Introduction
Humans are altering ecosystems on a scale never seen before, which has led to problems from high environmental pollution and biodiversity loss to outbreaks of infectious diseases (McMahon et al., Reference McMahon, Morand and Gray2018; Keys et al., Reference Keys, Galaz, Dyer, Matthews, Folke, Nyström and Cornell2019). The continuous fragmentation of natural habitats has increased contacts of wildlife with domesticated animals and humans, leading to the risk of pathogen concentration and spillover (Deplazes et al., Reference Deplazes, Hegglin, Gloor and Romig2004; Faust et al., Reference Faust, McCallum, Bloomfield, Gottdenker, Gillespie, Torney, Dobson and Plowright2018) and making parasitic diseases of wildlife a rising One Health concern (Waindok et al., Reference Waindok, Raue, Grilo, Siebert and Strube2021; Casulli et al., Reference Casulli, Massolo, Saarma, Umhang, Santolamazza and Santoro2022). One Health emphasizes the interdependence of human, animal and environmental health, and emerging infectious diseases of wildlife origin have made the concept of considerable importance (Jenkins et al., Reference Jenkins, Simon, Bachand and Stephen2015).
Among the most abundant mammalian carnivores in Europe and Estonia are the red fox (Vulpes vulpes) and raccoon dog (Nyctereutes procyonoides), and together with the golden jackal (Canis aureus), they are displaying opportunistic food habits (Kauhala et al., Reference Kauhala, Laukkanen and Rége1998; Baltrunaite, Reference Baltrunaite2002; Soe et al., Reference Soe, Davison, Süld, Valdmann, Laurimaa and Saarma2017; Lange et al., Reference Lange, Lelieveld and de Knegt2021). Their food includes small mammals, insects, reptiles and fish that can act as vectors or paratenic hosts for helminths, playing an important role in transmissions of parasitic helminths of zoonotic potential, including tapeworms such as Echinococcus spp., Taenia spp., and roundworms like Trichinella spp. and Toxocara spp. Although a number of zoonotic diseases caused by parasitic helminths have been drastically reduced due to improved hygiene and prophylactic treatment, many parasitic zoonoses remain a considerable health and economic burden due to ineffective control programmes. Mammalian predators such as the red fox, golden jackal, pine marten (Martes martes) and raccoon dog are common definitive hosts for many zoonotic helminths in Estonia and many other countries in Europe and beyond. However, these species are known to move often in areas where domestic animals and humans reside (e.g., Plumer et al., Reference Plumer, Davison and Saarma2014; Süld et al., Reference Süld, Saarma and Valdmann2017a). Defecating in these areas contaminates the environment with various pathogens, including zoonotic helminths. Studies have found that in Europe, raccoon dogs are infected with a minimum of 32 helminth species of which 19 are zoonotic (Laurimaa et al., Reference Laurimaa, Moks, Soe, Valdmann and Saarma2016a). It is also known that red foxes and golden jackals are hosts for many helminth parasites, most of them infecting also domestic dogs and cats (e.g., Otranto et al., Reference Otranto, Cantacessi, Dantas-Torres, Brianti, Pfeffer, Genchi, Guberti, Capelli and Deplazes2015; Gherman and Mihalca, Reference Gherman and Mihalca2017; Karamon et al., Reference Karamon, Dąbrowska, Kochanowski, Samorek-Pieróg, Sroka, Różycki, Bilska-Zając, Zdybel and Cencek2018). Moreover, foxes have been colonizing urban areas in Europe, including Estonia, bringing zoonotic pathogens to the vicinity of humans and their pets (Deplazes et al., Reference Deplazes, Hegglin, Gloor and Romig2004; Laurimaa et al., Reference Laurimaa, Davison, Plumer, Süld, Oja, Moks, Keis, Hindrikson, Kinkar, Laurimäe, Abner, Remm, Anijalg and Saarma2015a).
