Introduction
Non-alcoholic fatty liver disease (NAFLD) is now the most common cause of chronic liver disease and a significant public health problem worldwide. Closely associated with obesity, the global prevalence of NAFLD is estimated to be 24%, placing a significant clinical and economic burden on many countries, including the United Kingdom(Reference Younossi, Anstee and Marietti1,Reference Younossi, Blissett and Blissett2) . Defined physiologically by excess accumulation of lipids in the liver, NAFLD is an umbrella term encompassing a range of histopathology from hepatic steatosis (non-alcoholic fatty liver, NAFL), to non-alcoholic steatohepatitis (NASH), fibrosis and cirrhosis. There is tremendous inter-individual variation both in disease phenotype and its progression, determined by dynamic interactions between genetic, metabolic and environmental factors that are not completely understood(Reference Moore3,Reference Eslam, Sanyal and George4) . Although several genetic variants have been identified that influence NAFLD susceptibility, dietary and lifestyle factors strongly influence disease progression, and weight loss is the mainstay of current clinical management guidelines(5–Reference Chalasani, Younossi and Lavine7).
The integral relationship between metabolic dysfunction and NAFLD, along with its complex and variable pathogenesis between individuals, prompted a consensus-driven proposal in April 2020 for a name change to ‘metabolic associated fatty liver disease’ (MAFLD)(Reference Eslam, Sanyal and George4,Reference Eslam, Newsome and Sarin8) . More than a name change, this would change the diagnostic criteria from being exclusionary (absence of excess alcohol intake and other chronic liver diseases) to a positive diagnosis of: hepatic steatosis alongside either overweight/obesity, type 2 diabetes, or the presence of two metabolic risk factors in normal-weight individuals(Reference Eslam, Newsome and Sarin8). Importantly, the recognition of the heterogeneity of NAFLD presentation and the disease as a continuum rather than a dichotomous stratification between NASH and non-NASH would permit the recognition of the coexistence of metabolic and other chronic liver diseases (including alcohol-related fatty liver), and potentially present opportunities for improved clinical trial designs(Reference Eslam, Sanyal and George4,Reference Eslam, Newsome and Sarin8) . Multiple positive endorsements for the proposal have been published in recent months, from patients(Reference Shiha, Korenjak and Eskridge9), nurses(Reference Clayton, F, abrellas and Luo10), and professional associations(Reference Spearman, Desalegn and Ocama11–Reference Mendez-Sanchez, Arrese and Gadano13). Nonetheless, time is required to update International Classification of Diseases (ICD) diagnostic codes. At the time of writing, the most recent codes (ICD-11, 09/2020) refer only to NAFLD and NASH; therefore, we use these terms here.
Individual dietary nutrients have been implicated in NAFLD pathogenesis and may differentially affect disease development and/or progression. For example, separate from their provision of energy, dietary macronutrients (saturated and n-3 fatty acids, fructose) appear to differentially effect lipid accumulation in the liver through multiple molecular and cellular mechanisms(Reference Parry and Hodson14,Reference Yki-Järvinen, Luukkonen and Hodson15) . Similarly, micronutrient deficiencies have been associated with NAFLD, and a mechanistic basis exists for their therapeutic targeting(Reference Pickett-Blakely, Young and Carr16). However, to date, only a limited number of intervention trials have examined dietary supplements in patients with NAFLD, with mixed results(Reference Moore3,Reference Pickett-Blakely, Young and Carr16) . Vitamin D is of interest in part because it has anti-proliferative, anti-inflammatory and anti-fibrotic properties that have been shown to attenuate NAFLD progression in pre-clinical models(Reference Pacifico, Osborn and Bonci17,Reference Karatayli, Stokes and Lammert18) . Moreover, low levels of dietary vitamin D intakes and poor vitamin D status are widespread and are notably observed in paediatric NAFLD(Reference Gibson, Lang and Gilbert19–Reference Zhu, Wang and Luo21). However, intervention trials of vitamin D supplementation in patients with NAFLD have been heterogeneous in their duration, dosing and outcome measurements, and questions remain about optimal regimens for efficacy in chronic liver disease(Reference Zhang, Thorne and Moore22).
The molecular pathogenesis of NAFLD is complex, involving numerous signalling molecules involved in hepatic metabolism, oxidative, inflammatory and fibrotic processes(Reference Moore3). These include microRNAs (miRNAs) that play an essential role in gene expression and regulatory networks involved in lipid and carbohydrate metabolism and cellular stress response pathways(Reference Gjorgjieva, Sobolewski and Dolicka23). Dysregulation of miRNA expression in the liver is associated with hepatic inflammation, fibrosis and the development and progression of multiple liver diseases, including hepatocellular carcinoma (HCC)(Reference Wang, He and Mackowiak24,Reference Oura, Morishita and Masaki25) . Separately, a body of evidence exists for miRNAs in mediating the cellular response to vitamin D, including the post-transcriptional regulation of the vitamin D receptor (VDR) and vitamin D’s anti-cancer properties(Reference Zeljic, Supic and Magic26). Interestingly, while the anti-cancer effects of vitamin D have been observed in liver(Reference Chiang, Yeh and Chen27,Reference Wu, Wang and Chen28) , the potential role of vitamin-D-regulated miRNAs in NAFLD remains unexplored.
Therefore, the aims of the present paper were to first comprehensively review the data from human studies for involvement of miRNA in NAFLD pathogenesis. Secondly, we aimed to review serum miRNA profiling studies that have examined vitamin D status or response to supplementation, along with the limited research that has investigated miRNAs and vitamin D in the context of liver pathology. Finally, integrating these data, this paper aimed to critically assess the evidence for a potential subset of miRNAs that are both dysregulated in NAFLD and modulated by vitamin D.
MicroRNAs in health and disease
Typically only twenty-two nucleotides long(Reference Fang, Du and Edwards29), miRNAs are small, non-coding RNAs that are critical for development, regulate a variety of normal physiological processes, and are found dysregulated in cancer(Reference Goodall and Wickramasinghe30), liver(Reference Wang, He and Mackowiak24) and metabolic diseases(Reference Ji and Guo31). They play a key role in the post-transcriptional regulation of gene expression, binding complementary sequences within mRNA transcripts and typically suppressing gene expression through mRNA degradation and translation repression. Recent estimates suggest there are approximately 2300 human miRNAs(Reference Alles, Fehlmann and Fischer32). However, miRNAs can have numerous mRNA targets, and individual mRNAs can be targeted by several miRNAs. Indeed, miRNAs regulate an estimated 60% of human protein coding genes impacting almost all biological processes(Reference Friedman, Farh and Burge33,Reference Chipman and Pasquinelli34) .
Primary miRNAs (pri-miRNAs) are transcribed and processed in the nucleus into pre-miRNA molecules that contain a characteristic hairpin structure. After export into the cytoplasm, pre-miRNAs are processed into miRNA duplexes by the Dicer enzyme. During the subsequent multistep assembly of the RNA-induced silencing complex, only one of the miRNA strands (the guide strand) of these duplexes will be retained, becoming the mature miRNA targeting complimentary mRNA for repression(Reference Kobayashi and Tomari35). By current nomenclature conventions, mature miRNAs are given the prefix ‘miR’ and a number indicating identification order; family members with nearly identical sequences expressed from different precursors or genomic loci have lettered suffixes (e.g. miR-30b and miR-30c)(Reference Budak, Bulut and Kantar36). Mature miRNAs derived from the 5′ and 3′ arms of the pre-miRNA hairpin structure are annotated with the suffixes –5p and –3p, respectively. Although both the –5p and –3p forms may be functional depending on context, experimental data suggest the 5p form is more frequently found as mature miRNAs(Reference Alles, Fehlmann and Fischer32,Reference Chiang, Schoenfeld and Ruby37) .
