Introduction
Small mammals including rodents play important roles in shaping ecological structure and species composition and diversity of plants within ecosystems (Nyirenda et al., Reference Nyirenda, Namukonde, Simwanda, Phiri, Murayama, Ranagalage and Milimo2020). They are often referred to as bio-engineers (Cameron, Reference Cameron, Lacey, Patton and Cameron2000; Reichman, Reference Reichman, Begal, Burda and Schleich2007) because they contribute to the chemical and physical properties of soil (Galiano et al., Reference Galiano, Kubiak, Overbeck and de Freitas2014; Yong et al., Reference Yong, Jalaludin, Brau, Shamsudin and Heo2019) and facilitate seed dispersal (Midgley and Anderson, Reference Midgley, Anderson, Forget, Lambert, Hulme and Vander Wall2005; Flores-Peredo et al., Reference Flores-Peredo, Sánchez-Velásquez, Galindo-González and Morales-Mávil2011) and pollination (Wiens et al., Reference Wiens, Rourke, Casper, Rickart, LaPine, Paterson and Channing1983; Wester et al., Reference Wester, Stanway and Pauw2009). Small mammals also form an integral part of food webs by acting as a food source for predators as well as consumers of plant material and arthropods (Morand et al., Reference Morand, Krasnov, Poulin, Degen, Morand, Krasnov and Poulin2006). In addition, rodents are known hosts for a diverse range of ectoparasite taxa (e.g. fleas, lice, ticks and mites) (Morand et al., Reference Morand, Krasnov, Poulin, Degen, Morand, Krasnov and Poulin2006). Life history traits (e.g. group size, nesting behaviour and habitat use) of a rodent species often influences their exposure to parasites and therefore their parasite profiles (Vaumourin et al., Reference Vaumourin, Vourc'h, Gasqui and Vayssier-Taussat2015). This is because ectoparasites vary in their level of host specificity, micro-habitat preference and mode of transmission (Hopla et al., Reference Hopla, Durden and Keirans1994; Paramasvaran et al., Reference Paramasvaran, Sani, Hassan, Krishnasamy, Jeffery, Oothuman, Salleh, Lim, Sumarni and Santhana2009). For example, lice are host-specific permanent parasites with all life stages occurring on the host's body and are transmitted through direct body contact between hosts, while ticks generally have a broader host range and attaches to a host only once during a life stage (larval, nymphal or adult) to obtain a bloodmeal (Morand et al., Reference Morand, Krasnov, Poulin, Degen, Morand, Krasnov and Poulin2006). Several ectoparasite taxa are known vectors for disease-causing pathogens (e.g. Yersinia pestis for plague and Rickettsia species for various rickettsioses). Consequently, it is important to develop accurate parasite profiles for rodents that routinely move between natural (reserves) and anthropogenic (e.g. agriculture and village) habitats to prevent spillover of pathogens into human-associated habitats.
The occurrence of parasites and infestation within a host population are influenced by both host-associated and environment-associated factors (Krasnov and Matthee, Reference Krasnov and Matthee2010; Stanko et al., Reference Stanko, Fričová, Miklisová, Khokhlova and Krasnov2015; Obiegala et al., Reference Obiegala, Arnold, Pfeffer, Kiefer, Kiefer, Sauter-Louis and Silaghi2021). Host-associated factors include body size, age, sex and reproductive state (Morand and Poulin, Reference Morand and Poulin1998; Kołodziej-Sobocińska, Reference Kołodziej-Sobocińska2019). For example, larger hosts can harbour more parasites due to larger total mass (more potential resources for parasites) and larger surface area (more space/niches for parasites) (Lindenfors et al., Reference Lindenfors, Nunn, Jones, Cunningham, Sechrest and Gittleman2007; Froeschke et al., Reference Froeschke, van der Mescht, McGeoch and Matthee2013). Body size is also indicative of host age and older hosts may accumulate parasites over time (Moore and Wilson, Reference Moore and Wilson2002; Poulin, Reference Poulin2007). Host sex can also influence parasite infestations, which are often related to sexual size dimorphism characteristic for many host species (Moore and Wilson, Reference Moore and Wilson2002) and difference in home range sizes between male and female hosts (Krasnov et al., Reference Krasnov, Morand, Hawlena, Khokhlova and Shenbrot2005). In addition, elevated hormone levels can facilitate host sex-associated differences in mammals during the breeding season (Lightfoot, Reference Lightfoot2008). Reproductively active males that experience elevated testosterone levels, may become more aggressive towards conspecifics (Zielinski and Vandenbergh, Reference Zielinski and Vandenbergh1993; Simon and Lu, Reference Simon, Lu and Nelson2006; Gleason et al., Reference Gleason, Fuxjager, Oyegbile and Marler2009) and enlarge their home range size in search of females (Tew and Macdonald, Reference Tew and Macdonald1994; Bergallo and Magnusson, Reference Bergallo and Magnusson2004). Testosterone has immunosuppressive properties that can increase male susceptibility to parasites (Hughes and Randolph, Reference Hughes and Randolph2001; Klein, Reference Klein2004; Matthee et al., Reference Matthee, McGeoch and Krasnov2010), whereas lowered immune defences during gestation can render female mammals more susceptible to parasites (Christe et al., Reference Christe, Arlettaz and Vogel2000; Viljoen et al., Reference Viljoen, Bennett, Ueckermann and Lutermann2011). Larger home ranges increase contacts between male hosts increasing probability of encounter with ticks and chiggers (Scantlebury et al., Reference Scantlebury, Maher McWilliams, Marks, Dick, Edgar and Lutermann2010; Butler et al., Reference Butler, Trout Fryxell, Houston, Bowers, Paulsen, Coons and Kennedy2020). In contrast, during the breeding season, reproductively active female hosts are more tolerant of conspecifics and engage in social grooming between group members (Meaney and Stewart, Reference Meaney and Stewart1979; Ganem and Bennett, Reference Ganem and Bennett2004). A higher contact rate between host individuals can facilitate parasite exchange (Bordes et al., Reference Bordes, Blumstein and Morand2007; Patterson and Ruckstuhl, Reference Patterson and Ruckstuhl2013), though host-induced mortality of parasites due to grooming can benefit female hosts (Marshall, Reference Marshall1981; Krasnov et al., Reference Krasnov, Khokhlova and Shenbrot2002). In many rodent species, females may have a stronger nest association during the breeding season (Choate, Reference Choate1972; Zenuto et al., Reference Zenuto, Vassallo and Busch2001), which can promote infestations by nidicolous parasites such as fleas and mites (Krasnov et al., Reference Krasnov, Matthee, Lareschi, Korallo-Vinarskaya and Vinarski2010). The effect of environment ( = habitat)-associated factors are first and foremost determined by high sensitivity of ectoparasites to air temperature and relative humidity in terms of, for example, development rate and survival (Krasnov et al., Reference Krasnov, Khokhlova, Fielden and Burdelova2001; Herrmann and Gern, Reference Herrmann and Gern2010; van der Mescht et al., Reference Van der Mescht, le Roux and Matthee2013). This is particularly true for taxa with free-living life stages (fleas, mites and ticks). The vegetation structure (plant growth forms and vegetation cover) in a habitat can influence the microclimatic conditions by reducing the soil temperature and loss of soil moisture (He et al., Reference He, D'Odorico, De Wekker, Fuentes and Litvak2010; Jucker et al., Reference Jucker, Hardwick, Both, Elias, Ewers, Milodowski, Swinfield and Coomes2018; Lozano-Parra et al., Reference Lozano-Parra, Pulido, Lozano-Fondón and Schnabel2018). Consequently, variation in the microclimatic conditions between habitat types (e.g. natural and transformed habitat types) can affect parasite occurrence and infestation levels (Lorch et al., Reference Lorch, Fisher and Spratt2007; Froeschke et al., Reference Froeschke, van der Mescht, McGeoch and Matthee2013; Froeschke and Matthee, Reference Froeschke and Matthee2014; van der Mescht et al., Reference Van der Mescht, Le Roux, Matthee, Raath and Matthee2016).
