Introduction
Inflammatory bowel disease (IBD) is the generic term used to describe a group of chronic diseases characterised by uncontrolled inflammation of the intestinal mucosa( Reference Abraham and Cho 1 ). The aetiology of IBD is currently unknown. However, IBD progression is influenced by genetic factors, dysregulation of immune responses, dysfunction of the mucosal barrier, and loss of immune tolerance to enteric flora( Reference Sanchez-Munoz, Dominguez-Lopez and Yamamoto-Furusho 2 ). Altogether, these factors result in the production of inflammatory mediators such as oxygen and nitrogen reactive species, prostaglandins and cytokines, which can contribute to an uncontrolled inflammatory response that ultimately culminates in irreversible tissue damage. The two main forms of IBD are Crohn’s disease and ulcerative colitis (UC), each differing in location and severity. Crohn’s disease is known to affect multiple sections of the gastrointestinal tract, whereas UC is limited to the rectum and colon( Reference Baliga, Saxena and Kaur 3 , Reference Ferguson and Gentschew 4 ). Furthermore, IBD patients with an extended history of the disease maintain a high risk of developing colon cancer( Reference Francescone, Hou and Grivennikov 5 ). A greater understanding of the pro-inflammatory cytokine networks underlying enteric mucosa inflammation has led to the development of targeted anti-TNF-α therapies. Additionally, recent studies have been aimed at exploring novel treatments for IBD, including regulatory T cell therapy, the use of anti-inflammatory cytokines – for example, IL-10 – and stem cell-based therapies( Reference Duran and Hommes 6 , Reference Plevy and Targan 7 ). Previous epidemiological studies have identified a correlation between the consumption of red wine and a decreased incidence of heart disease, known as the ‘French paradox’( Reference Richard 8 ). This correlation might be due to the presence of resveratrol, a known antioxidant, anti-inflammatory, and anti-proliferative compound, in red wine( Reference Catalgol, Batirel and Taga 9 ). In vitro and in vivo studies have also shown that resveratrol ameliorates IBD by decreasing mucosal inflammation( Reference Lozano-Pérez, Rodriguez-Nogales and Ortiz-Cullera 10 ).
In the present review, we will discuss the effects of resveratrol on IBD pathology, and – by reviewing data from in vitro, animal and human studies – we will describe the mechanisms utilised by resveratrol that mediate its anti-inflammatory activity. We will begin by characterising the natural occurrence and biosynthesis of resveratrol, followed by describing its metabolism. Finally, we will focus on how resveratrol improves the prognosis in patients with IBD.
Resveratrol occurrence and synthesis
Resveratrol (3,4′,5-trihydroxystilbene) is a naturally occurring stilbene found in a variety of plant species (for example, grapes, groundnuts, Japanese knotweed, and several species of berries, such as blueberries, bilberries and cranberries), and exists as both cis- and trans-isomers, the latter being the most commonly found and stable form( Reference Stervbo, Vang and Bonnesen 11 ). The compound was first isolated in 1940 from white hellebore (Veratrum grandiflorum O. Loes)( Reference Takaoka 12 ) and, in 1963, was found in Japanese knotweed (Polygonum cuspidatum), currently one of the principal sources of resveratrol found in nature( Reference King, Bomser and Min 13 ). In 1976, Langcake & Pryce( Reference Langcake and Pryce 14 ) detected, for the first time, trans-resveratrol in grapevines (Vitis vinifera), where resveratrol is synthesised in response to exogenous stimuli such as fungal damage (for example, Botrytis cinerea) or UV light. Resveratrol was later found to be highly photosensitive and susceptible to UV-induced isomerisation, as more than 80 % of trans-resveratrol in solution is converted to cis-resveratrol if exposed to light for 1 h( Reference Vian, Tomao and Gallet 15 ). The final step of resveratrol biosynthesis involves stilbene synthase-mediated condensation of three molecules of malonyl-CoA with one molecule of p-coumaroyl acid, generating trans-resveratrol and four molecules of CO2 ( Reference Planas, Alfaras and Colom 16 ) (Fig. 1). In the 1990s, resveratrol was identified as a component of red wine( Reference Siemann and Creasy 17 ) and, since then, it has been studied extensively. In 2013, Tomé-Carneiro et al. ( Reference Tomé-Carneiro, Larrosa and González-Sarrías 18 ) estimated more than 22500 publications on resveratrol in three databases of literature (PubMed, Scopus, ISI Web of Knowledge). Resveratrol is produced especially in the skins of grapes( Reference Hurst, Glinski and Miller 19 ), and it is extracted in the maceration process during the production of red wine( Reference Gumienna, Lasik and Czarnecki 20 ). The amount of resveratrol present in red wine is influenced by grape variety, soil, climate and winemaking techniques, including manipulation of the amount of time that grape skins remain during the fermentation process( Reference Siemann and Creasy 17 , Reference Zhang, Anderson and Kaushik 21 ). Resveratrol occurs in wine both in its unconjugated, aglycone, form and as resveratrol glucoside (resveratrol-3-O-β-d-glucoside, often referred to as piceid), both in cis and trans arrangements( Reference Lamuela-Raventós, Romero-Pérez and Waterhouse 22 , Reference Romero-Pérez, Ibern-Gómez and Lamuela-Raventós 23 ). The concentration of piceid is approximately three times higher than that of aglycone resveratrol in wines originating from Spain and Portugal( Reference Moreno-Labanda, Mallavia and Pérez-Fons 24 , Reference Ribeiro de Lima, Waffo-Téguo and Teissedre 25 ).