Similar to mRNA, the expression of miRNAs varies by cell and tissue type; and while some miRNAs are ubiquitously expressed, others are tissue specific(Reference Ludwig, Leidinger and Becker38). For example, miR-122 is specifically and highly expressed in the liver, representing a remarkable 70% of liver miRNA content(Reference Jopling39). Present in blood, urine and other body fluids(Reference Weber, Baxter and Zhang40), miRNA levels have been shown to be altered in multiple diseases as a result of genomic events or alterations in miRNA biogenesis, prompting significant interest in miRNAs as clinical biomarkers and therapeutic targets(Reference Wang, He and Mackowiak24,Reference Ji and Guo31,Reference Rupaimoole and Slack41,Reference Mori, Ludwig and Garcia-Martin42) . In the context of cancer in particular, functional and mechanistic studies have established that miRNA dysregulation can be causal, with miRNAs acting as tumour suppressors or oncogenes (oncomiRs)(Reference Goodall and Wickramasinghe30,Reference Rupaimoole and Slack41) . Although still at an early stage of development, both miRNA mimics and inhibitors of miRNAs (anti-miRs) have been investigated as therapeutics for cancer, liver and other diseases in early phase clinical trials(Reference Bonneau, Neveu and Kostantin43,Reference Bajan and Hutvagner44) . Interestingly, miR-122 binds a region of the hepatitis C virus (HCV) genome promoting its accumulation(Reference Jopling39), and Miravirsen, an anti-miR-122 oligonucleotide, was the first miRNA-targeting drug administered in humans. Although found to be safe and specific in phase 2a studies in HCV patients, its use was superseded by effective direct-acting anti-viral drugs(Reference Janssen, Reesink and Lawitz45,Reference van der Ree, van der Meer and van Nuenen46) .
A limited number of miRNA-based diagnostic assays have already been brought to market(Reference Bonneau, Neveu and Kostantin43), and considerable research has focused on profiling circulating miRNAs as biomarkers of obesity and metabolic disease, including NAFLD(Reference Gjorgjieva, Sobolewski and Dolicka23,Reference Ji and Guo31,Reference Mori, Ludwig and Garcia-Martin42) . However, the identification of miRNAs as clinical biomarkers or therapeutics is complicated by both technical and confounding factors related to miRNA biology(Reference Backes, Meese and Keller47,Reference Keller and Meese48) . While a growing body of evidence suggests miRNAs secreted in extracellular vesicles are stable in circulation and act as paracrine and endocrine factors in metabolic diseases(Reference Ji and Guo31,Reference Mori, Ludwig and Garcia-Martin42,Reference Watt, Miotto and De Nardo49) , this complicates the interpretation of miRNA expression and activity in any given cell, tissue or pathology. Age, sex and disease state all influence circulating miRNA profiles(Reference Fehlmann, Lehallier and Schaum50). Profiles vary between plasma and serum, and further variables include how long the sample was stored, whether the donor was fed or fasted and what the donor’s activity levels are(Reference Keller and Meese48,Reference Kern, Ludwig and Backes51,Reference Dufourd, Robil and Mallet52) . Importantly, expression and regulatory mechanisms observed in experimental models are not always conserved in humans(Reference Roux, Gonzàlez-Porta and Robinson-Rechavi53,Reference Schlosser, Taha and Deng54) . Historically authors have not consistently specified miRNA –5p and –3p forms or family member suffixes confounding data interpretation(Reference Paterson and Kriegel55), and quantitative polymerase chain reaction (qPCR) primer sequences are also often omitted from methods sections, potentially contributing to reproducibility issues. Given the missing detailed information of mature miRNAs in most studies, we do not use this notation throughout the text, but do detail the reported family member names and mature form suffixes from the studies reviewed and summarised in our tables.
The role of miRNAs in NAFLD pathogenesis
NAFLD pathogenesis is now recognised to involve ‘multiple hits’ and crosstalk between multiple organs and the intestinal microbiome(Reference Moore3,Reference Buzzetti, Pinzani and Tsochatzis56) . Although ‘simple’ fatty liver (NAFL) is typically benign, NASH, defined by steatosis with inflammation, hepatocyte injury (ballooning) and possibly fibrosis, more frequently develops into advanced liver disease(Reference Younossi, Koenig and Abdelatif57). In the liver at a cellular level, exacerbated by insulin resistance, lipid accumulation in the hepatocytes is driven by: increased lipid influx (from diet or adipose tissue lipolysis) and increased lipid synthesis (de novo lipogenesis), as well as impaired lipid oxidation and export. Notably, some of the miRNAs identified as altered in NAFLD play critical roles in hepatic lipid and carbohydrate metabolism and lipogenesis, regulating multiple transcription factors such as sterol regulatory element-binding protein 1 (SREBP-1), carbohydrate response element binding protein (ChREBP) and the peroxisome proliferator-activated receptors (PPARs)(Reference Gjorgjieva, Sobolewski and Dolicka23).
Progression from NAFL to NASH is a result of lipotoxicity and the over-production of reactive oxygen species (ROS), which leads to mitochondrial dysfunction, endoplasmic reticulum stress and the recruitment and signalling of immune cells. Adipokines from peripheral adipose tissue dysfunction and signalling molecules derived from the gut further drive this pro-inflammatory state systemically, as well as the resulting wound healing response and fibrosis locally in the liver(Reference Diehl and Day58). Although progression typically occurs on the order of decades, prognosis is dependent on severity of fibrosis on index biopsy(Reference Hagstrom, Nasr and Ekstedt59), and NASH significantly increases risk of HCC and liver-specific mortality(Reference Younossi, Koenig and Abdelatif57). At each stage of the progression of NAFLD, from inflammation to NASH-related HCC, miRNA-regulated pathways are also implicated, and the potential utility of miRNAs as either diagnostic biomarkers or therapeutic targets is of significant interest(Reference Gjorgjieva, Sobolewski and Dolicka23,Reference Wang, He and Mackowiak24,Reference Su, Kumar and Sud60) .
In light of the aforementioned variables that may confound the reproducibility of miRNA research, we comprehensively surveyed the literature and examined the data from serum profiling or mechanistic studies involving liver tissues to identify miRNAs with good evidence for altered levels in humans with NAFLD. Specifically, we defined ‘good evidence’ as experimentally replicated beyond array or RNAseq profiling, and identified in at least two independent studies. From a PubMed search using the terms NAFLD, NASH, MAFLD, MASH and miRNA, we found eighty-nine papers that examined miRNA levels in serum or liver in humans with NAFLD. Close data review identified seventy-four unique miRNAs (fifty-one in liver, forty-three in serum and twenty in both) validated beyond array or RNASeq profiling (Fig. 1A). Focusing on miRNAs found dysregulated in subjects with NAFLD in at least two independent studies, identified twelve miRNAs in liver and nineteen miRNAs in serum. Notably, six miRNAs (miR-21, miR-30, miR-34, miR-122, miR-146 and miR-200; Table 1) were found dysregulated in both liver and serum in at least four independent studies (Fig. 1B). An additional six miRNAs were identified as altered in livers (miR-33, miR-141, miR-155, miR-199, miR-223 and miR-378; Supplementary Table 1), and a further thirteen (miR-16, miR-20, miR-22, miR-27, miR-29, miR-99, miR-125, miR-181, miR-192, miR-197, miR-375, miR-379 and miR-451; Supplementary Table 2) were found altered in serum from participants with NAFLD in more than one study.

Fig. 1. Evidence for intersection of miRNA pathways between NAFLD and vitamin D. (A) A PubMed search using the terms NAFLD, NASH, MAFLD, MASH and miRNA found eighty-nine papers that examined miRNA levels in serum or liver in humans with NAFLD. Close data review identified seventy-four miRNAs (fifty-one in liver, forty-three in serum and twenty in both) validated beyond array or RNAseq profiling. Of the twenty miRNAs found in both liver and serum, sixteen were found in three or more studies, while four (bold) were identified in only one serum and one liver study. (B) Focusing on miRNAs found dysregulated in subjects with NAFLD in at least two independent studies led to the identification of twelve miRNAs in liver and nineteen miRNAs in serum. Six miRNAs were found dysregulated in both liver and serum in at least four independent studies, which are detailed in Table 1. (C) A PubMed search using the terms calcitriol, vitamin D and miRNA found twenty vitamin-D-associated miRNAs. Venn analysis of these twenty with the twenty-nine NAFLD-dysregulated miRNAs (the four bold from panel A plus the twenty-five in panel B) suggested seven vitamin-D-modulated miRNAs potentially relevant to NAFLD pathogenesis. Only two (bold) miRNAs were found in more than one vitamin D study.