South Africa has a rich diversity of small mammals and, in particular, rodents (Skinner and Chimimba, Reference Skinner and Chimimba2005). Among the approximately 50 rodent species recorded in South Africa, many vary in geographic range and adaptability to habitat transformation (Skinner and Chimimba, Reference Skinner and Chimimba2005; Monadjem et al., Reference Monadjem, Taylor, Denys and Cotterill2015). Currently, most information on rodent parasites is limited to host-parasite lists in monographs of which some are outdated (Zumpt, Reference Zumpt1961; Theiler, Reference Theiler1962; Ledger, Reference Ledger1980; Segerman, Reference Segerman1995; Horak et al., Reference Horak, Heyne, Williams, Gallivan, Spickett, Bezuidenhout and Estrada-Peña2018). More recently, empirical studies based on large sample sizes have been conducted on a few rodent species (Matthee et al., Reference Matthee, Horak, Beaucournu, Durden, Ueckermann and Mcgeoch M2007, Reference Matthee, McGeoch and Krasnov2010; Fagir et al., Reference Fagir, Ueckermann, Horak, Bennett and Lutermann2014, Reference Fagir, Bennett, Ueckermann, Howard and Hart2021; Stevens et al., Reference Stevens, Stekolnikov, Ueckermann, Horak and Matthee2022). These studies highlighted the potentially large ectoparasite diversity in locally abundant and regionally widespread species such as the four-striped mouse (Rhabdomys pumilio) (Matthee et al., Reference Matthee, Horak, Beaucournu, Durden, Ueckermann and Mcgeoch M2007, Reference Matthee, McGeoch and Krasnov2010; Matthee and Krasnov, Reference Matthee and Krasnov2009), Namaqua rock mouse (Micaelamys namaquensis) (Fagir et al., Reference Fagir, Ueckermann, Horak, Bennett and Lutermann2014) and mole rats (Viljoen et al., Reference Viljoen, Bennett, Ueckermann and Lutermann2011; Fagir et al., Reference Fagir, Bennett, Ueckermann, Howard and Hart2021) that readily adapt to agricultural habitats. In addition, the occurrence of undescribed ectoparasite species and new parasite-host and parasite-locality records in these studies suggested that the ectoparasite diversity in South African rodents is currently underestimated (Matthee et al., Reference Matthee, Horak, Beaucournu, Durden, Ueckermann and Mcgeoch M2007; Matthee and Ueckermann, Reference Matthee and Ueckermann2008, Reference Matthee and Ueckermann2009; Fagir et al., Reference Fagir, Ueckermann, Horak, Bennett and Lutermann2014; Stevens et al., Reference Stevens, Stekolnikov, Ueckermann, Horak and Matthee2022). Moreover, ecological studies on factors that influence ectoparasite infestations and their species composition are sparse. In other words, ectoparasite communities of South African rodents and factors influencing structure of these communities remain to be further investigated.
The Bushveld gerbil (Gerbilliscus leucogaster) is a widespread, nocturnal rodent occurring mainly in the Grassland and Savanna biomes of southern Africa (Skinner and Chimimba, Reference Skinner and Chimimba2005; Odhiambo et al., Reference Odhiambo, Makundi, Leirs and Verhagen2008). These gerbils are also commonly found in agricultural areas where they are seen as pests of crops (Odhiambo et al., Reference Odhiambo, Makundi, Leirs and Verhagen2008; Von Maltitz et al., Reference Von Maltitz, Kirsten and Labuschagne2016). The species is medium in size (48–98 g) with no clear sexual dimorphism (Skinner and Chimimba, Reference Skinner and Chimimba2005; Lötter, Reference Lötter2010). It constructs burrows in sandy soils that are cleaned every night (Apps, Reference Apps2012; Monadjem et al., Reference Monadjem, Taylor, Denys and Cotterill2015) and demonstrates communal living (De Graaff, Reference De Graaff1981; Skinner and Chimimba, Reference Skinner and Chimimba2005), with family groups sharing burrows (Monadjem et al., Reference Monadjem, Taylor, Denys and Cotterill2015) and reproduce during spring-summer (Perrin and Swanepoel, Reference Perrin and Swanepoel1987; Neal, Reference Neal1991). Although the biology of this rodent is relatively well studied, limited data exist on the ectoparasite diversity associated with G. leucogaster. Current data for this species is, as mentioned above, restricted to historic monographs (Zumpt, Reference Zumpt1961; Ledger, Reference Ledger1980; De Graaff, Reference De Graaff1981; Segerman, Reference Segerman1995; Horak et al., Reference Horak, Heyne, Williams, Gallivan, Spickett, Bezuidenhout and Estrada-Peña2018) and a single field study at a single locality in the Savanna biome (Braack et al., Reference Braack, Horak, Jordaan, Segerman and Louw1996). The latter study identified a number of louse, flea and tick species on G. leucogaster, but mite species identification was incomplete.
Here, we studied ectoparasite diversity and factors that drive their infestation on G. leucogaster. Our objectives were (a) to record ectoparasite (especially mite due to incomplete knowledge) species and their level of infestation (mean abundance and prevalence) and (b) to establish the relationship between host-related (sex, body size and reproductive state) and habitat-related (natural vs agricultural) factors on ectoparasite infracommunity structure, namely (a) diversity in terms of species richness and (b) abundance in terms of the number of ectoparasite individuals. Given the nest type, social behaviour and habitat use of G. leucogaster, we expected high ectoparasite, especially mite, diversity and abundance.
Materials and methods
Study area
The study is part of a larger on-going research programme conducted within the Mnisi OneHealth platform in Mpumalanga Province, South Africa (Berrian et al., Reference Berrian, van Rooyen, Martínez-López, Knobel, Simpson, Wilkes and Conrad2016) (Fig. 1). The Mnisi community comprises several villages that are bordered by large, fenced nature reserves. Rodents were trapped in 4 villages and 4 crop fields within these villages' (Gottenburg 24°38′01″ S, 31°25′19″ E; Thlavekisa 24°37′51″ S, 31°22′42″ E, Athol 24°42′29″ S, 31°20′43″ E and Utah 24°50′14″ S, 31°02′45″ E). In general, subsistence farming is practiced within these settlements. Small vegetable patches and cattle occur on the property (village), while small crop fields (agricultural areas) often occur between the village and the nature reserve. Rodents were also trapped at 4 localities in the Manyeleti Nature Reserve (24°38′52″ S, 31°31′35″ E) that represents pristine natural Savanna vegetation.