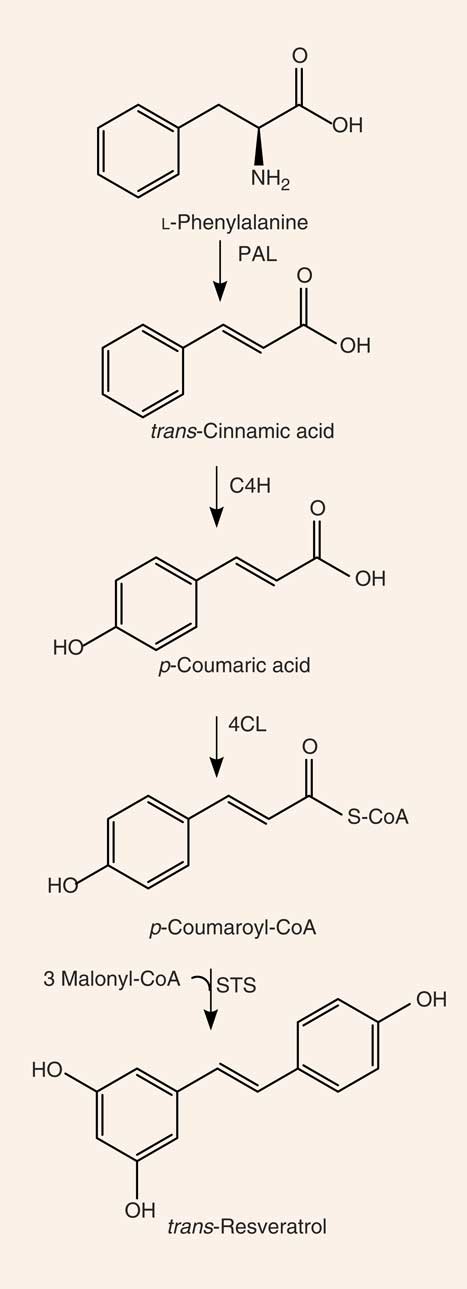
Fig. 1 Synthesis of resveratrol. PAL, phenylalanine ammonia-lyase; C4H, cinnamate 4-hydroxylase; 4CL, 4-coumarate-CoA ligase; STS, stilbene synthase.
Absorption, bioavailability and metabolism of resveratrol
It has been previously demonstrated that phenolic compounds, present in the plasma at low (nanomolar to micromolar) concentrations, are found in the intestine at much higher levels immediately after the consumption of foods and supplements rich in these compounds( Reference Jenner, Rafter and Halliwell 26 ). Thus, phenolic compounds may play a pivotal role in the modulation of the colon microenvironment in both healthy and pathological conditions( Reference Ahmed Nasef, Mehta and Ferguson 27 , Reference Danesi and Ferguson 28 ). Likewise, resveratrol has a rapid rate of uptake and is absorbed in large quantities by enterocytes. However, plasma concentrations of resveratrol are generally low due to high rates of intestinal and hepatic metabolism( Reference Walle 29 ). In one study, the maximum plasma concentration (Cmax) of resveratrol, evaluated in human subjects following administration of a high concentration of resveratrol, was found to be within the range of 0·3 to 2·4 µmol/l after approximately 1·5 h. Here, the authors described two main types of resveratrol metabolites, resveratrol-3-sulfate and resveratrol monoglucuronides, that were found in the plasma at concentrations of up to about 20-fold higher than that of resveratrol itself( Reference Boocock, Faust and Patel 30 ).
Previous studies have also characterised the rate of uptake of resveratrol in vitro and in vivo. Resveratrol, upon entering the small intestine, crosses the enterocyte apical membrane by either passive diffusion or carrier-mediated transport. The aglycone form of resveratrol is absorbed by passive diffusion into human epithelial colorectal adenocarcinoma cells (Caco-2) across the apical membrane, whereas the piceid form is actively transported via Na-dependent transporter proteins( Reference Henry, Vitrac and Decendit 31 ) (Fig. 2). The transepithelial transport of piceid occurs at a higher rate compared with the aglycone form of resveratrol( Reference Henry-Vitrac, Desmoulière and Girard 32 ). Nevertheless, piceid is then hydrolysed by β-glycosidases that are produced by the intestinal microflora, promoting the absorption of the aglycone form( Reference Chukwumah, Walker and Vogler 33 ). Phase II metabolism of resveratrol occurs in the gut, where resveratrol is conjugated with sulfate by sulfotransferase or with glucuronic acid by uridine-5′-diphosphate-glucuronosyltransferase, yielding metabolites resveratrol sulfates and resveratrol glucuronides, respectively( Reference Kaldas, Walle and Walle 34 , Reference Patel, Andreadi and Britton 35 ). Three derived metabolites have been identified: resveratrol-3-glucuronide, resveratrol-4′-O-glucuronide and resveratrol-3-O-sulfate. In addition, intestinal bacteria are able to convert resveratrol to dihydroresveratrol, which is also transported into enterocytes and metabolised to sulfated or glucuronidated forms( Reference Patel, Andreadi and Britton 35 , Reference Maier-Salamon, Hagenauer and Wirth 36 ).

Fig. 2 Absorption and metabolism of resveratrol. After ingestion, resveratrol may be absorbed as glycoside (G-RSV) and then cleaved to the aglycone form of resveratrol (RSV) by cytosolic glucosidase (CBG). Alternatively, this process can occur in the lumen with the action of β-glycosidases (BG) produced by the intestinal microflora (MF). Within enterocytes, RSV is rapidly metabolised to resveratrol glucuronides (RSV-glucuronides) and resveratrol sulfates (RSV-sulfates) via uridine-5′-diphosphate-glucuronosyltransferase (UGT) and sulfotransferase (SULT), respectively. There is some efflux of these metabolites back into the small intestine, which involves multidrug resistance protein (MRP) 2 and breast cancer resistance protein (BCRP) 1. Resveratrol conjugates can also efflux though the basal side of the enterocytes via MRP3. RSV can passively diffuse through the enterocyte basal membrane. Once in the bloodstream, resveratrol and its metabolites reach the liver, where they are further glucuronidated or sulfated. Resveratrol and its conjugates can be recycled back to the small intestine through the bile or excreted via urine. SGLT1, sodium-dependent glucose cotransporter-1; A, albumin.