Table 1. miRNAs dysregulated in both liver and serum from NAFLD patients

ALT, alanine aminotransferase; AST, aspartate transaminase; APTI, AST-to-platelet ratio index (fibrosis score); AUROC, the area under the receiver operating characteristic; CHB, chronic hepatitis B; CK18, cytokeratin-18; CSD, cardiac sudden death; CVD, cardiovascular disease; eLP-IR, enhanced lipo-protein insulin-resistance index; F, fibrosis stage; FIB4, fibrosis 4; GGT, gamma-glutamyl transpeptidase; HC, healthy control; HCC, hepatocellular carcinoma; HR, hazard ratio; LFTs, liver function tests; MO, morbidly obese; NAFL, non-alcoholic fatty liver; NAFLD, non-alcoholic fatty liver disease; NAS, NAFLD activity score; NASH, non-alcoholic steatohepatitis; NCSD, non-cardiac sudden death; NNL, near-normal liver; PBC, primary biliary cirrhosis; PNPLA3, patatin-like phospholipase domain containing protein 3; SAF, steatosis, activity, and fibrosis score; T2DM, type 2 diabetes mellitus; TM6SF2, transmembrane 6 superfamily member 2.
† Activity is the sum of the score of lobular inflammation and hepatocellular ballooning.
Human NAFLD studies requiring a liver biopsy are typically done in single centres and are often limited in size, similar to miRNA biomarker discovery studies more generally(Reference Keller and Meese48). For this reason, the number of participants in each study group was noted in addition to summarising the direction of expression of the miRNAs and the stages of NAFLD examined. Unsurprisingly, liver sample sizes (n ranged from three to fifty-eight per group; Table 1 and Supplementary Table 1) were smaller than the number of observations in serum (n ranged from 8 to 392 per group Table 2 and Supplementary Table 2). While serum samples typically came from NAFLD case series, liver samples were often from bariatric surgery patients or tissue banks, and in one case(Reference Braza-Boïls, Marí-Alexandre and Molina61), a post-mortem series. In spite of heterogeneous study designs, there was reasonably good concordance between studies in the (generally increased) direction of the miRNAs found dysregulated.
Table 2. Functional and pathophysiological effects of dysregulated miRNA in NAFLD

ACSL1, acyl-CoA synthetase long-chain family member 1; Col1α, collagen α1; CTGF, connective tissue growth factor; CTDNEP1, contactin-associated protein 1; EDAFN, extra domain A-fibronectin; HMGCR, 3-hydroxy-3-methylglutaryl-co-enzyme A reductase; HNF4α, hepatocyte nuclear factor 4α; NAFL, non-alcoholic fatty liver; NAFLD, non-alcoholic fatty liver disease; NASH, non-alcoholic steatohepatitis; NNL, near-normal liver; NS, not specified; PPARα, peroxisome proliferator-activated receptor α; PRKAA1, AMP-activated protein kinase; SIRT1, sirtuin 1; α-SMA, α-smooth muscle actin; SMAD7, mothers against decapentaplegic homologue 7; SREBP1, sterol regulatory element-binding protein 1; TGF-β, transforming growth factor-β; TUNEL, terminal deoxynucleotidyl transferase dUTP nick end labelling.
Given the diagnostic and therapeutic potential of miRNAs in NAFLD, we briefly outline relevant regulatory functions of the six miRNAs found altered in both liver and serum (Table 1) below. The limited data concerning functional and pathophysiological effects of their dysregulation arising from these studies, specifically in humans with NAFLD, are summarised in Table 2.
MiR-21
Originally described as an oncomiR dysregulated in multiple cancers, miR-21 is now recognised to play a role in numerous inflammatory and fibrotic diseases, including multiple liver diseases(Reference Kumarswamy, Volkmann and Thum62). In the context of NAFLD, miR-21 is up-regulated in both liver(Reference Dattaroy, Pourhoseini and Das63–Reference Rodrigues, Afonso and Simão65) and serum(Reference Rodrigues, Afonso and Simão65–Reference Liu, Pan and Zhang68), and circulating miR-21 levels have been positively correlated with serum ALT(Reference Becker, Rau and Schmitt67,Reference López-Riera, Conde and Quintas69) , steatosis, lobular inflammation(Reference Becker, Rau and Schmitt67) and hepatic activity(Reference Liu, Pan and Zhang68) (Table 1). A single study found decreased hepatic miR-21 levels, but this was in post-mortem samples from people who had died of sudden cardiac death from severe coronary artery disease(Reference Braza-Boïls, Marí-Alexandre and Molina61). The single study reporting decreased serum hepatic miR-21 levels had relatively fewer participants (twenty-five NAFLD versus twelve healthy controls (HCs)), and the diagnosis of NAFLD was not specified(Reference Sun, Huang and Liu70).
Experimental knockdown(Reference Wu, Ng and Chen71) or deletion of miR-21(Reference Calo, Ramadori and Sobolewski72) in mice markedly reduces lipogenesis and hepatic steatosis in response to high-fat feeding. Among the numerous targets of miR-21 are multiple metabolic and signalling pathways implicated in NAFLD pathogenesis(Reference Benhamouche-Trouillet and Postic73,Reference Zhang, Yang and Kusumanchi74) . These include the genes for several master transcriptional regulators involved in glucose and lipid metabolism, including the hepatocyte nuclear factor 4 alpha (HNF4α), forkhead box protein O1 (FOXO1) peroxisome proliferator-activated receptor alpha (PPARα) and sterol regulatory element-binding transcription protein 1c;(SREBP-1c)(Reference Wu, Ng and Chen71,Reference Calo, Ramadori and Sobolewski72) , as well as 3-hydroxy-3-methylglutaryl-co-enzyme A reductase (HMGCR)(Reference Sun, Huang and Liu70). In addition, miR-21 is pro-fibrotic and, as the most up-regulated miRNA during hepatic stellate cell (HSC) activation(Reference Caviglia, Yan and Jang75), serves to amplify multiple genes involved in inflammation, fibrosis and NAFLD progression(Reference Benhamouche-Trouillet and Postic73,Reference Zhang, Yang and Kusumanchi74) . While the therapeutic targeting of miR-21 is considered to have potential for multiple fibrotic diseases(Reference Liu, Ning and Ma76), somewhat surprisingly the deletion of miR-21 did not inhibit fibrosis in mouse models of toxic and biliary liver injury(Reference Caviglia, Yan and Jang75). More research is required to resolve the likely differences in miR-21 targets between humans and mice, and the complexity of miR-21 actions in the context of NAFLD, as well as other chronic liver diseases.
MiR-30
Of the miRNAs profiled in Table 1, relatively fewer studies suggest a role for miR-30 in NAFLD. One challenge in interpreting the data is that the miR-30 family has five members (miR30a–e). Beyond the aforementioned issues of inconsistent specification of family member and –5p and –3p forms in the literature, the miR-30 family members have overlapping sequences that have been found to interfere with expression profiling, and it has been suggested that only high-throughput sequencing can clearly differentiate between miR-30 family members(Reference Stokowy, Eszlinger and Świerniak77). While two studies described decreased hepatic miR-30b(Reference Latorre, Moreno-Navarrete and Mercader78,Reference Latorre, Ortega and Liñares-Pose79) expression in NAFLD, along with decreased hepatic miR-30c in one of these reports(Reference Latorre, Ortega and Liñares-Pose79), these data appear to be from the same bariatric surgery patients. Separately, two studies have reported a decrease in circulating miR-30c in NAFLD(Reference López-Riera, Conde and Quintas69,Reference Zarrinpar, Gupta and Maurya80) , with one of these reporting decreased levels and negative correlations between miR-30c and multiple measures of disease severity(Reference López-Riera, Conde and Quintas69). On the other hand, a single study has reported increased serum levels of miR-30a in a smaller number of participants (eleven NAFLD versus twelve HCs)(Reference W, ang, Wang and Yang81).