Figure 1. Locality map of the study area for Gerbilliscus leucogaster (n = 127) within the Mnisi OneHealth platform in the Mpumalanga Province, South Africa. The village sites are represented by black triangles (n = 4) and the shaded area is Manyeleti nature reserve.
Rodent trapping
Gerbilliscus leucogaster individuals were trapped at 3 villages and their respective crops in (August–October) of 2014 and 2015 and once in summer (January) of 2015 and at 4 villages and their respective crops in spring (August–October) 2019 and 2020 (Fig. 1). All localities were >1 km apart. In each habitat type, a standardized rodent trap design was used. Sherman-type live traps (80) were set in trap lines baited with a mixture of peanut butter and oats and set for 3–4 days per locality. Each locality was only trapped once during each trap period. During this time, traps were checked twice a day and closed in the heat of the day (10:00–15:00). Targeted rodents were removed from traps, placed in pre-marked plastic bags and euthanized with Isoflurane. Once labelled, the carcasses were frozen at −20°C (to preserve the integrity of the material and to kill the ectoparasites) for later examination. The study was approved by the Animal Ethics Committees of Stellenbosch University (Reference numbers: ACU 2016-00190; ACU 2018-4555; ACU 2020-17062), the Mpumalanga Tourism and Parks Agency (Permit number ES 5/14; MPB. 5694; MPB. 5663), Department of Agriculture, Forestry and Fisheries (Reference number 12/11/1/7/5) and Pretoria University (Reference number V046-14; VO23-19).
Laboratory procedures
Prior to ectoparasite removal, the carcasses were thawed. Each rodent body was systematically (anteriorly and posteriorly) examined for ectoparasites using a Zeiss Stemi DV4 stereomicroscope (Carl Zeiss Light Microscopy, Göttingen, Germany) and fine point forceps (used to separate hair shafts and to remove the ectoparasites). All rodents were preliminarily identified based on morphological and dental morphology using taxonomic references (De Graaff, Reference De Graaff1981; Perrin and Swanepoel, Reference Perrin and Swanepoel1987; Skinner and Chimimba, Reference Skinner and Chimimba2005; Monadjem et al., Reference Monadjem, Taylor, Denys and Cotterill2015) and thereafter confirmed molecularly (cytochrome b gene) (Bastos et al., Reference Bastos, Nair, Taylor, Brettschneider, Kirsten, Mostert, von Maltitz, Lamb, van Hooft, Belmain, Contrafatto, Downs and Chimimba2011). For each rodent individual, we recorded sex and body measurements (weight, total body length, tail length and hind foot length). Thereafter reproductive state (reproductively active females were characterized by a perforated vagina and reproductively active males were characterized by enlarged testes) was recorded. All fleas, lice, ticks and mesostigmatan mites (hereafter referred to as mites) were removed and transferred to sample tubes filled with 100% ethanol. All fleas were counted (male and female) but only male individuals were available for identification and used for the species abundance (this is because female fleas were used in a separate project). For each louse species counts of immature stages were combined and reported as nymphs. In the case of chiggers, only a sub-sample was collected and the parasitope (region on the body where chiggers occurred) recorded. Fleas (males), lice, mites and chiggers were cleared, and slide mounted (fleas in Canada balsam and the rest in Hoyer's mounting medium) using standard techniques, while ticks remained unmounted. Ectoparasite identification was conducted using taxonomic reference keys. Fleas were identified according to Segerman (Reference Segerman1995) and lice were identified using various reference sources (Ledger, Reference Ledger1980; Durden and Musser, Reference Durden and Musser1994). Ticks were identified according to Walker et al. (Reference Walker, Keirans and Horak2000) and Horak et al. (Reference Horak, Heyne, Williams, Gallivan, Spickett, Bezuidenhout and Estrada-Peña2018). Mites were identified using various reference sources (Till, Reference Till1963; Herrin and Tipton, Reference Herrin and Tipton1975; Matthee and Ueckermann, Reference Matthee and Ueckermann2008), but species identification was not possible for all mites. Chiggers were identified following Zumpt (Reference Zumpt1961) and Stekolnikov and Matthee (Reference Stekolnikov and Matthee2019). Identification of lice, fleas and chiggers was done using a Leica DM1000 light microscope (Leica Microsystems GmbH, Wetzlar, Germany) and that of ticks using a Leica MZ75 high-performance stereomicroscope (Leica Microsystems GmbH, Wetzlar, Germany).
Data analysis
Rodent and ectoparasite data were pooled per locality within each of the habitat types (natural, agriculture and village) within a sampling year. We divided the ectoparasites into higher taxonomic groups (fleas, lice, mites and ticks) and pooled the different life stages (i.e. larvae, nymphs, males and females) within the respective ectoparasite taxa. For each higher ectoparasite taxon, we calculated mean abundance (mean number of parasites on an individual host) and prevalence (% of hosts infested). In addition, we considered total counts of ectoparasites of a given higher taxon and their species richness (the number of species) on an individual host (i.e. infracommunity). Analysis of species richness was not carried out for fleas and lice because these taxa were dominated by 1 and 2 species, respectively, even though more than one species were recorded. Because (a) the data were collected in different years and (b) some dependent variables (the number of ectoparasite individuals and ectoparasite species richness) were not normally distributed (Shapiro–Wilk tests), we applied generalized linear mixed-effect models with the lme4 package (Bates et al., Reference Bates, Maechler, Bolker and Walker2015) in R (R Core Team, 2020) using year of sampling as a random factor and a negative-binomial error distribution. Thereafter, we applied model selection and model averaging using the R package MuMIn (Bartoń, Reference Bartoń2018) to identify host-associated (sex, body size using tail length as a proxy and reproductive state) and habitat-associated (habitat type) variables and the interactions between them affecting the numbers of ectoparasite individuals and species. For each model averaging scenario, 95% confidence interval values are reported, and explanatory variables were considered significant when confidence intervals did not include zero. We also calculated conditional R2 (the proportion of the variance explained by both fixed and random effects) and marginal R2 (the proportion of the total variance explained by the fixed effects) of Nakagawa et al. (Reference Nakagawa, Johnson and Schielzeth2017) for all models.
Results
A total of 127 G. leucogaster (77 males and 50 females) were captured and examined for ectoparasites (Table 1). The average tail length was 13.98 ± 0.20 cm in males and 14.02 ± 0.26 cm in females. Data on reproductive state were available for 123 rodents of which 74 were reproductively active (61 males and 13 females). We identified 21 genera that comprised of 28 ectoparasitic and 4 non-parasitic species (predatory mites: mites that predate on invertebrates in the nest of the host) (Table 2). Mites were represented by 14 species, followed by 9 chigger species, 5 tick species, 3 flea species and 2 louse species (Table 2). In total, 6758 epifaunistic individuals (excluding chiggers) were recorded of which mites and lice were the most abundant. Fleas were the most prevalent followed by mites (Table 3).