In an ex vivo study using an isolated rat small intestine perfusion model, Andlauer et al. ( Reference Andlauer, Kolb and Siebert 37 ) showed that 46 % of the luminally administered resveratrol was absorbed by the small intestine, 21 % of which appeared in vascular tissue while another 2 % was located inside the intestine. In the luminal effluent, 40 % of resveratrol was unconjugated, 11 % was glucuronidated, and 3 % was sulfated. Here, a likely explanation for the presence of resveratrol conjugated metabolites in the intestinal lumen is the presence of apically located efflux transporters( Reference Gao, Basu and Yang 38 ), such as multidrug resistance protein (MRP) 2, MRP3 and breast cancer resistance protein (BCRP) 1, which are all present in the membrane of the enterocytes( Reference Englund, Rorsman and Rönnblom 39 ). MRP3 is present in the basolateral membrane of enterocytes, whereas BCRP1 is present in the apical membrane( Reference van de Wetering, Burkon and Feddema 40 ). When BCRP1 is inhibited, the efflux of resveratrol glucuronide and sulfate conjugates is diminished without affecting the absorption of resveratrol. BCRP1 is the most important efflux transporter for sulfate conjugates, as evidenced in BCRP1 knockout mice, where sulfate and glucuronide metabolite efflux was inhibited by 95 and 70 %, respectively( Reference Lançon, Delmas and Osman 41 ). Glucuronidated resveratrol is also a substrate of MRP2( Reference Henry, Vitrac and Decendit 31 ). Kaldas et al. ( Reference Kaldas, Walle and Walle 34 ) showed that treatment with low concentrations of resveratrol resulted in the production of resveratrol metabolites primarily on the apical side of Caco-2 cells. On the other hand, using high concentrations of resveratrol, sulfate conjugates were exported towards the basolateral side, where the MRP3 transporter is localised, due to the saturation of transporters on the apical side. Therefore, the trans-resveratrol conjugates that are not transported to the apical side by MRP2 and BCRP1 are transported by MRP3 into the bloodstream( Reference Juan, González-Pons and Planas 42 ). There, the metabolites are hydrolysed and converted back into an aglycone form by the intestinal microflora, and the excreted compounds can be reabsorbed back into the interior of the enterocytes( Reference Gao, Basu and Yang 38 ).
Once resveratrol has been metabolised by intestinal epithelial cells, it is transported to the liver and enters hepatocytes by passive and carrier-mediated transport( Reference Lançon, Delmas and Osman 41 ). After crossing the hepatocyte membrane, resveratrol is rapidly conjugated and metabolised into its glucuronidated and sulfated derivatives( Reference Lançon, Hanet and Jannin 43 ). All forms of resveratrol are then subsequently excreted in the bile( Reference Maier-Salamon, Hagenauer and Reznicek 44 ).
In the blood, transport of resveratrol is accomplished through its association with lipoproteins, Hb, albumin and serum proteins as it is carried to and from various organs and tissues, followed by elimination in the urine( Reference Jannin, Menzel and Berlot 45 ).
Because resveratrol is extensively metabolised in the gastrointestinal tract and the liver, it will be crucial for future studies to determine if the biological beneficial effects mediated by resveratrol are due not only to the parent compound but also to their metabolites, as has been suggested by Aragonès et al. ( Reference Aragonès, Danesi and Del Rio 46 ) (for a full review, see Del Rio et al. ( Reference Del Rio, Rodriguez-Mateos and Spencer 47 )).
Effects of resveratrol on inflammation and inflammatory bowel disease
Inflammatory bowel disease pathophysiology
The intestinal wall is protected by epithelial cells attached via tight junctions that form a seal against the lumen of the intestinal tract. These epithelia provide a physical barrier between the internal environment and luminal microbes, representing the first line of defence of mucosal immunity( Reference Ramanan and Cadwell 48 ). Impairment of this barrier, due to the dysregulation of tight junctions, leads to increases in permeability and infection( Reference Lee 49 ). Loss of intestinal barrier integrity also promotes abnormal immune and inflammatory responses that are triggered by increased interactions between gut microbiota and the host’s immune system, a key factor that precedes UC and Crohn’s disease (Fig. 3)( Reference Ferguson and Gentschew 4 , Reference Salim and Söderholm 50 , Reference Miner-Williams and Moughan 51 ).
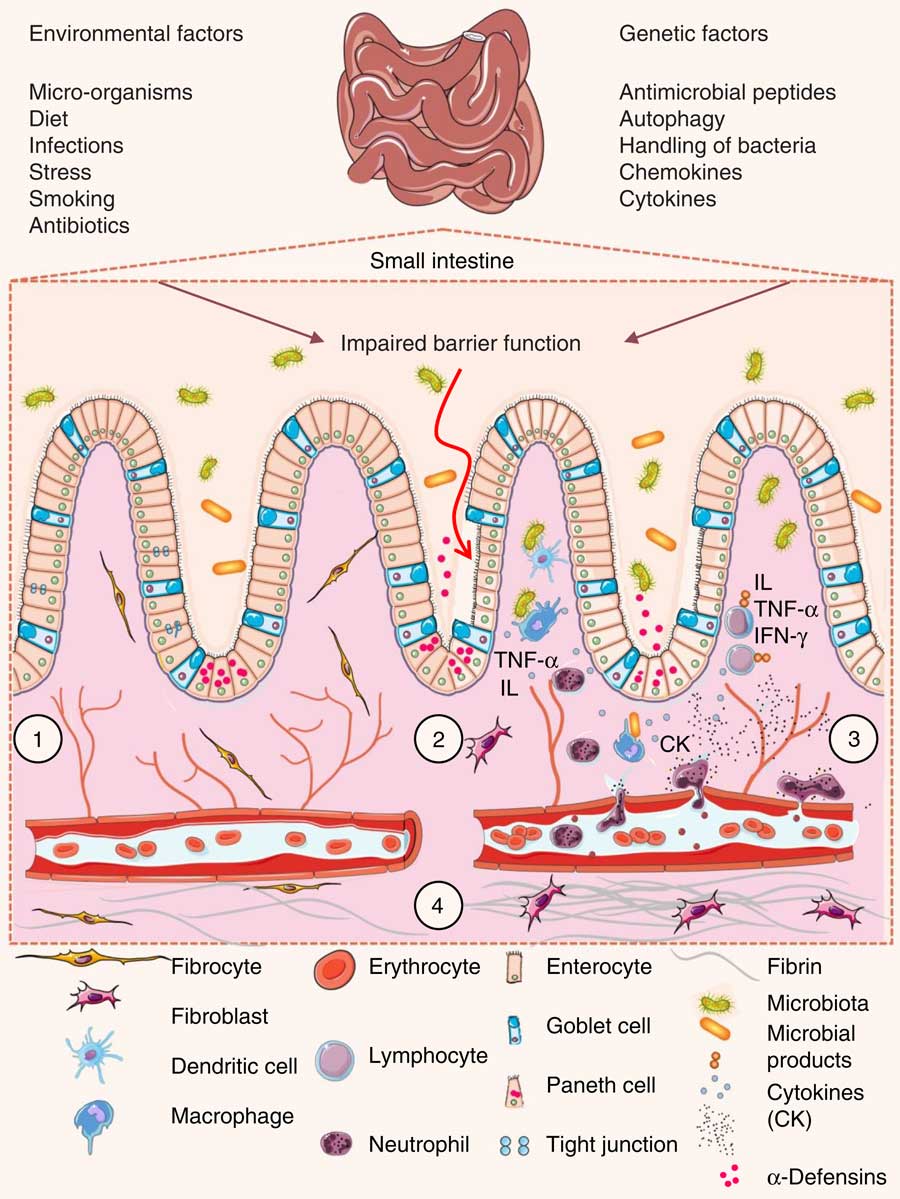
Fig. 3 Pathophysiology of inflammatory bowel disease. (1) The villi and intestinal glands, along with the lamina propria, associated gut-associated lymphoid tissue and muscularis mucosae, constitute the essential features of the small-intestinal mucosa. The glands are composed of a simple columnar epithelium that is continuous with the epithelium of the villi. Intestinal epithelium cells include enterocytes, goblet cells, Paneth cells, enteroendocrine cells and M cells. Tight junctions in the enterocytes establish a barrier between the intestinal lumen and the epithelial intercellular compartment. (2) Genetic and environmental factors induce the disruption of tight junctions, causing increased permeability of the intestinal epithelium and increased uptake of commensal bacteria and microbial products. The recognition of these by macrophages and dendritic cells leads to immune cell activation and cytokine (CK) production. (3) After activation by macrophages and dendritic cells, T-cells produce various interleukins (IL) and TNF-α. (4) If acute mucosal inflammation cannot be resolved by anti-inflammatory mechanisms, chronic intestinal inflammation develops. In turn, chronic inflammation may cause tissue destruction and complications such as fibrosis, stenosis and cancer. IFN-γ, interferon γ.