Nonetheless, there are some experimental data to suggest a role for miR-30 in NAFLD pathogenesis. As recently reviewed(Reference Ezhilarasan82), miR-30 has anti-fibrotic properties and has been found down-regulated in the context of HSC activation, hepatic fibrosis and cirrhosis in multiple models of liver injury, as well as human cirrhotic liver. Indeed, overexpression or restoration of miR-30a has been shown both in vitro and in vivo to suppress HSC activation by inhibiting epithelial–mesenchymal transition, reducing cell proliferation and migration, in line with its reported tumour suppressor activity(Reference Zheng, Wang and Yu83). Separately, limited in vitro data suggest increased miR-30a-3p expression prompts triacylglycerol accumulation in hepatocytes via targeting and decreasing PPARα expression(Reference W, ang, Wang and Yang81). Conversely, in separate experiments also in immortalised hepatocytes, fatty acid deposition triggered by both AMP-activated protein kinase (AMPK) disruption and Dicer knockdown was attenuated by overexpression of miR-30b and miR-30c associated with increased PPARα expression(Reference Latorre, Ortega and Liñares-Pose79). These limited data nevertheless suggest that a biological role for miR-30 family members cannot be ruled out.
MiR-34
In contrast, many more studies have been in complete agreement in finding increased expression of miR-34 in liver (seven studies) and increased serum levels (nine studies) in patients with NAFLD (Table 1). Higher serum miR-34 levels have been found in more advanced stages of NAFLD(Reference Yamada, Suzuki and Ichino66,Reference Liu, Pan and Zhang68,Reference López-Riera, Conde and Quintas69,Reference Cermelli, Ruggieri and Marrero84–Reference Ezaz, Trivedi and Connelly87) , and found to positively correlate with multiple markers of disease severity(Reference López-Riera, Conde and Quintas69,Reference Hendy, Rabie and El Fouly85) , including histological severity(Reference Liu, Pan and Zhang68,Reference Hendy, Rabie and El Fouly85,Reference Ezaz, Trivedi and Connelly87) and the PNPLA3 I148M and TM6SF2 E167K variants(Reference Ezaz, Trivedi and Connelly87). Moderate diagnostic accuracy has been found in multiple studies for miR-34 in discriminating NAFLD(Reference Hendy, Rabie and El Fouly85,Reference Salvoza, Klinzing and Gopez-Cervantes88) , NASH(Reference Liu, Pan and Zhang68,Reference López-Riera, Conde and Quintas69,Reference Cermelli, Ruggieri and Marrero84,Reference Hendy, Rabie and El Fouly85) and fibrosis(Reference López-Riera, Conde and Quintas69,Reference Ezaz, Trivedi and Connelly87) , with area under the receiver operating characteristics (AUROCs) ranging from 0·73 to 0·84.
Notably, a recent meta-analysis published in 2020 that examined the utility of miRNAs as non-invasive biomarkers of NAFLD concluded that miR-34 had moderate diagnostic accuracy for total NAFLD with the lowest heterogeneity (sensitivity I 2 = 5·73%, specificity I 2 = 33·16%, AUROC = 0·85) of the three miRNAs found most commonly studied (miR-34a, miR-99a, miR-122)(Reference Xin, Zhan and Chen89). An earlier meta-analysis from 2018 had concluded (from three studies at the time) miR-34a to have the best diagnostic accuracy (AUROC = 0·7783) for discriminating NASH versus NAFLD, whereas, (from three studies) miR-122, discussed in detail below, was best at discriminating NAFLD from healthy controls (AUROC = 0·8174)(Reference Liu, Ampuero and Gil-Gómez90). In addition, a recent systematic review has concluded that both miR-34a and miR-122 may be useful biomarkers in children with obesity and NAFLD(Reference Ose, s, Margareto Sanchez and Portillo91).
As direct targets of the tumour suppressor gene p53, induction of miR-34 family members is associated with apoptosis and cell cycle arrest, and inactivation of the miR-34a and miR-34b/c genes is typically observed in cancer(Reference Hermeking92). Among multiple cell cycle and apoptosis-related direct gene targets of miR-34 is sirtuin 1 (SIRT1), which, in a fascinating regulatory loop, links cholesterol, lipid and energy homoeostasis to inflammation and p53-dependent apoptosis(Reference Hermeking92,Reference Rottiers and Näär93) . An NAD-dependent deacetylase, SIRT1 directly deacetylates multiple metabolic regulators of relevance to NAFLD pathogenesis, including SREBP-1c, the PPARs (alpha and gamma), the farnesoid X receptor (FXR) and liver X receptors (LXR, alpha and beta), as well as nuclear factor-κB (NF-κB) and p53 itself(Reference Rottiers and Näär93–Reference Kosgei, Coelho and Guéant-Rodriguez95). Data from experimental models suggest possible benefit from targeting and inhibiting the miR-34/p53 pathway(Reference Castro, Ferreira and Afonso96–Reference Tian, Ji and Zang98), although some conflicting data exist around effects in HSCs(Reference Tian, Ji and Zang98,Reference Feili, Wu and Ye99) . Interestingly, resveratrol, a naturally occurring dietary polyphenol that activates SIRT1, has been tested in a number of small clinical trials for benefit in NAFLD and other metabolic diseases(Reference Kosgei, Coelho and Guéant-Rodriguez95,Reference Ding, Bao and Deng100) . A recent meta-analysis synthesising data from six randomised controlled trials (RCTs) found that, although resveratrol significantly lowered tumour necrosis factor alpha (TNF-α) and high-sensitivity C-reactive protein (hs-CRP) levels, there were no changes in numerous other cardiometabolic risk markers(Reference Rafiee, Mohammadi and Ghavami101). More potent, synthetic SIRT1 agonists are under development, and some are in early phase clinical trials(Reference Ding, Bao and Deng100).
MiR-122
In addition to its aforementioned role in HCV promotion, miR-122 is essential for lipid metabolism and has anti-inflammatory and anti-carcinogenic effects in the liver(Reference Esau, Davis and Murray102,Reference Hsu, Wang and Kota103) . The role and therapeutic targeting of miR-122 in liver disease has long been of considerable interest(Reference Jopling39,Reference Bandiera, Pfeffer and Baumert104) , and unsurprisingly, miR-122 has been the most explored miRNA in NAFLD. We identified twenty-three studies that measured miR-122 levels in serum, and eight studies that examined hepatic miR-122 expression in NAFLD (Table 1). Of the twenty-three serum studies, twenty-one found increased levels of miR-122 positively correlated with markers of NAFLD severity. Consistent with these findings, one study in a small number of patients in Japan reported decreased serum miR-122 levels with histological improvement on second biopsy(Reference Akuta, Kawamura and Suzuki105), although, other data from the same group suggest that at stage 4 fibrosis miR-122 levels decrease(Reference Akuta, Kawamura and Suzuki106) and decreased levels of miR-122 (expressed relative to the median of the cohort) may be associated with risk of mortality(Reference Akuta, Kawamura and Arase107).
Multiple studies found miR-122 to have moderate diagnostic accuracy in discriminating either NAFLD(Reference Cermelli, Ruggieri and Marrero84,Reference Salvoza, Klinzing and Gopez-Cervantes88,Reference Tan, Ge and Pan108,Reference Ye, Zhang and Lou109) , NASH(Reference Cermelli, Ruggieri and Marrero84,Reference Hendy, Rabie and El Fouly85) or histological severity(Reference Erhartova, Cahova and Dankova86,Reference Miyaaki, Ichikawa and Kamo110–Reference Auguet, Aragonès and Berlanga112) . Although a diagnostic assay for miR-122 is in pre-clinical development and has been tested in the context of drug-induced liver injury(Reference Rissin, López-Longarela and Pernagallo113), given that miR-122 is altered in multiple liver diseases, any potential utility as a biomarker for NAFLD will most likely be in combination with other miRNAs or biochemical markers(Reference Becker, Rau and Schmitt67). Notably, the limited number of currently available miRNA-based diagnostics for other diseases are panels of ten or more miRNAs(Reference Bonneau, Neveu and Kostantin43).