Table 1. Sampling period and sample size for Gerbilliscus leucogaster (n = 127) trapped in Mpumalanga, South Africa (2014–2020)

Table 2. Epifaunistic arthropod taxa recorded on Gerbilliscus leucogaster (n = 127) in Mpumalanga, South Africa, 2014–2020

Family name indicated in bold. Taxonomic authority included.
PPredatory feeding strategy.
Table 3. Epifaunistic arthropod taxa and their infestation parameters recorded from Gerbilliscus leucogaster (n = 127) in Mpumalanga Province, South Africa, 2014–2020

Number/proportions for ectoparasite groups are indicated in bold.
a Taxon count includes all male and female individuals.
b Count for flea species based on male individuals only, lice nymphs: instars I, II and III combined.
c Mite nymphs represents proto- and deutronymphs.
P Predatory feeding strategy.
Epifaunistic diversity
Three flea species from the genus Xenopsylla were recovered from G. leucogaster (Table 3). Based on adult male fleas, Xenopsylla frayi was the most abundant and prevalent (>70%) flea species. The 2 remaining species (Xenopsylla brasiliensis and Xenopsylla bechuanae) occurred on <5% of the rodents (Table 3). Among lice, Polyplax biseriata was the most prevalent (74.80%) compared to Hoplopleura biseriata (2.67%) (Table 3). Five ixodid tick species, from 4 genera, were recorded (Table 3). Hyalomma truncatum was the most abundant and prevalent, followed by Dermacentor rhinocerinus. Ticks were represented by nymph and larval life stages (Table 3). Fourteen mite species (excluding Trombiculidae) were found. Androlaelaps oliffi was the most abundant and prevalent species, followed by Androlaelaps marshalli. Androlaelaps mites represented 90% of the 14 mite species. Three unknown mite species were recorded (1 in the genus Pachylaelaps and 1 each from families Acaroidae and Uropodidae). Cheyletus zumpti was the most prevalent (22.05%) predatory mite. The adult female life stage was the most common life stage for 6 of the Laelapidae species (Table 3). Nine chigger species were recorded with an overall prevalence of 35.43% (Table 3). Schoutedenichia lumsdeni was the most prevalent species followed by Gahrliepia nana. Chiggers occurred on various parasitopes of which the pinna (external part of ear) was the most preferred followed by the front leg (Table 4).
Table 4. Prevalence and parasitope for chigger species (Trombiculidae) recorded from Gerbilliscus leucogaster (n = 127) in Mpumalanga Province, South Africa, 2014–2020.

Effects of host- and habitat-associated factors
The most abundant species in 4 of the higher taxa (excluding chiggers) were more abundant and prevalent on male compared to female rodents (Supplementary Table 1). The results of model selection and averaging are presented in Tables 5 and 6. None of the infestation parameters for any of the ectoparasite taxa was significantly associated with host body size. The number of flea and mite individuals were significantly related to host sex and the interaction between host sex and reproductive state (Table 5), with male hosts harbouring more flea and mite individuals than female hosts (Fig. 2A and 2B). Furthermore, the effect of the interaction of host sex and reproductive state on flea and mite numbers in infracommunities was manifested by higher parasite counts on reproductively active males followed by that on non-breeding females (Fig. 2A and 2B; Table 5). In addition, mite species richness was also significantly related to host sex, with a larger number of mite species occurring on males compared to females (Fig. 2C; Table 6).
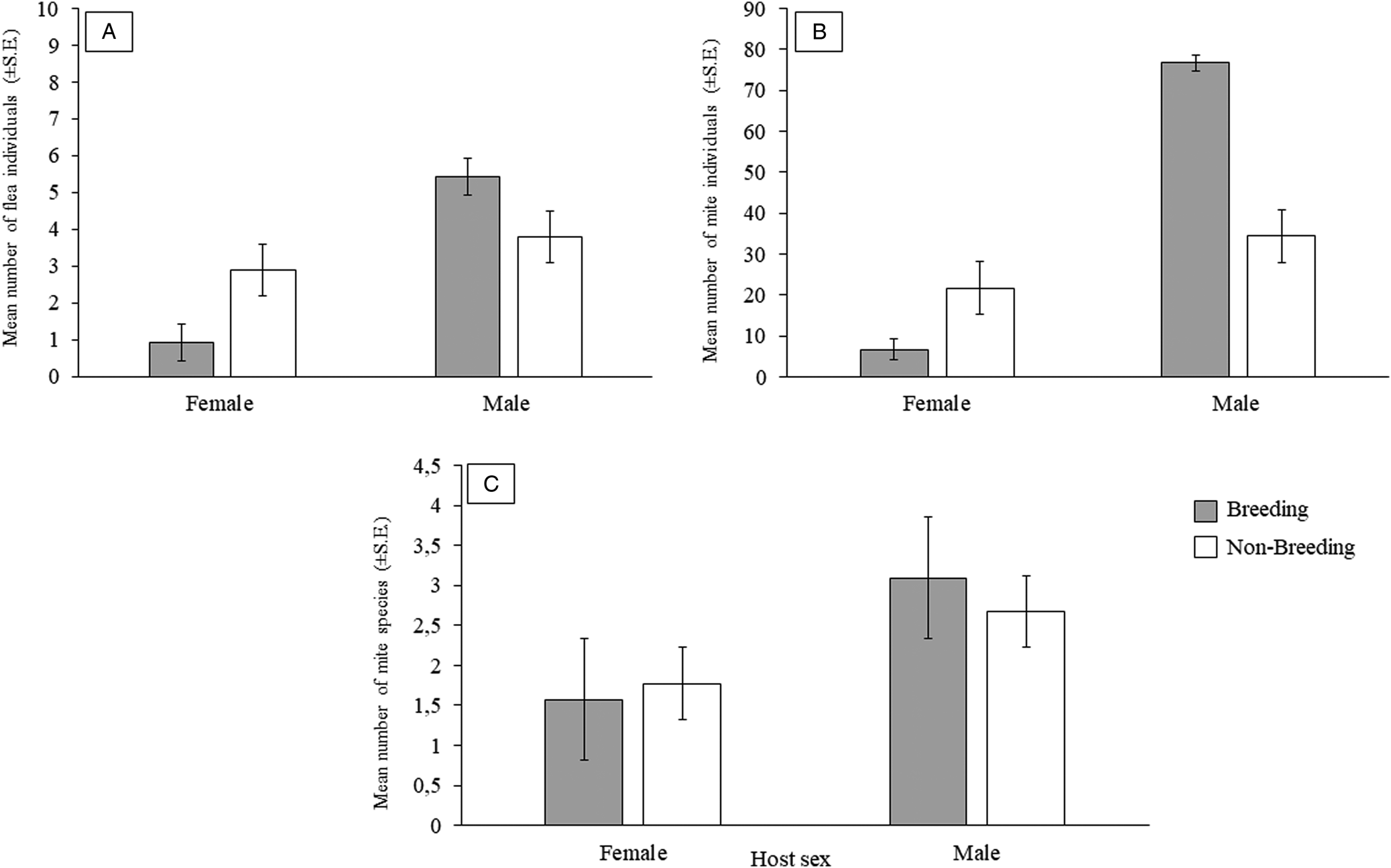
Figure 2. Mean number of: (A) flea individuals (±s.e.), (B) mite individuals (±s.e.) and (C) mite species (±s.e.) per host sex and per reproductive state for Gerbilliscus leucogaster (n = 123*) in Mpumalanga, South Africa, 2014–2020. *Data on reproductive state was only available for 123 individuals.