Luminal microbes that cross the epithelial barrier and multiply within host tissues are recognised by macrophages that reside in the lamina propria of the mucosa. Macrophages act as antigen presenters for T lymphocytes and are responsible for secreting cytokines and chemokines that attract and activate other immune cells, such as lymphocytes and neutrophils( Reference Kühl, Erben and Kredel 52 ). Thus, circulating leucocytes are ‘arrested’ at the site of inflammation due to the production of selectins and factor VIII by endothelial cells. In these instances, selectins allow for the adhesion of lymphocytes and granulocytes to the target tissue, and factor VIII promotes the activation of the complement pathway and the kinin cascade, thereby increasing intestinal permeability( Reference Danese and Fiocchi 53 ). Macrophages and neutrophils represent the primary source of reactive oxygen and nitrogen species present within inflamed colon mucosa( Reference Deniz, Cetinel and Kurtel 54 ). These cells have a reduced NADPH oxidase system that leads to the production of an array of reactive oxygen and nitrogen species( Reference Martín, Villegas and Sánchez-Hidalgo 55 ). Once activated, NADPH oxidase transfers one electron from NADPH to molecular oxygen, yielding one superoxide anion radical (O2 ∙−). Superoxide radicals are then converted into hydrogen peroxide (H2O2) and the hydroxyl radical (HO∙)( Reference Clark, Epperson and Valente 56 ). IBD patients with intestinal inflammation show elevated levels of reactive species. Consequently, mucosal damage caused by high levels of oxidative stress plays a key role in the pathogenesis of IBD( Reference Piechota-Polanczyk and Fichna 57 ).
The nuclear factor κ light-chain-enhancer of activated B cells (NF-κB) is an important regulator of immunity that actively participates in the progression of IBD. Once activated, NF-κB induces the expression of genes involved in inflammation and immunity, such as proinflammatory cytokines (TNF-α, IL-1β, IL-6, IL-12), adhesion molecules, and enzymes such as inducible NO synthase and cyclo-oxygenase-2 (COX-2)( Reference Danese, Sans and Fiocchi 58 ). The induction of cytokine expression by NF-κB is responsible for the stimulation, activation and differentiation of lamina propria immune cells, resulting in persistent mucosal inflammation. For example, TNF-α acts as a positive feedback signal that enhances the production of reactive oxygen and nitrogen species, and NF-κB activation( Reference Schottelius and Dinter 59 ). The uncontrolled production of reactive species in the mucosa appears to play a significant role in IBD disease pathogenesis and is responsible for the onset of IBD-related symptoms, including diarrhoea, toxic megacolon and abdominal pain( Reference Kovacic and Somanathan 60 ).
Another factor that has been shown to affect the pathology of IBD is the IL-23/T helper 17 cells (Th17) pathway. This pathway contributes to immune responses that regulate intestinal inflammation in both animal models of colitis and human patients with IBD( Reference Abraham and Cho 61 ). Importantly, experimental evidence has confirmed the involvement of the IL-12/IL-23 pathway in the pathogenesis of IBD( Reference McGovern and Powrie 62 , Reference Danesi, Philpott and Huebner 63 ).
Effects of resveratrol on inflammation in vitro
In vitro resveratrol’s effects on inflammation are summarised in Table 1. Several groups have previously characterised the anti-inflammatory effects of resveratrol in intestinal cells. One study focused on the protective effects of moderate to high concentrations of resveratrol (10–50 µm) in Caco-2 cells exposed to bacterial-derived lipopolysaccharides. Here, it was shown that, in resveratrol-treated cells, lower levels of PGE2 correlated with a decrease in COX-2 expression. Furthermore, resveratrol inhibited NF-κB activation by reducing the rate of degradation of IκB, an endogenous NF-κB inhibitor( Reference Cianciulli, Calvello and Cavallo 64 ). A similar effect of resveratrol on the activation of NF-κB was observed in Caco-2 cells and SW480 human colon adenocarcinoma cells treated with lipopolysaccharides. High concentrations of resveratrol (40 µm) abrogated the inflammatory responses of both cell types by reducing the expression of Toll-like receptor 4 and inducible NO synthase and decreasing the rate of IκB-α degradation( Reference Panaro, Carofiglio and Acquafredda 65 ). Conversely, treatment with resveratrol (50 μm) did not inhibit NF-κB activation in a different study that used Caco-2 cells but instead exacerbated inflammatory responses( Reference Romier, Van De Walle and During 66 ).