On the other hand, the studies of miR-122 hepatic expression in aggregate were more inconclusive. Sample sizes were typically low, and participants and/or liver samples were heterogenous in origin and variable in disease stage of NAFLD. Of the five studies reporting that miR-122 decreased in liver biopsies, three were staged as NASH(Reference Braza-Boïls, Marí-Alexandre and Molina61,Reference Castro, Ferreira and Afonso96,Reference Cheung, Puri and Eicken114) , one as NAFLD(Reference Latorre, Moreno-Navarrete and Mercader78) and one involved a small number of non-tumour HCC resected liver samples with steatosis(Reference Takaki, Saito and Takasugi115). Of the three studies reporting increased expression of hepatic miR-122 in steatosis, two found decreased expression in more advanced disease, such as NASH(Reference Pirola, Fernández Gianotti and Castaño111) and fibrosis(Reference Miyaaki, Ichikawa and Kamo110), and the third specifically excluded NASH samples, only examining steatosis in bariatric surgery patients(Reference Naderi, Pazouki and Arefian116).
The hepatic data perhaps suggest decreased expression of miR-122 in advanced disease, and the possibility of increased expression in steatosis. This fits the hypothesis of an early defensive response (in steatosis) and later causal factor in NASH progression(Reference Wang, He and Mackowiak24), and reconciles with several lines of experimental data highlighting the complexity of the dynamics of miR-122 expression and secretion in the regulation of lipid metabolism. While antisense oligonucleotide inhibition of miR-122 in vivo had beneficial effects on plasma cholesterol and hepatic steatosis in high-fat-fed (60% lard, for 19 weeks) mice(Reference Esau, Davis and Murray102), genetic deletion of miR-122 causes steatohepatitis and tumourigenesis(Reference Hsu, Wang and Kota103,Reference Tsai, Hsu and Hsu117) . In addition, NEFAs have been demonstrated to increase the expression and secretion of miR-122 inhibiting triacylglycerol synthesis in both liver and muscle(Reference Chai, Rivkin and Berkovits118). This mechanism would account for the increased serum levels of miR-122, but underscores that circulating miRNAs do not always reflect tissue expression or activity(Reference Gjorgjieva, Sobolewski and Dolicka23). The question of whether humans with NAFLD might benefit from therapeutic targeting of miR-122 through either antagomirs (anti-miRs) or miRNA mimics will require considerable more research and development and larger trials with careful staging of NAFLD.
MiR-146
Along with miR-155, miR-146 is recognised for multiple roles in the innate and adaptive immune responses and is considered an oncomiR(Reference Testa, Pelosi and Castelli119). However, knowledge regarding a role for miR-146 during NAFLD pathogenesis is limited. Three studies identified an increase in hepatic miR-146 in biopsies from participants with metabolic syndrome and NASH(Reference Cheung, Puri and Eicken114), bariatric surgery patients with NAFLD(Reference Latorre, Moreno-Navarrete and Mercader78), and steatotic tissue bank biopsies(Reference Wang, Qiu and Yang120). Two studies detecting circulating miR-146 levels were conflicting, possibly relating to clinical stage of NAFLD or ethnic differences. Whereas one European cohort comparing histologically proven NAFLD patients with healthy age-matched participants (n = 20 per group) found miR-146b decreased in NAFLD(Reference Celikbilek, Baskol and Taheri121), a separate Chinese cohort found miR-146b increased in serum from NASH patients (biopsy diagnosed, n = 31) versus healthy controls (n = 37)(Reference Liu, Pan and Zhang68).
In experimental models, decreased expression of miR-146 has been detected in dietary-induced NAFLD models(Reference Jin, Liu and Chen122,Reference He, Guo and Deng123) , and in vitro data show that miR-146 mimics can suppress lipid accumulation and inflammatory cytokines, such as tumour necrosis factor-α (TNF-α) and interleukin-6 (IL-6)(Reference He, Guo and Deng123,Reference Jiang, Liu and Dai124) . In addition, miR-146 is pro-fibrotic, and modulates fibrosis signalling pathways in HSCs(Reference Ezhilarasan82). In transforming growth factor-beta (TGF-β)-stimulated cellular models, overexpression of miR-146 inhibited the proliferation and apoptosis of HSCs and the expression of pro-fibrogenic markers(Reference He, Huang and Sun125,Reference Yuan, Chen and Wu126) . Furthermore, in a hepatic fibrosis rat model induced by CCl4, vein injection of miR-146a-expressing adenovirus increased the level of miR-146 and served to alleviate fibrogenesis(Reference Zou, Li and Li127). Collectively, the data suggest that roles of the miR-146 family in NAFLD pathogenesis should be further explored, paying attention to the different isoforms and their unique regulatory functions as previously recommended(Reference Paterson and Kriegel55).
MiR-200
Key inhibitors of the epithelial-to-mesenchymal transition, the miR-200 family (miR-200a, miR-200b, miR-200c, miR-141 and miR-429) play critical roles in both normal development and cancer metastases. High expression of miR-200 is associated with an epithelial phenotype, and recent bioinformatic analyses suggest potential binding sites for miR-200b in the 3′ untranslated regions of sixty different mRNAs involved in the epithelial-to-mesenchymal transition, the majority of which are associated with a mesenchymal phenotype that miR-200 likely inhibits(Reference Górecki and Rak128). Typically considered tumour suppressors, the miR-200 family have generally been reported down-regulated in multiple cancers, including HCC(Reference Mao, Chen and Wu129). However, this may depend on tumour stage, with data suggesting the miR-200 family are down-regulated at the primary tumour site permitting intravasation, but up-regulated in distal metastases facilitating colonisation of metastatic breast cancer cells(Reference Hilmarsdottir, Briem and Bergthorsson130).
In the context of NAFLD, to date, there are only limited data about the miR-200 family. While two studies have found miR-200a/b/c increased in steatotic and NASH liver samples from tissue banks(Reference Wang, Qiu and Yang120,Reference Tran, Lee and Shin131) , a separate study using biopsies found miR-200b/c decreased in NAFLD compared with healthy controls(Reference Guo, Fang and Sun132). An additional two studies have found miR-200 increased in serum from NAFLD patients(Reference Ezaz, Trivedi and Connelly87,Reference Wang, Zeng and Guan133) . Some evidence from animal models supports the idea that inhibition of miR-200 may attenuate steatosis, inflammation and fibrosis. For example, double deletion of miR-141 and miR-200 in mice with NASH induced from a methionine and choline deficient diet resulted in decreased steatosis and inflammation and alterations in multiple signalling pathways(Reference Tran, Lee and Shin131). In a similar vein, high-fat-fed (20% lard for 4 weeks) mice transduced with an miR-200 inhibitor also exhibited reduced steatosis and fibrosis(Reference Wang, Zeng and Guan133). However, in a separate study of high-fat-fed (45% fat for 10 weeks) mice, miR-200b and miR-200c, but not miR-200a, were found markedly decreased(Reference Guo, Fang and Sun132). These data may reflect the use of different animal models, but could also relate to disease stage. While a rationale can be made for increased expression of miR-200 early in NAFLD, and decreased expression later during disease progression conferring risk of HCC, more data at greater resolution (e.g. from single-cell experiments discriminating between hepatocytes and HSCs) are required to confirm this.