Table 5. Summary of model-averaged (conditional average) coefficients for generalized linear mixed-effects models with negative binomial distribution on the effect of host sex (SX), reproductive state (RS) and habitat type (HBT) on the epifaunistic taxon abundance belonging to different higher taxa on Gerbilliscus leucogaster (n = 123a)

The random factor in all models was year. R 2c and R 2m are – conditional and marginal R 2. Significance of estimates –.
a Data on reproductive state was only available for 123 individuals.
***P < 0.001, **P < 0.01, *P < 0.05.
Table 6. Summary of model-averaged (conditional average) coefficients for generalized linear mixed-effects models with poisson distribution on the effect of host sex (SX) and habitat type (HBT) on the epifaunistic taxon richness belonging to different higher taxa on Gerbilliscus leucogaster (n = 123a)

The random factor in all models was year. R 2c and R 2m are – conditional and marginal R 2. Significance of estimates – ***P < 0.001, **P < 0.01, *P < 0.05.
a Data on reproductive state was only available for 123 individuals.
Overall, a higher number of epifaunistic individuals were recorded on G. leucogaster in natural than agricultural habitats (3984 vs 2244, respectively) (Supplementary Table 2). However, a significant relationship was only recorded for ticks with more tick individuals (overall mean: 2.31 ± 0.66 vs 0.34 ± 0.16, respectively) and species (overall 5 vs 3 species, respectively) collected from rodents captured in natural as compared to agricultural habitats (Fig. 3A and 3B; Tables 5 and 6). The overall prevalence was also higher on G. leucogaster that occur in natural (48.53%) compared to agricultural (13.79%) habitat type (Supplementary Table 2).
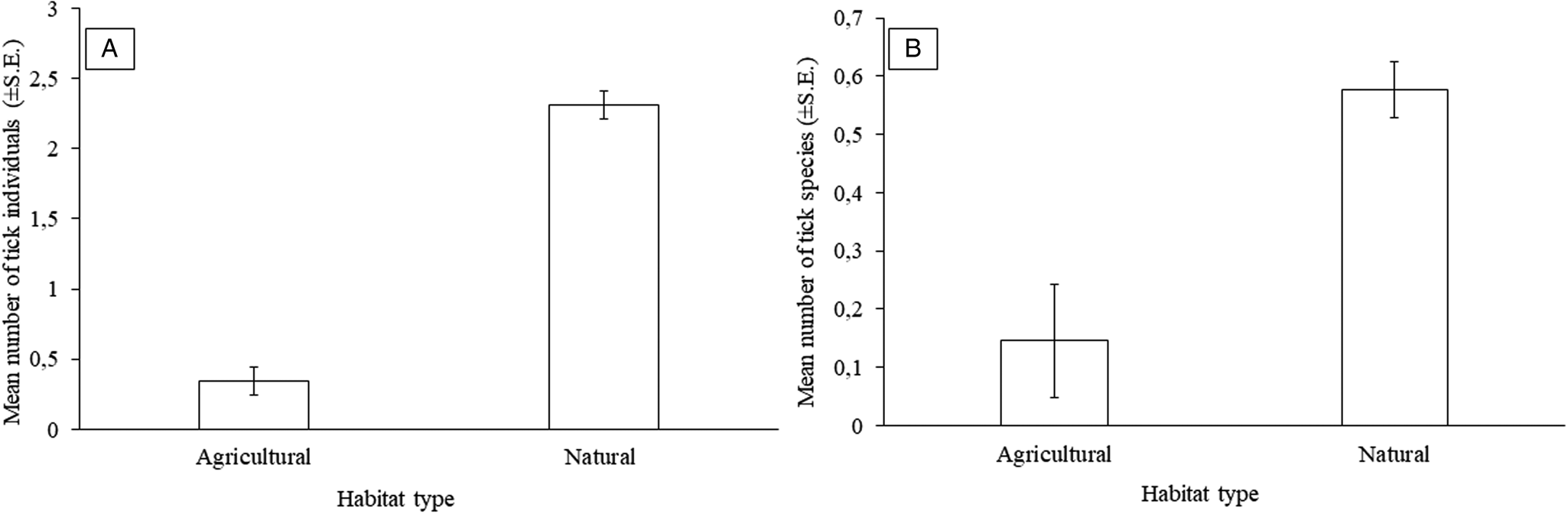
Figure 3. Mean number of: (A) tick individuals (±s.e.) and (B) tick species (±s.e.) per habitat type for Gerbilliscus leucogaster (n = 127) in Mpumalanga, South Africa, 2014–2020.
Discussion
Epifaunistic diversity
The flea X. frayi was the most common species, which supports a close association between X. frayi and G. leucogaster as reported by Segerman (Reference Segerman1995) and Braack et al. (Reference Braack, Horak, Jordaan, Segerman and Louw1996). The study area in the present study falls within the known distributional range of X. frayi, which spans the eastern and north-eastern Savanna bushveld areas of South Africa (Segerman, Reference Segerman1995). The low occurrence of X. brasiliensis may be due to the fact that other rodent species (e.g. Rattus spp. and Mastomys spp.) represent principal hosts for this flea (Segerman, Reference Segerman1995; Braack et al., Reference Braack, Horak, Jordaan, Segerman and Louw1996). The presence of X. bechuanae on G. leucogaster in the present study could be accidental because (a) X. bechuanae is reported as host-specific to the pouched mouse (Saccostomus campestris) (Segerman, Reference Segerman1995) co-occurring with G. leucogaster and (b) only 2 individuals of X. bechuanae were recorded. In addition, a similar pattern was previously recorded for X. bechuanae on G. leucogaster and S. campestris in Namibia (Shihepo et al., Reference Shihepo, Eiseb and Cunningham2008).
The occurrence of 2 louse species, H. biseriata and P. biseriata, supports earlier findings (Ledger, Reference Ledger1980; Braack et al., Reference Braack, Horak, Jordaan, Segerman and Louw1996). Polyplax biseriata was the most prevalent of the 2 species, and only 4 H. biseriata individuals were recorded. The dominance of P. biseriata is supported by Braack et al. (Reference Braack, Horak, Jordaan, Segerman and Louw1996). Unfortunately, Braack et al. (Reference Braack, Horak, Jordaan, Segerman and Louw1996) did not provide differential prevalence values for the 2 louse species, but rather an overall prevalence of 71.70%, which is comparable to the 76.38% recorded in our study.