Table 1 Summary of the effects of resveratrol on inflammation assayed in intestinal cell studies

LPS, lipopolysaccharide; ↓, decrease; COX-2, cyclo-oxygenase 2; mRNA, messenger RNA; iNOS, inducible NO synthase; TLR-4, Toll-like receptor 4; ↑, increase; p-, phosphorylated; IκB, inhibitor of NF-κB; IFN-γ, interferon-γ; ↔ , no change; STAT1, signal transducer and activator of transcription 1; SAPK, stress-activated protein kinase; JNK, c-Jun N-terminal kinase; ROS, reactive oxygen species; Nrf2, nuclear factor (erythroid-derived-2)-like 2; HO-1, haeme oxygenase 1; GCLC, glutamate-cysteine ligase catalytic subunit; GCLM, glutamate-cysteine ligase modifier subunit; GSH, reduced glutathione; GSSG, oxidised glutathione.
5-Aminosalicylic acid (5-ASA) is a well-known pharmacological compound used to treat IBD patients. One study assessed the effects of pre-treatment with resveratrol and/or 5-ASA in HT-29 human colorectal adenocarcinoma cells after exposure to varying combinations of pro-inflammatory cytokines. At a concentration of 25 µm, resveratrol reduced PGE2 production, inducible NO synthase and COX-2 expression, reactive species formation( Reference Serra, Rufino and Mendes 67 ), and activated the Nrf2 pathway, inducing the expression of antioxidant and cytoprotective enzymes (haeme oxygenase 1 and glutamate cysteine ligase)( Reference Serra, Almeida and Dinis 68 ). Likewise, resveratrol efficiently decreased the expression of phosphorylated signal transducer and activator of transcription (STAT) 1, suggesting that the Janus kinase/signal transducers and activators of transcription (JAK-STAT) pathway is a key mediator of resveratrol’s anti-inflammatory activity( Reference Serra, Rufino and Mendes 67 ).
Mitochondrial dysfunction plays an important role in IBD pathogenesis, as oxidative stress and impaired ATP production are key players in the development and progression of the disease. Resveratrol, applied at extremely high concentrations (440 µm), protected Caco-2 cells against indomethacin-induced mitochondrial dysfunction( Reference Carrasco-Pozo, Mizgier and Speisky 69 , Reference Carrasco-Pozo, Pastene and Vergara 70 ). Because resveratrol has an analogous structure to rotenone (a complex I inhibitor), it has been proposed that one of the mechanisms whereby resveratrol protects against mitochondrial dysfunction is through binding to the ubiquinone site of complex I, thus inhibiting the interaction between indomethacin and rotenone( Reference Carrasco-Pozo, Mizgier and Speisky 69 ). In agreement, resveratrol has been shown to inhibit the mobilisation of Ca2+, the release of caspase-3, -9, and cytochrome c from the mitochondria, and the induction of apoptosis in response to cytotoxic concentrations of indomethacin( Reference Carrasco-Pozo, Pastene and Vergara 70 ). Therefore, resveratrol appears to potently inhibit mitochondrial dysfunction( Reference Ungvari, Sonntag and de Cabo 71 ) and prevent the onset of programmed cell death.
Resveratrol has also been tested in cultured cells derived from patients with chronic obstructive pulmonary disease (COPD), another disease characterised by the dysregulation of immune responses. Culpitt et al. ( Reference Culpitt, Rogers and Fenwick 72 ) showed that resveratrol, at very high concentrations (about 600 µm), inhibits inflammatory cytokine release from alveolar macrophages isolated from patients with COPD and alleviates inflammation.
With regards to the discrepancies in the concentrations of resveratrol used in each study, it has been shown that administration of 0·5 g/d of resveratrol resulted in plasma concentrations ranging from 2·4 µm (for a single dose)( Reference Boocock, Faust and Patel 30 ) to 4·24 µm (for repeated doses)( Reference Brown, Patel and Viskaduraki 73 ). Unfortunately, many in vitro studies have used relatively high concentrations of the compound. Therefore, it will be important for future research to focus on using physiological conditions( Reference Aragonès, Danesi and Del Rio 46 ) in order to verify if resveratrol, at concentrations normally found in serum, retains its anti-inflammatory properties. However, resveratrol concentrations within the environment of the gastrointestinal tract vary widely among different patients and are frequently higher than those found in the blood and peripheral tissues( Reference Singh, Ndiaye and Ahmad 74 , Reference Patel, Brown and Jones 75 ). Accordingly, evaluation of resveratrol uptake and analysis of its metabolites will be a crucial step in order to define the most effective concentration of resveratrol for testing in vitro ( Reference Chachay, Kirkpatrick and Hickman 76 ).
In conclusion, in vitro studies show that resveratrol, at moderate to high concentrations, can modulate inflammatory responses within intestinal cells by down-regulating NF-κB activation and preventing mitochondrial dysfunction, providing evidence for its potential use as a novel anti-inflammatory compound in the framework of IBD.
Effects of resveratrol on inflammation in animal studies
In vivo studies using models for inflammatory intestinal chronic diseases have contributed to our understanding of the effects of resveratrol in IBD (Table 2). In a rat model of chronic inflammation induced by 2,4,6-trinitrobenzenesulfonic acid (TNBS), Martín et al. ( Reference Martín, Villegas and Sánchez-Hidalgo 55 ) demonstrated that resveratrol attenuated intestinal mucosal damage by reducing the recruitment of neutrophils and the secretion of TNF-α. Furthermore, resveratrol decreased the production of PGE2 and PGD2 to basal levels, reduced COX-2 expression, and stimulated apoptosis in colon mucosa during early stages of the disease( Reference Martín, Villegas and Sánchez-Hidalgo 55 ). In a previous study, these authors also demonstrated the efficacy of resveratrol in early colon inflammation induced by TNBS in rats( Reference Martín, Villegas and La Casa 77 ). In another study using TNBS as a promoter of UC in rats, resveratrol inhibited the activity of intercellular adhesion molecule-1 and vascular cell adhesion protein-1, thereby preventing neutrophil infiltration due to the weakened interaction between leucocytes and endothelial cells( Reference Abdallah and Ismael 78 ). Additionally, Yildiz et al. ( Reference Yildiz, Yildiz and Ulutas 79 ) showed that intraperitoneal pre-treatment with resveratrol for 5 d before the induction of UC by TNBS significantly reduced the microscopy injury score and malondialdehyde levels, and increased glutathione peroxidase activity.