Intersection of miRNA pathways between NAFLD and vitamin D
Vitamin D is a misnomer for a family of secosteroid hormones with varying degrees of activity(Reference Demer, Hsu and Tintut134). The biological effects of the active form of vitamin D (calcitriol, 1,25-dihydroxycholecalciferol) are mediated through the interaction with the vitamin D receptor (VDR), a ligand-activated, nuclear receptor transcription factor(Reference Moore, Kato and Xie135). A significant amount of experimental research shows vitamin D has anti-proliferative, anti-inflammatory and anti-fibrotic properties that might impact disease progression in chronic liver diseases, including NAFLD(Reference Kitson and Roberts136,Reference Barchetta, Cimini and Cavallo137) . Polymorphisms in the vitamin D metabolic pathway are associated with histological severity of paediatric NAFLD(Reference Gibson, Quaglia and Dhawan20), and inadequate vitamin D status has been commonly observed in association with NAFLD severity in adults(Reference Pacifico, Osborn and Bonci17). While vitamin D has been shown to improve insulin sensitivity and glycaemic control in people with prediabetes and type 2 diabetes(Reference Mirhosseini, Vatanparast and Mazidi138,Reference Li, Liu and Zheng139) , vitamin D supplementation trials in NAFLD have been typically small (twenty to thirty people per arm(Reference Guo, Wang and Yang140)), single-centre trials and have been heterogeneous in duration, the form (e.g. vitamin D versus calcitriol), dose and mode of delivery of supplement, as well as the liver outcomes measured(Reference Zhang, Thorne and Moore22). Only a minority have measured liver biomarkers by magnetic resonance(Reference Barchetta, Del Ben and Angelico141) or biopsy(Reference El Amrousy, Abdelhai and Shawky142). Multiple questions around dosing regimes, duration of intervention and ideal stage of NAFLD to intervene remain unanswered(Reference Pacifico, Osborn and Bonci17,Reference Zhang, Thorne and Moore22) , but potential benefits for younger people with earlier stages of NAFLD have been hypothesised(Reference Barchetta, Cimini and Cavallo137), and recently demonstrated(Reference El Amrousy, Abdelhai and Shawky142).
Interestingly, miRNAs have multiple essential roles in mediating the cellular response to vitamin D, including the post-transcriptional regulation of VDR(Reference Zenata and Vrzal143). Within the 3′ untranslated region of the VDR mRNA are binding sites for four miRNAs (miR-27, miR-125, miR-298, miR-346), which have been shown to decrease VDR protein levels(Reference Pan, Gao and Yu144–Reference Chen, Du and Zhang147). Indeed, miR-125 inhibitors have been demonstrated to increase VDR expression and decrease proliferation and cell viability in vitro in HCC cells, while VDR levels were negatively correlated with miR-125 levels in tumour tissue from patients with HCC(Reference Xu, Wang and Zhang148). Moreover, multiple genes (CYP27B1, CYP24A1, RXRα) in the vitamin D pathway are regulated by miRNAs(Reference Liu, Wheelwright and Teles149–Reference Ji, Zhang and Huang151), and VDR directly regulates the transcription of multiple miRNAs(Reference Peng, Vaishnav and Murillo152). While a large body of pre-clinical research suggests the anti-cancer effects of vitamin D are mediated through miRNA regulation, data from human trials are more limited(Reference Zeljic, Supic and Magic26). Although anti-cancer effects of vitamin D have been observed in the liver(Reference Chiang, Yeh and Chen27,Reference Wu, Wang and Chen28) , potential roles for vitamin-D-regulated miRNAs in the molecular pathogenesis of NAFLD remain largely unexplored.
With the question ‘is there a potential subset of miRNAs that are both dysregulated in NAFLD and modulated by vitamin D?’ in mind, we searched PubMed broadly using the terms calcitriol, vitamin D and miRNA. As calcitriol and miRNA are both medical subheadings in PubMed, we searched a large number of related entry terms. In contrast to the number of studies profiling serum miRNAs in NAFLD, this yielded only a very limited number of human studies that had examined miRNAs in relation to either vitamin D status (Table 3; six studies) or response to vitamin D supplementation (Table 4; four studies), or had investigated miRNAs and vitamin D in the context of the liver (Table 5; seven studies). Interestingly, one manuscript identified was a study protocol for an ongoing trial that aims to measure miR-21, miR-34 and miR-122 in response to 12 weeks supplementation of 4000 IU/d vitamin D in NAFLD patients(Reference Ebrahimpour-Koujan, Sohrabpour and Foroughi153). Five studies characterized miRNAs regulated by vitamin D in a variety of diverse pathologies, including chronic hepatitis B (CHB)(Reference Mohamadkhani, Bastani and Khorrami154), primary biliary cholangitis (PBC)(Reference Kempinska-Podhorodecka, Milkiewicz and Wasik155), cirrhosis(Reference He, Ni and Zhao156) and HCC(Reference Xu, Wang and Zhang148,Reference Provvisiero, Negri and de Angelis157) . The study involving patients with CHB(Reference Mohamadkhani, Bastani and Khorrami154) measured miR-378 expression in relation to plasma 25-hydroxycholecalciferol (calcidiol, 25(OH)D) and was therefore placed in both Tables 3 and 5. A single study examined the association between miR-27b expression and its targets VDR and cytochrome P450 3A (CYP3A) in normal liver samples from a tissue bank(Reference Ekström, Skilving and Ovesjö158).
Table 3. Serum miRNA profiling studies examining vitamin D status

AML, acute myeloid leukemia; CHB, chronic hepatitis B; FAS, fatty acid synthase; GLM, generalised linear regression model; ns, not specified; NR, not reported; HBV, hepatitis B virus; 25(OH)D, 25-hydroxycholecalciferol; qPCR, quantitative polymerase chain reaction; SLE, systemic lupus erythematosus.
† 25(OH)D status defined as high: ≥79·25 nmol/l, or low: <63·75 nmol/l; ‡ The recommended adequate daily intake for vitamin D intake for vitamin D in Australia is 10 μg/d for 51–70 years old and 15 μg/d for those aged over 70 years; §Intake estimated by food frequency questionnaire 0–65·6 g/d; #Estimated from EPIC food frequency questionnaire.
Table 4. Serum miRNA profiling studies response to vitamin D supplementation

AR, allergic rhinitis; HC, healthy control; NS, not specified; NR, not reported; 25(OH)D, 25-hydroxyvitamin D; 1,25(OH)D, 1,25-dihydroxyvitamin D; PCa, prostate cancer; UM, ultra-marathon.
§ Jorde(Reference Jorde, Svartberg and Joakimsen192) used microarrays with quantitative polymerase chain reaction (qPCR) for validation; Nunez Lopez(Reference Nunez Lopez, Pittas and Pratley160) and Pastuszak-Lewandoska(Reference Pastuszak-Lewandoska, Domańska-Senderowska and Kiszałkiewicz164) used qPCR alone.
Table 5. Research studies characterising miRNA regulated by vitamin D involving liver pathology

CHB, chronic hepatitis B; CYP3A, cytochrome P450 3A; PBC, primary biliary cholangitis; PBMCs, peripheral blood mononuclear cells; PSC, primary sclerosing cholangitis; HC, healthy control; HCC, hepatocellular carcinoma; IHC, immunohistochemistry; NAFLD, non-alcoholic fatty liver disease; NL, normal liver; NR, not reported; qPCR, quantitative polymerase chain reaction; RCT, randomised clinical trial; SOCS1, suppressor of cytokine signalling 1; VDR, vitamin D receptor.
* CYP3A activity in serum measured by its endogenous marker 4β-hydroxycholesterol; CYP3A activity in liver measured by dextromethorphan N-demethylation.
From the sixteen non-redundant studies reviewed, we identified twenty miRNAs measured by qPCR. While in some cases this was secondary to microarray analysis or alongside other experimental validation (e.g. luciferase), in some instances there was no within-study experimental validation. Venn analysis of the twenty vitamin-D-associated miRNAs with the twenty-nine NAFLD-dysregulated miRNAs suggested that seven vitamin-D-modulated miRNAs (miR-27, miR-125, miR-155, miR-192, miR-223, miR-375 and miR-378; Fig. 1C) may be relevant to NAFLD pathogenesis. Notably, miR-27b directly targets and regulates VDR(Reference Zeljic, Supic and Magic26), and has been found altered in serum from NAFLD patients in three studies(Reference López-Riera, Conde and Quintas69,Reference Tan, Ge and Pan108,Reference Ando, Yamazaki and Yamada159) summarised in Table S2. Also interesting among the intersecting miRNAs was miR-192, shown to decrease in the serum of adults with prediabetes supplemented with vitamin D (2000 IU [50 μg] cholecalciferol) for 4 months in correlation with favourable changes in fasting plasma glucose levels and disposition index (the product of insulin sensitivity and insulin secretion)(Reference Nunez Lopez, Pittas and Pratley160). As eight independent studies have shown miR-192 to be up-regulated in NAFLD patients (plus one outlier showing down-regulation; summarised in Table S2), in light of the data from Nunez Lopez and colleagues(Reference Nunez Lopez, Pittas and Pratley160), it remains tempting to speculate a benefit for vitamin D supplementation in NAFLD patients. Although trials of vitamin D supplementation in adults with NAFLD have been disappointing in terms of liver endpoints, as previously discussed, sufficient questions around trial design preclude completely rejecting vitamin D as having therapeutic benefit(Reference Pacifico, Osborn and Bonci17,Reference Zhang, Thorne and Moore22,Reference Barchetta, Cimini and Cavallo137) , especially given its benefit to people with prediabetes and type 2 diabetes(Reference Mirhosseini, Vatanparast and Mazidi138,Reference Li, Liu and Zheng139) .