The occurrence of H. truncatum on G. leucogaster in the present study supports the findings of Braack et al. (Reference Braack, Horak, Jordaan, Segerman and Louw1996) who recorded the tick species on 20% of G. leucogaster at a locality in the same geographic region as the present study. According to Horak et al. (Reference Horak, Heyne, Williams, Gallivan, Spickett, Bezuidenhout and Estrada-Peña2018) the immature stages of H. truncatum seem to prefer G. leucogaster in addition to some other host species. Dermacentor rhinocerinus was the second most prevalent tick. Horak and Cohen (Reference Horak and Cohen2001) also recorded this tick on G. leucogaster in the Mthethomusha Game Reserve in Mpumalanga Province. It is thus possible that G. leucogaster is a preferred host of the immature stages of this tick. Morphological stasis of larval and nymph life stages often limits species-level identification for ticks in the genera Rhipicephalus and Haemaphysalis (see Walker et al., Reference Walker, Keirans and Horak2000 for the genus Rhipicephalus).
Mites (including chiggers) represented the majority (23 of the 32 species) of the epifaunistic arthropods on G. leucogaster. The 3 most prevalent mite species are parasitic and belong to Laelapidae (Androlalaps oliffi, A. marshalli and A. theseus). Two species (A. marshalli and A. theseus) were previously recorded on G. leucogaster in the Savanna biome (Braack et al., Reference Braack, Horak, Jordaan, Segerman and Louw1996). Zumpt (Reference Zumpt1961) also lists A. oliffi, A. marshalli, A. taterae and A. theseus on several Gerbilliscus species (including G. leucogaster). The dominance of Androlaelaps species, compared to Laelaps species, on G. leucogaster in the present supports earlier findings (Braack et al., Reference Braack, Horak, Jordaan, Segerman and Louw1996) and this), gerbil seems to be the main host for Androlaelaps mites in southern Africa (Zumpt, Reference Zumpt1961; Till, Reference Till1963). The predominance of a female-bias of Laelapidae in the present study is in accordance with previous studies on rodents in South Africa (Matthee et al., Reference Matthee, Horak, Beaucournu, Durden, Ueckermann and Mcgeoch M2007, Reference Matthee, McGeoch and Krasnov2010) and in other regions (Martins-Hatano et al., Reference Martins-Hatano, Gettinger and Bergallo2002; Gettinger and Gardner, Reference Gettinger and Gardner2005, Reference Gettinger and Gardner2017). Androlaelaps and Laelaps females are generally found on the hosts' bodies whereas males and immature individuals are frequently in the nest (Radovsky, Reference Radovsky and Houck1994). However, exceptions do occur, where male and immature life stages are more represented on the host's body; as seen in Laelaps dearmasi (Tipton et al., Reference Tipton, Altman, Keenan, Wenzel and Tipton1966) and A. oliffi (this study). We provide the first record of Listrophoroides (Afrolistrophoroides) mastomys on G. leucogaster (4.75% prevalence). Listrophoroides (A.) mastomys was previously recorded on the Natal multimammate mouse (Mastomys natalensis) in north and west Africa (e.g. Rwanda, Uganda and Ivory Coast) (Fain, Reference Fain1972; Dusbabek, Reference Dusbabek1983). Species in the fur mite genus Listrophoroides are associated with rodents, shrews and primates and are globally distributed (Fain and Bochkov, Reference Fain and Bochkov2004). The presence of another fur mite, the myobiid Austromyobia forcipifer on G. leucogaster in our study is not surprising as mites in this genus are known exclusively from murid rodents (Bochkov, Reference Bochkov2009). Members of the Myobidae are morphologically specialized to attach themselves firmly to the fur and hair of mammals (Wall and Shearer, Reference Wall, Shearer, Wall and Shearer2001; Herrera-Mares et al., Reference Herrera-Mares, Guzman-Cornejo and Morales-Malacara2021). The macronyssid mite, Ornithonyssus bacoti is a bloodsucking ectoparasite that only attaches to the host (birds and mammals) during feeding (Wall and Shearer, Reference Wall, Shearer, Wall and Shearer2001). Although the presence of this species on G. leucogaster is the first record, it has been recorded on the four-striped mouse in the Western Cape Province of South Africa (Matthee et al., Reference Matthee, Horak, Beaucournu, Durden, Ueckermann and Mcgeoch M2007). Interestingly, although A. forcipifer and O. bacoti were recorded in low abundance and prevalence in the present study, they were both more common in the agricultural habitat type. The 4 predatory mite species that were recorded represent 4 families: Pachylaelapidae (Pachylaelaps sp.), Uropodidae, Cheyletidae (C. zumpti) and Acaroidae. The Pachylaelapidae are predators of micro-fauna (arthropods and soil-dwelling nematodes) in litter, humus, moss and are found in the nests of mammals, birds and insects (Lindquist et al., Reference Lindquist, Krantz, Walter, Krantz and Walter2009). Uropodidae are found in highly organic, insular deposits of manure and compost where they feed on bacteria, fungi, ants, nematodes and other mites (Lindquist et al., Reference Lindquist, Krantz, Walter, Krantz and Walter2009). Approximately 78% of cheyletid species are predators, the remaining species are permanent parasites of mammals and birds. Cheyletus zumpti was previously recorded in the nests of rodents at various localities in South Africa and tropical African countries (Rwanda, Nigeria, Angola and the Democratic Republic of the Congo) (Fain and Bochkov, Reference Fain and Bochkov2001). A single specimen was previously found on G. leucogaster in Skukuza in the Kruger National Park (Zumpt, Reference Zumpt1961). Predacious individuals occupy a wide variety of habitats including plant and soil-litter and are mostly associated with nests of vertebrates or stored grains (Hughes, Reference Hughes1976; Bochkov and OConnor, Reference Bochkov and OConnor2004). The deutonymphs of the Acaroidae recorded in this study, attach themselves to insects or fur of animals, and use them as transport vehicles between habitats (also known as phoresy). Members of the Acaroidae are mainly fungivorous or saprophytic (Lindquist et al., Reference Lindquist, Krantz, Walter, Krantz and Walter2009).