Table 2 Overview of the effects of resveratrol (RES) in experimental animal models of inflammatory bowel disease
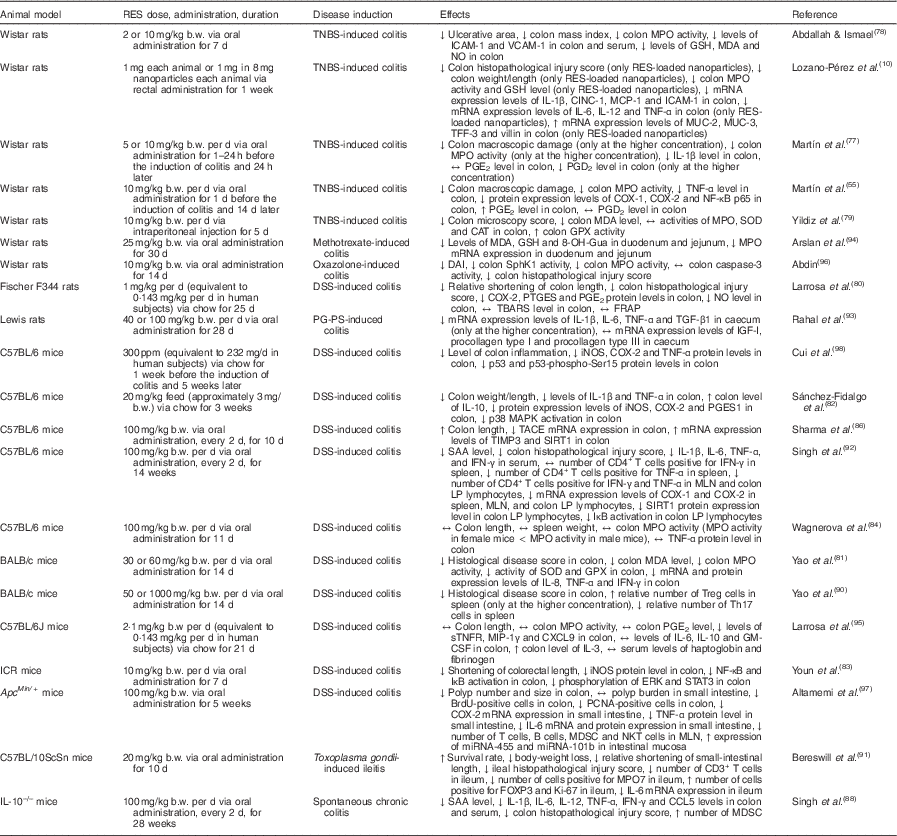
↑, Increase; ↔ , no change; ↓, decrease; 8-OH-Gua, 8-hydroxyguanine; b.w., body weight; BrdU, bromodeoxyuridine; CAT, catalase; CD3, cluster of differentiation 3; CD4, cluster of differentiation 4; CINC-1, cytokine-induced neutrophil chemoattractant 1; COX-1, cyclo-oxygenase-1; COX-2, cyclo-oxygenase-2; CXCL9, chemokine (C-X-C motif) ligand 9; DAI, disease activity index; DSS, dextran sulfate sodium; ERK, extracellular signal-regulated kinase; FOXP3, forkhead box P3; FRAP, ferric-reducing ability of plasma; GM-CSF, granulocyte-macrophage colony-stimulating factor; GPX, glutathione peroxidase; GSH, reduced glutathione; ICAM-1, intercellular adhesion molecule 1; ICR, Institute for Cancer Research; IFN-γ, interferon-γ; IGF-I, insulin-like growth factor 1; iNOS, inducible NO synthase; IκB, inhibitor of NF-κB; Ki-67, protein encoded by MKI67, marker of proliferation Ki-67 gene; LP, lamina propria; MAPK, mitogen-activated protein kinase; MCP-1, monocyte chemoattractant protein 1; MDA, malondialdehyde; MDSC, myeloid-derived suppressor cells; MIP-1γ, macrophage inflammatory protein-1 γ; miRNA, microRNA; MLN, mesenteric lymph nodes; MPO, myeloperoxidase; mRNA, messenger RNA; MUC-2, mucin 2; MUC-3, mucin 3; NF-κB, nuclear factor κ light-chain-enhancer of activated B cells; NKT, natural killer T; p38, p38 protein; p53, p53 protein; p53-phospho-Ser15, p53 protein phosphorylated at Ser15; p65, p65 protein; PCNA, proliferating cell nuclear antigen; PGES1, PGE synthase 1; PG-PS, peptidoglycan-polysaccharide; ppm, parts per million; PTGES, PGE synthase; SAA, serum amyloid A; SIRT1, sirtuin 1; SOD, superoxide dismutase; SphK1, sphingosine kinase 1; STAT3, signal transducer and activator of transcription 3; sTNFR, soluble TNF receptors; TACE, TNF-α-converting enzyme; TBARS, thiobarbituric acid-reactive substances; TFF-3, trefoil factor 3; TGF-β1, transforming growth factor β1; Th17, T helper 17 cells; TIMP3, metalloproteinase inhibitor 3; TNBS, 2,4,6-trinitrobenzenesulfonic acid; Treg, regulatory T cells; VCAM-1, vascular cell adhesion molecule 1.
Larrosa et al. ( Reference Larrosa, Yañéz-Gascón and Selma 80 ), using a dextran sulfate sodium (DSS)-induced colitis rat model, explored the hypothesis that resveratrol exerts anti-inflammatory effects in vivo at an attainable dietary dose. Their microarray analysis revealed that 6 % of the total genes analysed from distal colon mucosa were significantly regulated by resveratrol treatment, including those involved in IL-6 signalling, apoptosis, mitochondrial fatty acid oxidation and Wnt signalling. These results strengthen the importance of resveratrol as a multi-target anti-inflammatory compound, suggesting that resveratrol is not only a promising anti-inflammatory compound at pharmacological doses, but also at doses attained through normal ingestion of resveratrol-rich foods.