Out of the twenty miRNAs identified as altered by both NAFLD and vitamin D, only two, miR-125 and miR-155, were found in more than one vitamin D study (Fig. 1C). We identified three studies that reported dysregulation of miR-125 in the context of vitamin D(Reference Xu, Wang and Zhang148,Reference He, Ni and Zhao156,Reference Chen, Li and Yang161) , plus two studies that examined miR-125 in the serum of NAFLD patients (Table S2)(Reference Cai, Chen and Xu162,Reference Zhang, Yu and Cao163) . Separately, miR-155 was found in independent studies in a vitamin D context(Reference Kempinska-Podhorodecka, Milkiewicz and Wasik155,Reference Pastuszak-Lewandoska, Domańska-Senderowska and Kiszałkiewicz164) , as well as in two studies involving NAFLD liver samples (Table S1)(Reference Wang, Qiu and Yang120,Reference Wang, Zhang and Wang165) . Both miR-125 and miR-155 are expanded on briefly below.
MiR-125
The miR-125 family (miR-125a, miR-125b-1 and miR-125b-2) play essential roles in haematopoiesis and the normal function of immune cells and, perhaps unsurprisingly, have also been linked to a variety of cancers(Reference Wang, Wang and Li166). Their effects in cancer are dependent on cell type, and they have been shown to have both oncogenic and tumour suppressive activities. Along with the previously discussed miR-27, miR-125 is of note because it also targets and inhibits VDR translation(Reference Zeljic, Supic and Magic26). We identified two studies where miR-125a was examined in relation to VDR in patients with HCC(Reference Xu, Wang and Zhang148) and liver cirrhosis(Reference He, Ni and Zhao156) (Table 5). In HCC tissues (n = 31), miR-125a was found to be negatively correlated with VDR expression, and was expressed at much higher levels than in non-tumour controls (n = 11)(Reference Xu, Wang and Zhang148). Similarly, in cirrotic liver biopsies (n = 60), miR-125a expression increased with severity of liver fibrosis in association with a corresponding decrease in VDR expression(Reference He, Ni and Zhao156).
To date, only a single observational study has evaluated miR-125 in relation to vitamin D status. Chen and colleagues reported a positive correlation between miR-125 expression in T cells and serum 25(OH)D levels in patients with systemic lupus erythematosus(Reference Chen, Li and Yang161) (Table 3). Separately, two studies have examined miR-125 in serum from NAFLD patients, with conflicting results(Reference Cai, Chen and Xu162,Reference Zhang, Yu and Cao163) . Whereas Cai and colleagues found miR-125 decreased in serum from patients with ultrasound-diagnosed NAFLD (n = 34) compared with non-NAFLD (n = 20)(Reference Cai, Chen and Xu162), a separate study reported the opposite, finding miR-125 increased in NAFLD (n = 29) compared with healthy volunteers (n = 24)(Reference Zhang, Yu and Cao163). Differences in NAFLD phenotype and/or qPCR methodologies employed may explain these contradictory findings. Notably, in the latter study, the diagnostic modality for NAFLD was unsepecified and SYBR green staining was used for qPCR(Reference Zhang, Yu and Cao163). However, the associated experimental work of Cai and colleagues(Reference Cai, Chen and Xu162), in combination with previous experimental work demonstrating that miR-125 targets fatty acid synthase(Reference Zhang, Liu and Xiao167), suggests miR-125 up-regulation is likely to be protective for NAFLD and liver fibrosis, and that miR-125 in relation to NAFLD is worthy of further investigation.
MiR-155
A notorious oncomiR, increased expression of miR-155 has been found in a host of different cancers, including HCC(Reference Tang, Lei and Qi168,Reference Zhang, Wang and Li169) . Transcription of the MIR155 host gene (MIRHG155), historically termed B-cell integration cluster, is regulated by numerous transcription factors involved in the inflammatory response, including nuclear factor-kappa B (NF-kB), interferon regulatory factors, TGF-β and hypoxia inducible factor 1 alpha, among others(Reference Mahesh and Biswas170). Therefore, the aberrant expression of miR-155 plays a vital role in multiple inflammatory molecule and signalling pathways. Critical to both innate and adaptive immune responses, miR-155 influences the immune inflammatory response in part through directly targeting suppressors of cytokine signalling 1 (SOCS1)(Reference Yao, Ma and Liang171).
Interestingly, miR-155 is inhibited by VDR, which directly interacts with IκB kinase (IKKβ), preventing nuclear factor kB (NFkB) activation and transrepression of MIRHG155 (Reference Chen, Zhang and Ge172). Calcitriol decreases miR-155 expression in human macrophages(Reference Chen, Liu and Sun173,Reference Arboleda, Fernandez and Urcuqui-Inchima174) and adipocytes(Reference Karkeni, Bonnet and Marcotorchino175). In mice, vitamin D supplementation ameliorated the increase in miR-155 in adipose tissue in response to high-fat feeding, in further support of an anti-inflammatory role of vitamin D in obesity(Reference Karkeni, Bonnet and Marcotorchino175). Moreover, miR-155 has been observed to decrease in response to both dietary weight loss and bariatric surgery, and has been proposed as a biomarker of weight loss(Reference Langi, Szczerbinski and Kretowski176,Reference Catanzaro, Filardi and Sabato177) . In the context of NAFLD, hepatic miR-155 expression was shown to be increased alongside miR-34a and miR-200a-c and other miRNAs in a small number (n = 4 per group) of tissue bank biopsies from patients with and without steatosis(Reference Wang, Qiu and Yang120). Hepatic expression of miR-155 has also been found elevated in cholestatic liver disease, and was related to decreased levels of VDR and SOCS1 protein in the peripheral blood mononuclear cells of patients(Reference Kempinska-Podhorodecka, Milkiewicz and Wasik155). The authors point out that the decreased VDR expression was observed in spite of patients being supplemented with vitamin D and having normal vitamin D status.
Perhaps counterintuitively, in 2016, Wang and colleagues(Reference Wang, Zhang and Wang165) reported significantly decreased circulating levels of miR-155 in fifty participants with NAFLD compared with fifty healthy controls, as well as decreased hepatic miR-155 levels in eleven biopsy samples from NAFLD patients compared with eleven control biopsies. However, in accompanying experimental work, they showed miR-155 directly targets LXRα, which targets SREBP-1c and fatty acid synthase (FAS) influencing lipid accumulation. In addition, high-fat-fed mice transfected with miR-155 mimics had significantly reduced hepatic steatosis, as well as decreased expression of LXRα, SREBP-1c and FAS(Reference Wang, Zhang and Wang165). Apart from the aforementioned study in cholestatic liver disease, we identified only one other study examining miR-155 response to vitamin D supplementation. Unusually, it involved very-high-dose vitamin D supplementation (10 000 IU/250 μg cholecalciferol) for 2 weeks prior to a 100 km ultra-marathon. In this small study done in a unique population, miR-155 levels increased in both groups after the ultra-marathon, but there was no difference between groups(Reference Pastuszak-Lewandoska, Domańska-Senderowska and Kiszałkiewicz164).
