde and conjugated dienes and the reduction of the plasma antioxidant concentrations ‘might be the result of enhanced oxidative stress caused by the stimulation of the immune system induced by both probiotic bacteria and conventional yoghurt strains’( Reference Fabian and Elmadfa 133 ). In contrast, in the cross-over and double-blind South Korean study by Nam et al.( Reference Nam, Lee and Lee 134 ) performed in twenty-six healthy volunteers consuming a fermented milk or placebo two times/d for 2 weeks, the consumption of fermented milk significantly increased the total antioxidant status of the volunteers and decreased the level of TBARS compared with the period before the consumption of fermented milk.
The objective of another study was to develop a goats’ milk fermented with the human lactobacilli strain, Lactobacillus fermentum ME-3, and to test its effect on oxidative stress markers in the blood and urine of healthy human subjects (35–65 years old)( Reference Kullisaar, Songisepp and Mikelsaar 135 ). Two groups consumed either goats’ milk or fermented goats’ milk groups (150 g/d) for 21 d. Fermentation had significant additive effects by increasing the systemic total antioxidant activity and total antioxidant status and decreasing the baseline value of diene conjugates in the lipoprotein fraction, oxidised LDL, glutathione redox ratio and 8-isoprostanes after 21 d. This pilot study therefore confirmed that the probiotic Lactobacillus fermentum ME-3 can survive in the gastrointestinal tract and exhibits antioxidative effects in human subjects.
Conclusions
Overall, milk-based diets tested in human subjects have no significant effects on antioxidant biomarkers. However, two studies showed antioxidant effects of dairy products, intact milk protein concentrate and hydrolysed whey proteins. Concerning fermented milks, most studies showed improved antioxidant status in human subjects compared with unfermented milks. Finally, whey protein consumption was shown to be correlated with glutathione levels.
Conclusions
Overall, the antioxidant potential of dairy products is of the same order of magnitude as that of some food groups such as grain-based foods, vegetables and fruit juices and some intact vegetable and fruit species (Table 1). A synthesis of the in vitro antioxidant capacity of the main dairy products is presented in Table 2. Among all dairy products, cheeses would present the highest antioxidant potential, probably because of their higher protein content and the fermentation process. This seems to be confirmed in the study by Carlsen et al.( Reference Carlsen, Halvorsen and Holte 6 ) who reported, among more than 3100 foods, that cheeses tend to have on average a slightly higher antioxidant capacity than milks and yoghurts. However, the use of different antioxidant assays (for example, ABTS, FRAP and ORAC) renders dairy product antioxidant values difficult to compare from one study to another.
In humans, the few available studies – together with in vitro and animal studies – suggest that the antioxidant potential of dairy products, rather than milk alone, may contribute to an improved antioxidant status in humans and subsequently to their reported protective effects against some chronic diseases such as obesity, the metabolic syndrome, type 2 diabetes and some CVD. For yoghurts and fermented milks, most in vivo studies support the in vitro findings showing an improved antioxidant status (increased levels of antioxidant enzymes, overall antioxidant capacity, and resistance of LDL to oxidation and decreased levels of malondialdehydes/TBARS) in mice, rats and human subjects after consumption of fermented milk/probiotic yoghurts compared with unfermented milk. However, some studies in human subjects showed no significant effect on antioxidant status, and one study showed increased oxidative stress. However, it is important to keep in mind that an in vivo increase in antioxidant enzymes can also be interpreted as a defence system – compensated by increased levels of antioxidant enzymes – against a potential pro-oxidant effect of some milk fractions.
The present literature review therefore clearly illustrates the lack of human studies exploring the antioxidant potential of dairy products. Therefore, there is still insufficient information from which to draw solid conclusions regarding the antioxidant potential of milk in humans, especially considering the contradictory results of some studies. In addition, the available in vivo studies greatly differ in terms of their objectives and designs and the health status of the animal or human subjects and, as such, cannot be used to reach solid conclusions.
Acknowledgements
The present review received no specific grant from any funding agency in the commercial or not-for-profit sectors.
A. F. drafted the manuscript. E. R. provided critical revision of the manuscript. Both authors contributed to and approved the final version of the manuscript.
A. F. and E. R. declare no conflicts of interest.