Several groups have attempted to describe resveratrol’s antioxidative effects in vivo, including one study that used a DSS-induced UC mouse model. These authors found that resveratrol ameliorated UC, decreased mucosal inflammation, and increased the activity of superoxide dismutase and glutathione peroxidase, demonstrating that resveratrol can modulate the expression of antioxidant enzymes. In these mice, myeloperoxidase activity and IL-8, TNF-α and interferon-γ production were significantly reduced in a dose-dependent manner in response to resveratrol( Reference Yao, Wang and Liu 81 ). Resveratrol also improved clinical symptoms related to DSS-induced chronic inflammation, such as loss of body weight, diarrhoea and rectal bleeding. Moreover, the rate of mortality was significantly reduced in mice treated with both DSS and resveratrol compared with those that were exposed to DSS alone( Reference Sánchez-Fidalgo, Cárdeno and Villegas 82 ). Similarly, oral administration of resveratrol can prevent the onset of DSS-induced mouse colitis by down-regulating the phosphorylation of extracellular signal-regulated kinase and STAT3, two key mediators of NF-κB signalling and inflammation( Reference Youn, Lee and Na 83 ). Interestingly, Wagnerova et al. ( Reference Wagnerova, Babickova and Liptak 84 ) discovered some sex-based differences in the biological effects of resveratrol tested in mice treated with DSS. In mice exposed to DSS and resveratrol, the myeloperoxidase activity in the colon of females was lower compared with that in males. The dissimilarity is probably related to the modulation of the neutrophils’ activity by sex hormones.
An overexpression of TNF-α-converting enzyme (TACE) has been observed in the colon tissue of human subjects with IBD( Reference Freour, Jarry and Bach-Ngohou 85 ), and evidence suggests that resveratrol can ameliorate the intestinal inflammation, through inhibition of TACE, in C57BL/6 mice with DSS-induced colitis( Reference Sharma, Mohapatra and Wagh 86 ).
The IL-10–/– mouse model lacks a functional version of the anti-inflammatory cytokine IL-10( Reference Kühn, Löhler and Rennick 87 ), and has been widely used as a model of IBD. Singh et al. ( Reference Singh, Singh and Singh 88 ) showed that the oral administration of resveratrol ameliorates chronic colitis in IL-10–/– mice by the induction of myeloid-derived suppressor cells (MDSC) and lowering of both mucosal and systemic inflammatory cytokine responses. MDSC possess strong immunosuppressive activities( Reference Haile, von Wasielewski and Gamrekelashvili 89 ), and their modulation has potential therapeutic effects on inflammatory diseases such as IBD.
Administration of moderate to high doses of resveratrol (50 and 100 mg/kg) was able to regulate the imbalance between Th17 and regulatory T (Treg) cells through reducing the number of Th17 cells and up-regulating the number of Treg cells in a DSS murine model of UC( Reference Yao, Wei and Wang 90 ).
A Toxoplasma gondii inflammation model has also been used to study the protective effects of resveratrol. Bereswill et al. ( Reference Bereswill, Muñoz and Fischer 91 ) observed that administration of resveratrol results in: (i) a high proliferation rate of intestinal epithelial cells in the ileal mucosa, (ii) a reduction of CD3+ T lymphocytes, (iii) a decrease in the levels of inflammatory cytokines such as interferon-γ and TNF-α in the lamina propria, (iv) a reduction in neutrophil recruitment, and (v) a reduction in reactive species production. Furthermore, treatment with resveratrol induced changes in the gut microbiota (fewer proinflammatory enterobacteria and enterococci, and higher anti-inflammatory lactobacilli and bifidobacteria) and a decrease in bacterial translocation( Reference Bereswill, Muñoz and Fischer 91 ). It also up-regulated sirtuin 1, suppressed Th1 lymphocytes( Reference Singh, Singh and Singh 92 ), and decreased the levels of transforming growth factor β1, all of which led to a decrease in caecal wall fibrosis( Reference Rahal, Schmiedlin-Ren and Adler 93 ).
Arslan et al. ( Reference Arslan, Ozcicek and Keskin Cimen 94 ) found that resveratrol is able to protect against gastrointestinal toxicity of chemotherapeutic agents such as methotrexate, demonstrating that it can reduce the oxidative stress and induce antioxidant responses in duodenal and jejunal tissues.
The application of resveratrol is limited because of its low oral bioavailability. Thus, attempts to increase its bioavailability include its encapsulation in silk fibroin nanoparticles. Administration of resveratrol nanoparticles in rats treated with TBNS and forced to undergo IBD resulted in an increased anti-inflammatory effect compared with treatment with resveratrol alone, underlining a synergy between the particles and resveratrol or an enhanced anti-inflammatory effect of resveratrol. Unsurprisingly, mice treated with encapsulated resveratrol exhibited decreases in myeloperoxidase activity and expression of TNF-α, IL-1, IL-6 and IL-12. Thus, the use of silk fibroin nanoparticles may be an attractive strategy for the controlled release of resveratrol to target intestinal inflammation( Reference Lozano-Pérez, Rodriguez-Nogales and Ortiz-Cullera 10 ). Yet another approach to increasing resveratrol bioavailability involved using a DSS murine model of inflammation. Here, resveratrol prodrugs and pro-prodrugs were applied to decrease resveratrol metabolism and to increase the availability of resveratrol in the colon. These prodrugs and pro-prodrugs restored mucosal barrier function in response to DSS and enhanced the beneficial effects of resveratrol on colon mucosa( Reference Larrosa, Tomé-Carneiro and Yáñez-Gascón 95 ). Altogether, it appears that further investigation into the potential effects of prodrugs and nanoparticles as methods to improve resveratrol’s bioavailability is warranted.
Patients with IBD have an elevated risk of developing colon cancer. Abdin( Reference Abdin 96 ) carried out a study to investigate the effects of resveratrol on sphingosine kinase 1 (SphK1) activity and apoptosis using a rat model of oxazolone-induced UC. SphK1 has been reported to mediate inflammatory, pro-survival and pro-proliferative signalling pathways. This study demonstrated that the activation of SphK1 plays a key role in the pathogenesis of UC and confers an increased risk of colon tumorigenesis. Furthermore, resveratrol was shown to reduce inflammation and apoptosis, possibly due to the inhibition of SphK1( Reference Abdin 96 ). Additionally, it has been shown by Ibrahim Altamemi et al. ( Reference Altamemi, Murphy and Catroppo 97 ) that resveratrol inhibited the formation of polyps, and reduced cell damage and proliferation of epithelial cells in the intestinal mucosa in mice exposed to DSS. In this study, resveratrol also decreased the presence of inflammatory cells (T, B and natural killer T lymphocytes) and decreased the levels of circulating cytokines. Finally, microarray analysis identified two microRNA (miRNA), miRNA-101b and miRNA-455, with anti-inflammatory properties that were up-regulated in response to resveratrol( Reference Altamemi, Murphy and Catroppo 97 ). Similarly, Cui et al. ( Reference Cui, Jin and Hofseth 98 ) observed that resveratrol improves the intestinal inflammation and down-regulates the markers of inflammation (inducible NO synthase, COX-2 and TNF-α) and the sensors of inflammatory stress (p53 and p53-phospho-Ser15) in mice treated with DSS. Tumour incidence and multiplicity also decreased with resveratrol treatment.