Genome-wide analyses have demonstrated miR-155 has many hundreds of gene targets, and furthermore miR-155 binding and miR-155-dependent repression are regulated in a cell-context-dependent fashion(Reference Nam, Rissland and Koppstein178,Reference Hsin, Lu and Loeb179) , which may explain these somewhat disparate results. However, the pre-clinical data and data from weight loss intervention studies suggest that the potential interactions between miR-155, vitamin D, and hepatic lipid metabolism and inflammation in the molecular pathogenesis of NAFLD, are worth pursuing.
Conclusions
This review critically assessed the evidence for a potential subset of miRNAs that are both dysregulated in NAFLD and modulated by vitamin D. Comprehensive review of the literature found numerous studies examining dysregulation of miRNA levels in humans with NAFLD. We identify twenty-nine miRNAs found dysregulated in more than one NAFLD study, including six (miR-21, miR-30, miR-34, miR-122, miR-146 and miR-200) found dysregulated in multiple independent NAFLD studies. On the other hand, a paucity of human studies were identified that had investigated miRNAs in relation to vitamin D status, response to supplementation, or vitamin D in the context of the liver. This is a notable gap in the evidence base, given that VDR mediates its cellular response in part by directly targeting miRNAs that regulate transcription factors involved in NAFLD pathogenesis, and considering that VDR expression is directly regulated by miRNAs likely disrupted in NAFLD.
Our critical review found evidence from human studies for seven vitamin-D-modulated miRNAs (miR-27, miR-125, miR-155, miR-192, miR-223, miR-375 and miR-378) potentially relevant to NAFLD pathogenesis (overall summary in Fig. 2). While we await the results of the ongoing trial of Ebrahimpour-Koujan and colleagues(Reference Ebrahimpour-Koujan, Sohrabpour and Foroughi153) with interest, we believe that the measurement of serum and hepatic miRNAs in response to vitamin D supplementation in larger trials or biobanked samples is warranted. While miRNA analyses of liver tissue are unlikely to add diagnostic value to already informative, but invasive, liver biopsies, they may be key to further understanding pathobiology. On the other hand, the measurement of serum miRNAs is non-invasive. Given that current genetic risk factors for NAFLD are non-specific and predict severity of multiple liver diseases, a fascinating, unanswered question worthy of deliberate inquiry is whether serum miRNA signatures might yield diagnostic specificity for either chronic liver disease stage or aetiology. Although individual miRNAs alone seem unlikely to provide such specificity, for the earlier stages of NAFLD in particular, panels of diet-responsive miRNAs may be particularly intriguing. The summary tables within this review provide a significant resource to underpin future hypothesis-driven research to tackle such questions, including gene expression meta-analysis studies. We conclude that the modulation of miRNAs by vitamin D has been understudied and that, based on of the evidence to date, a therapeutic benefit for vitamin D supplementation in NAFLD can not be ruled out.
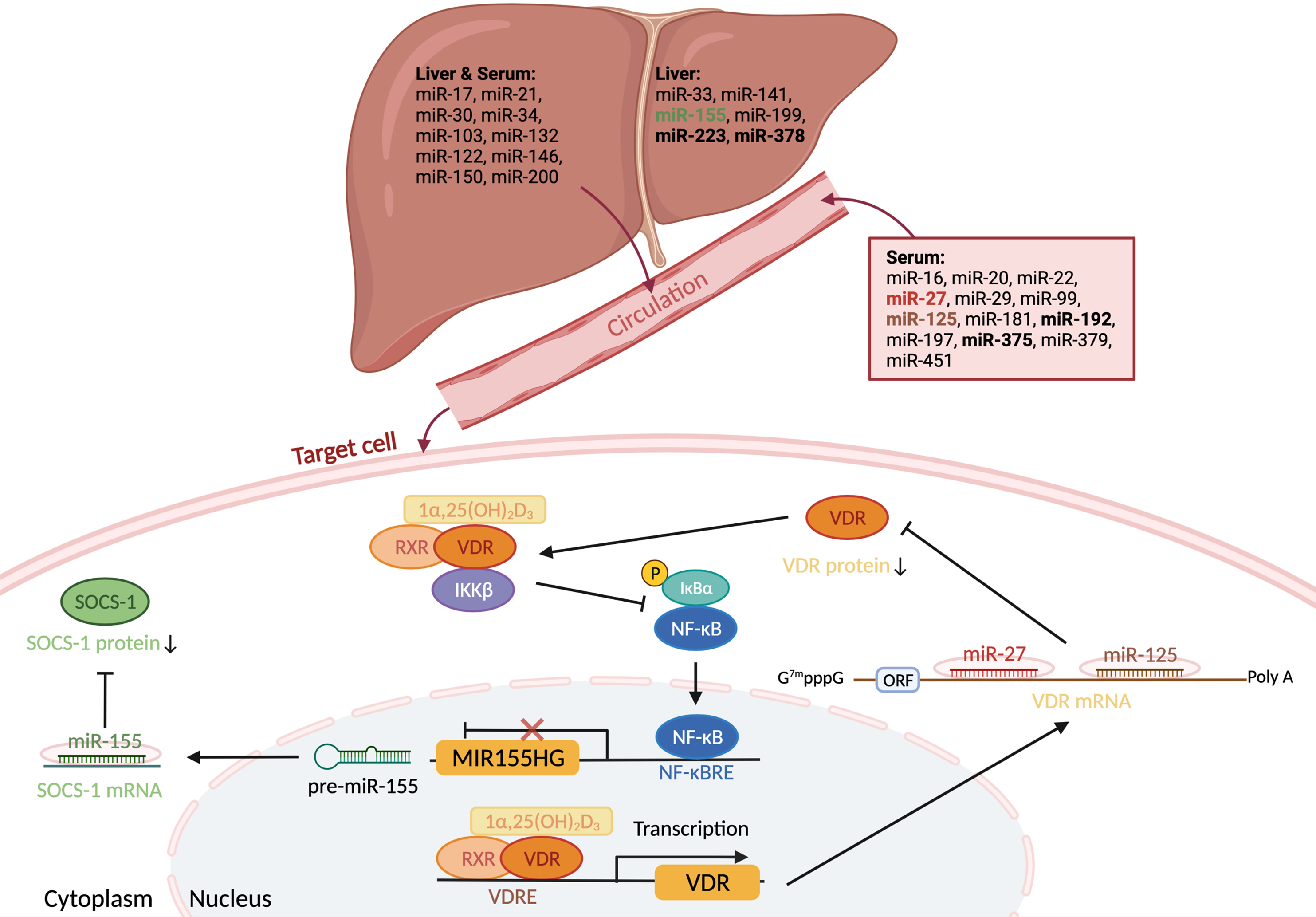
Fig. 2. Overview of miRNAs altered by NAFLD and vitamin D. Twenty-nine miRNAs were identified dysregulated in NAFLD in more than one study. Seven (bold) were also found in separate studies as vitamin D modulated. Two of these miRNAs, miR-27 and miR-125, target vitamin D receptor (VDR) mRNA and decrease translation. The transcription of a third miRNA, miR-155, is inhibited by VDR, which directly interacts with IκB kinase (IKKβ), preventing nuclear factor kB (NFkB) activation and transrepression of the MIR155 host gene. Relevant to NAFLD, in the context of low vitamin D/VDR signalling, miR-155 lowers expression of the suppressor of cytokine signalling 1 (SOCS-1), increasing expression of pro-inflammatory cytokines.
Author contributions
Z.Z., R.M., J.L.T. and J.B.M contributed to review concept and design. Z.Z and J.B.M extracted data. R.M. and Z.Z. contributed to manuscript drafts. J.B.M. wrote the final manuscript. All authors critically reviewed the manuscript for intellectual content and approved the final version of the manuscript. Fig. 2 was created with BioRender.com.
Financial support
This research received no specific grant from any funding agency, commercial or not-for-profit sectors.
Conflicts of interest
All authors declare no conflicts of interest.
Supplementary material
To view supplementary material for this article, please visit https://doi.org/10.1017/S095442242100038X.