The chigger, S. lumsdeni, is known from the Savanna biome where it was recorded on tree squirrels (Paraxerus cepapi) (Zumpt, Reference Zumpt1961; Skinner and Chimimba, Reference Skinner and Chimimba2005; Stekolnikov, Reference Stekolnikov2018) and the pouched mouse (Matthee et al., Reference Matthee, Stekolnikov, Van Der Mescht, Froeschke and Morand2020). Gahrliepia nana is known to parasitize the common mole rat (Cryptomys hottentotus), the lesser leaf-nose bat (Hipposideros caffer) and the Namaqua rock mouse in the Grassland biome in the central-eastern and eastern region of South Africa (Gauteng and Kwa-Zulu Natal) (Zumpt, Reference Zumpt1961; Stekolnikov, Reference Stekolnikov2018; Matthee et al., Reference Matthee, Stekolnikov, Van Der Mescht, Froeschke and Morand2020; Stevens et al., Reference Stevens, Stekolnikov, Ueckermann, Horak and Matthee2022). Schoutedenichia morosi was previously recorded on the Cape gerbil (Gerbilliscus afra) and vlei rat (Otomys irroratus) in the south-eastern Grassland biome of Lesotho (Zumpt, Reference Zumpt1961; Stekolnikov, Reference Stekolnikov2018). Although S. dutoiti was described by Zumpt (Reference Zumpt1961) on the South African pouched mouse in the south-eastern part of South Africa, its presence on G. leucogaster has earlier been reported by Matthee et al. (Reference Matthee, Stekolnikov, Van Der Mescht, Froeschke and Morand2020) in the same locality. Microtrombicula mastomyia is known from Central and West Africa where it has a broad host range. Its presence in South Africa was marked as a new country locality by Matthee et al. (Reference Matthee, Stekolnikov, Van Der Mescht, Froeschke and Morand2020) but it was also reported on the Namaqua rock mouse in the Savanna biome by Stevens et al. (Reference Stevens, Stekolnikov, Ueckermann, Horak and Matthee2022). The remaining chigger species, A. ueckermanni, S. horaki and T. walkerae, were recently described as new (Stekolnikov and Matthee, Reference Stekolnikov and Matthee2019). Stekolnikov and Matthee (Reference Stekolnikov and Matthee2019) noted that Trombicula walkerae represented the first record of the genus Trombicula sensu stricto on the African continent. The Ascoschoengastia genus is known from 4 species in Africa. However, the recently described A. ueckermanni represents the first record for this genus in South Africa where it has been recorded on Mastomys sp. and the Tete veld rat (Aethomys ineptus). The genus Schoutedenichia is well represented in Africa (Stekolnikov, Reference Stekolnikov2018) and the recently described species, S. horaki, has been recorded on Mastomys sp. and the pouched mouse.
In this study, the pinna was one of the preferred parasitopes for chiggers. This parasitope was also recorded for a Leptotrombidium species on the white-footed mouse (Peromyscus leucopus) in northern Michigan (Wrenn, Reference Wrenn1974). Additionally, Goff (Reference Goff1979) noted that 96% of Guntheria omega were associated with the ear fringe of rodents in Papua New Guinea. In South Africa, this parasitope was previously recorded for chiggers on the Namaqua rock mouse in the Savanna (Fagir et al., Reference Fagir, Ueckermann, Horak, Bennett and Lutermann2014; Stevens et al., Reference Stevens, Stekolnikov, Ueckermann, Horak and Matthee2022) and the Grassland biome (Stevens et al., Reference Stevens, Stekolnikov, Ueckermann, Horak and Matthee2022). Here, we found that the tail base was another preferred parasitope. The tail base and perineum of the host were also previously recorded for chiggers on rodents in South Africa (Barnard et al., Reference Barnard, Krasnov, Goff and Matthee2015; Stevens et al., Reference Stevens, Stekolnikov, Ueckermann, Horak and Matthee2022).
Effects of host- and habitat-associated factors
Adult males (especially reproductively active) harboured significantly higher flea and mite counts. This pattern is supported by previous studies on ectoparasites associated with rodents in South Africa (Matthee et al., Reference Matthee, McGeoch and Krasnov2010; Archer et al., Reference Archer, Bennett, Ueckermann and Lutermann2014; Fagir et al., Reference Fagir, Horak, Ueckermann, Bennett and Lutermann2015) and elsewhere (Kowalski et al., Reference Kowalski, Bogdziewicz, Eichert and Rychlik2015; Hamidi and Bueno-Marí, Reference Hamidi and Bueno-Marí2021). As mentioned above, male biased ectoparasite infestation can be a result of several, not mutually exclusive, factors. Among them, sexual size dimorphism cannot explain sexual differences in ectoparasite infestation of G. leucogaster because males and females of this species are similar in size, as was also found in our study (Skinner and Chimimba, Reference Skinner and Chimimba2005). Consequently, these differences might be due to other mechanisms. For example, behavioural activities such as grooming and vagility (Krasnov et al., Reference Krasnov, Bordes, Khokhlova and Morand2012; Akinyi et al., Reference Akinyi, Tung, Jeneby, Patel, Altmann and Alberts2013). Indeed, Lötter and Pillay (Reference Lötter and Pillay2012) reported that female G. leucogaster groom more frequently than males. Given that grooming is an effective method to reduce ectoparasite infestations (Hawlena et al., Reference Hawlena, Bashary, Abramsky and Krasnov2007, Reference Hawlena, Bashary, Abramsky, Khokhlova and Krasnov2008), this may explain lower flea and mite counts and fewer mite species on females (Hart et al., Reference Hart, Hart, Mooring and Olubayo1992; Mooring et al., Reference Mooring, Blumstein and Stoner2004). Regarding vagility, our sampling was mainly carried out during the breeding season (September and October) of G. leucogaster. It is thus possible that reproductively active males roamed more widely than females (Wang et al., Reference Wang, Liu, Wang, Wan and Zhong2011; Gromov, Reference Gromov, Triunveri and Scalise2012). Burdelov et al. (Reference Burdelov, Leiderman, Khokhlova, Krasnov and Degen2007) demonstrated that starving fleas are positively phototactic and will therefore cluster at the openings of abandoned burrows and wait for a potential host (Darskaya and Besedina, Reference Darskaya and Besedina1961). More frequent roaming and larger home ranges by reproductively active males may result in higher visitation rates at burrows of other rodents, where they may encounter fleas and mites (Krasnov and Matthee, Reference Krasnov and Matthee2010). Although elevated testosterone levels during the breeding season may be another important contributing factor to male-biased infestations (Zuk and McKean, Reference Zuk and McKean1996; Hughes and Randolph, Reference Hughes and Randolph2001; Ezenwa et al., Reference Ezenwa, Stefan Ekernas and Creel2012), there is not consistent support for the association between high testosterone levels and parasite infestations (Grear et al., Reference Grear, Perkins and Hudson2009; O'Brien et al., Reference O'Brien, Waterman, Anderson and Bennett2018).