In summary, resveratrol has been shown to have potent anti-inflammatory effects in vivo. It decreases neutrophil infiltration in the intestinal mucosa, inhibits TNF-α production and NF-κB activation, and represses intestinal tumorigenesis by regulating anti-inflammatory miRNA. Altogether, these results indicate that resveratrol could be used to prevent inflammation and reduce the risk of colon carcinogenesis in IBD.
Resveratrol in human clinical trials
Human clinical trials of the potential therapeutic, or, better, ameliorating effects of resveratrol in IBD are few (Table 3). Recently, a randomised, double-blind, placebo-controlled clinical trial evaluating the effects of resveratrol supplementation on inflammatory biomarkers and the quality of life of patients with UC was carried out. In this study, a dose of 0·5 g/d of resveratrol was given to patients for 6 weeks. The authors observed that the plasma levels of high-sensitivity C-reactive protein in the resveratrol-treated group were reduced. Furthermore, plasma TNF-α and NF-κB p65 levels were decreased in response to resveratrol. The quality of life of these patients was improved, and the clinical colitis activity index score was significantly decreased when compared with the placebo group( Reference Samsami-Kor, Daryani and Asl 99 ). Furthermore, the oxidative status of patients with mild to moderate UC markedly improved – activity of superoxide dismutase and total antioxidant capacity were increased, whereas antioxidant malondialdehyde levels were decreased – in patients who were given resveratrol( Reference Samsamikor, Daryani and Asl 100 ).
Table 3 Summary of findings related to resveratrol intake in patients with inflammatory bowel disease

UC, ulcerative colitis; F, female; M, male; ↓, decrease; hs-CRP, high-sensitivity C-reactive protein; PBMC, peripheral blood mononuclear cells; ↑, increase; IBDQ-9, nine-item inflammatory bowel disease questionnaire; ↔, no change; SCCAI, simple clinical colitis activity index; SOD, superoxide dismutase; TAC, total antioxidant capacity; MDA, malondialdehyde.
In another study, patients with colorectal cancer were given daily doses of resveratrol of 0·5 and 1 g/d for 8 d before surgery. Interestingly, the levels of both resveratrol and its metabolites that were found in biopsy tissue samples were similar to the concentrations used in order to reduce tumorigenesis in vitro ( Reference Patel, Brown and Jones 75 ).
Taken together, these studies suggest that resveratrol can improve the quality of life of patients with IBD, and decrease inflammation and oxidative stress.
Conclusions
A number of in vitro and animal studies have shown that resveratrol may reduce the severity of intestinal inflammation in models of IBD. The beneficial effects of resveratrol were attributed to a variety of mechanisms that ultimately lead to the inhibition of several key components of the inflammatory cascade. The most commonly described effects were inhibition of NF-κB activation, decreased COX-2 expression, reduction of proinflammatory cytokines, decreased PGE2, and PGD2 levels and neutrophil infiltration, and attenuation of reactive species production. Resveratrol has also been shown to promote the reduction of bacterial translocation and to alter the intestinal microbial composition. Resveratrol also appeared to inhibit tumorigenesis by modulating a variety of signal transduction pathways and programmed cell death (Fig. 4). Nevertheless, the limited use of resveratrol metabolites in in vitro studies, the doses applied in animal models, and, most of all, the paucity of human intervention studies drastically limit the relevance of these results, as a real translation into a preventative strategy towards IBD is far from confirmed. For this reason, if resveratrol has to be kept within the list of dietary phytochemicals with a relevant anti-inflammatory effect in the framework of IBD, future research should focus on three main topics: (i) increasing the efficacy and bioavailability of resveratrol, (ii) investigating the anti-inflammatory effects of resveratrol metabolites on chronic inflammatory diseases such as IBD, while also determining possible antagonistic or synergistic interactions of these metabolites with resveratrol, and (iii) performing a number of trials in human subjects providing resveratrol as a strategic phytochemical to prevent or as a complementary treatment for IBD.
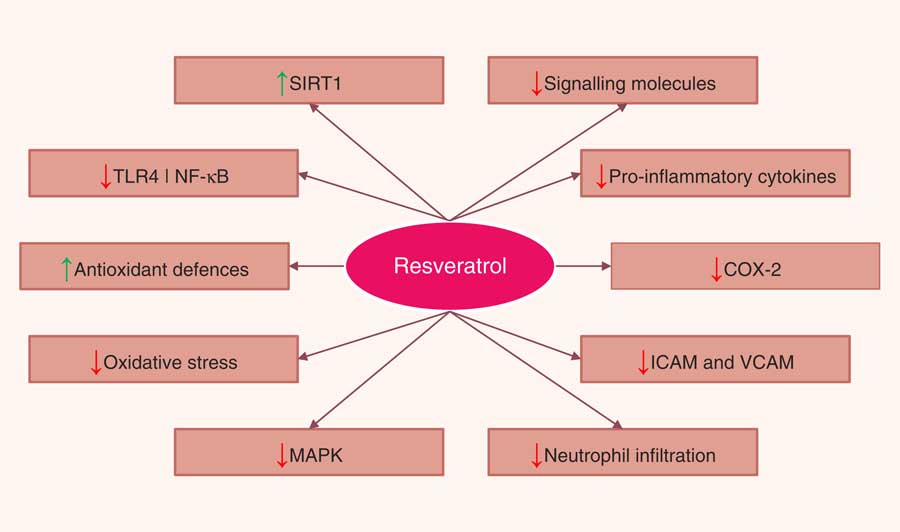
Fig. 4 Pathways regulated by resveratrol in the intestinal mucosa. SIRT1, sirtuin 1; TLR4, Toll-like receptor 4; NF-κB, nuclear factor κ light-chain-enhancer of activated B cells; COX-2, cyclo-oxygenase 2; ICAM, intercellular adhesion molecule; VCAM, vascular cell adhesion molecule; MAPK, mitogen-activated protein kinase.
Acknowledgements
The present study was partially funded by the Italian Ministry of Education, University and Research MIUR – SIR Programme (F. D., grant number RBSI14LHMB).
All authors contributed equally to the preparation of the paper.
There were no conflicts of interest.