In the present study, hosts captured in the natural compared to the agricultural habitat harboured more ticks. Several ixodid tick species require multiple host species and a favourable external environment, such as vegetation, to complete their life cycle (Cupp, Reference Cupp1991; Horak et al., Reference Horak, Heyne, Williams, Gallivan, Spickett, Bezuidenhout and Estrada-Peña2018). It is therefore not surprising that studies have reported a significant relationship between habitat type and tick occurrence (Gray, Reference Gray1998; Jaenson et al., Reference Jaenson, Eisen, Comstedt, Mejlon, Lindgren, BergstrÖm and Olsen2009; Ledger et al., Reference Ledger, Keenan, Sayler and Wisely2019). The vegetation structure that is associated with a particular habitat type can have direct and indirect effects on ticks. Firstly, vegetation structure can directly affect the microclimatic conditions to which free-living tick life stages are exposed (Schulze and Jordan, Reference Schulze and Jordan2005; Tack et al., Reference Tack, Madder, Baeten, Vanhellemont, Gruwez and Verheyen2012; Ledger et al., Reference Ledger, Keenan, Sayler and Wisely2019). For example, canopies of woody plants alter the microclimate beneath and around them by intercepting precipitation and by shading, which increases soil moisture (Breshears et al., Reference Breshears, Nyhan, Heil and Wilcox1998; Potts et al., Reference Potts, Scott, Bayram and Carbonara2010; Lozano-Parra et al., Reference Lozano-Parra, Pulido, Lozano-Fondón and Schnabel2018). In addition, a layer of vegetation and leaf litter can insulate the soil and buffer it against extreme heat and cold temperatures (Pierson and Wight, Reference Pierson and Wight1991; Breshears et al., Reference Breshears, Rich, Barnes and Campbell1997). Tick development and survival in the external environment is therefore facilitated in more sheltered habitats with a permanent vegetation layer and a more stable microclimate (Pfäffle et al., Reference Pfäffle, Littwin, Muders and Petney2013; Paul et al., Reference Paul, Cote, Le Naour and Bonnet2016). Tick genera recorded in the present study are 2- and 3-host ticks (i.e. those that need to find 2 or 3 different hosts, respectively, to complete their life cycle) (Horak et al., Reference Horak, Heyne, Williams, Gallivan, Spickett, Bezuidenhout and Estrada-Peña2018). Favourable microclimatic conditions seem to be particularly important for these taxa as their larval and nymphal life stages quest for hosts from the soil surface or from grass tufts (Horak and Cohen, Reference Horak and Cohen2001; Gallivan et al., Reference Gallivan, Spickett, Heyne, Spickett and Horak2011). In addition, shrub and grass cover is important for ticks to quest and search for a host (Ledger et al., Reference Ledger, Keenan, Sayler and Wisely2019; Mathews-Martin et al., Reference Mathews-Martin, Namèche, Vourc'h, Gasser, Lebert, Poux, Barry, Bord, Jachacz, Chalvet-Monfray, Bourdoiseau, Pamies, Sepúlveda, Chambon-Rouvier and René-Martellet2020). Apart from Hy. truncatum nymphs, all the nymphs from the tick taxa are dependent on vegetation to quest and find a host (Horak et al., Reference Horak, Heyne, Williams, Gallivan, Spickett, Bezuidenhout and Estrada-Peña2018). In the present study, the pristine natural habitat had a higher proportion of grass (80 and 79%, respectively) than the agricultural habitat type (70 and 62%, respectively) during August 2014 and January 2015 (S. Matthee unpublished data). It is therefore possible that natural habitats provide a conducive microclimate and physical structures that facilitate tick development and survival (Dube et al., Reference Dube, Hund, Turbek and Safran2018; Shilereyo et al., Reference Shilereyo, Magige, Ranke, Ogutu and Røskaft2022). Lastly, in the present study the natural habitat supports a larger diversity of vertebrate hosts and a larger diversity of small and large-bodied vertebrate families (e.g. Bovidae, Canidae, Giraffidae and Rhinocerotidae) (Du Toit, Reference Du Toit, du Toit, Rogers and Biggs2003) of which the latter act as natural hosts for the adult life stages (Horak et al., Reference Horak, Heyne, Williams, Gallivan, Spickett, Bezuidenhout and Estrada-Peña2018). This contrasts with the agricultural habitat type that comprises small crop fields that generally harbour rodents and are infrequently visited by dogs, cattle and goats (personal observation). In addition, habitat types with a higher proportion of larger bodied vertebrate hosts have a higher abundance of ticks (Horak et al., Reference Horak, Junker and Krasnov2022) and more adult tick life stages (Esser et al., Reference Esser, Foley, Bongers, Herre, Miller, Prins and Jansen2016).
This study represents the first systematic long-term assessment of the ectoparasite species associated with G. leucogaster. We conclude that G. leucogaster is host to a large diversity of epifaunistic species of which mites represent a significant proportion. The relationships recorded between ectoparasite infestations, and the host and habitat factors were life history specific. In particular, the level of infestation by ectoparasites closely associated with the host (fleas and mites) was affected by host-associated factors, while infestation by ectoparasites that spend most of their life in the external environment (ticks) was affected by habitat type. Although the study was limited to local conditions, it provides a valuable baseline for future broader scale studies on G. leucogaster in South and southern Africa.
Supplementary material
The supplementary material for this article can be found at https://doi.org/10.1017/S0031182023000562
Acknowledgement
The authors wish to thank the staff at Manyeleti nature reserve and the community leaders for permitting us to conduct fieldwork in the reserve and the various villages in the Mnisi community. The project would not have been possible without the support from property owners and local Environmental Monitors. Several postgraduate students, fellow researchers and research assistants supported the field and laboratory work. In particular, Liezl Retief, Dina Fagir, Jeanette Wentzel, Ilana van Wyk, Marinda Oosthuizen, Nicola Collins, Luis Neves, Armanda Bastos, Conrad Matthee, Luther van der Mescht, Götz Froeschke, Marcela Espinaze and Alyssa Little are thanked for logistical and technical support. We are grateful to Alexandr A. Stekolnikov (Zoological Institute of the Russian Academy of Sciences, Saint Petersburg, Russia) for his contribution to the identification of the chigger species found in this study.
Authors’ contribution
SM conceived the study and supervised ATS. ATS conducted the field and laboratory work and wrote the draft chapters of the article. IGH assisted with the identification of ticks. EAU identified the mites (excluding chiggers). BK assisted with the data analysis. All authors contributed to the final version of the article.
Financial support
Funding was provided by Stellenbosch University and the South African National Research Foundation (NRF) [GUN 85718 and GUN 129276 (to S. Matthee)]. Amber Smith was funded by a postgraduate bursary from the Department of Conservation Ecology and Entomology (Stellenbosch University) and a NRF Master's Innovation bursary (grant number 128978 and 138828). Any opinion, finding and conclusion or recommendation expressed in this material is that of the authors and the NRF does not accept any liability in this regard. Research reported in this publication was supported by the National Institute of Allergy and Infectious Diseases of the National Institutes of Health under Award Number R01AI136832 (PI: Prof MC Oosthuizen). The content is solely the responsibility of the authors and does not necessarily represent the official views of the National Institutes of Health.
Conflict of interest
None.
Ethical standards
The project was approved by Mpumalanga Tourism and Parks Agency (permit number ES 5/14, MPB. 5694; MPB. 5663), Department of Agriculture, Forestry and Fisheries (Reference number 12/11/1/7/5), the Animal Ethics Committees of Stellenbosch University (Reference numbers ACU2016-00190; ACU2018-4555; ACU2020-17062) and Pretoria University (Reference numbers V046-14; VO23-19).
Data availability
All data generated or analysed during this study are included in this published article. The datasets used and/or analysed are available from the corresponding author upon reasonable request.