Red fox and raccoon dog are among the most widely distributed canid species in Estonia and act as vectors of multiple zoonotic diseases, including alveolar echinococcosis, trichinellosis and sarcoptic mange (Süld et al., Reference Süld, Valdmann, Laurimaa, Soe, Davison and Saarma2014, Reference Süld, Tammeleht, Valdmann and Saarma2017b; Laurimaa et al., Reference Laurimaa, Davison, Süld, Plumer, Oja, Moks, Keis, Hindrikson, Kinkar, Laurimäe, Abner, Remm, Anijalg and Saarma2015b, Reference Laurimaa, Moks, Soe, Valdmann and Saarma2016a, Reference Laurimaa, Süld, Davison, Moks, Valdmann and Saarma2016b; Marcinkutė et al., Reference Marcinkutė, Šarkūnas, Moks, Saarma, Jokelainen, Bagrade, Laivacuma, Strupas, Sokolovas and Deplazes2015; Kärssin et al., Reference Kärssin, Häkkinen, Niin, Peik, Vilem, Jokelainen and Lassen2017). In Estonia, a total of 17 endoparasite taxa has been found in red foxes, 10 of them with zoonotic importance (Laurimaa et al., Reference Laurimaa, Süld, Davison, Moks, Valdmann and Saarma2016b), whereas for the raccoon dog the corresponding numbers were 17 and 9 (Laurimaa et al., Reference Laurimaa, Moks, Soe, Valdmann and Saarma2016a).
The golden jackal was included into the list of mammal species in Estonia nearly a decade ago and ever since the population has grown, mainly in Western Estonia, sharing overlapping territories with other wild and domestic animals (e.g., red foxes, raccoon dogs, dogs), and also humans. It is therefore important to assess which helminth infections the golden jackal as well as other canids transmit, especially those of zoonotic potential.
Apart from wild mammals, dogs can also be an important source of helminth infection, both in rural and urban areas. Recent investigations have found that about 10% of scats of urban dogs in Estonia were infected with the eggs of helminths (Tull et al., Reference Tull, Moks, Laurimaa, Keis and Süld2020), whereas in rural areas the infection rate was significantly higher, reaching up to 87% (Tull et al., Reference Tull, Valdmann, Rannap, Kaasiku, Tammeleht and Saarma2022). A zoonotic tapeworm parasite Echinococcus granulosus has also been recorded in dogs in Estonia (Laurimaa et al., Reference Laurimaa, Süld, Moks, Valdmann, Umhang, Knapp and Saarma2015c).
Estonia, especially its western part, provides the best opportunities to study the environmental contamination with the eggs of helminth parasites in a complex manner, analysing the role of mammalian predators, including red foxes and golden jackals, but also rural dogs. This part of the country hosts the largest population of golden jackals in Estonia (in other areas of the country the species is scarce) and, in addition, the data for rural dog helminths from this area has been obtained in a previous study (Tull et al., Reference Tull, Valdmann, Rannap, Kaasiku, Tammeleht and Saarma2022).
The aims were to: (1) determine helminth infections among wild mammalian predators; (2) evaluate the overlap between helminth faunas of different wild and domestic canids such as the red fox, golden jackal and dog; (3) assess the effect of diet on the risk of infection with helminths.
Materials and methods
Study area and samples
A non-probabilistic sampling was used for collecting scats of mammalian predators in rural areas of Western Estonia, namely in Matsalu National Park, Häädemeeste and Hiiumaa in April–June 2019 (Fig. 1). Each sample was placed into a separate plastic bag and tagged with unique ID, including global positioning system coordinates. To inactivate eggs of zoonotic parasites, for example Echinococcus multilocularis and E. granulosus s.l., which are endemic in Estonia (Moks et al., Reference Moks, Jõgisalu, Saarma, Talvik, Järvis and Valdmann2006, Reference Moks, Jõgisalu, Valdmann and Saarma2008; Laurimaa et al., Reference Laurimaa, Davison, Plumer, Süld, Oja, Moks, Keis, Hindrikson, Kinkar, Laurimäe, Abner, Remm, Anijalg and Saarma2015a, Reference Laurimaa, Davison, Süld, Plumer, Oja, Moks, Keis, Hindrikson, Kinkar, Laurimäe, Abner, Remm, Anijalg and Saarma2015b), samples were kept at −80°C for a minimum of 7 days. Data for dog samples (n = 84), that are described in detail in Tull et al. (Reference Tull, Valdmann, Rannap, Kaasiku, Tammeleht and Saarma2022) and are from the same study area, were also included to provide a comparison with other mammalian predators.
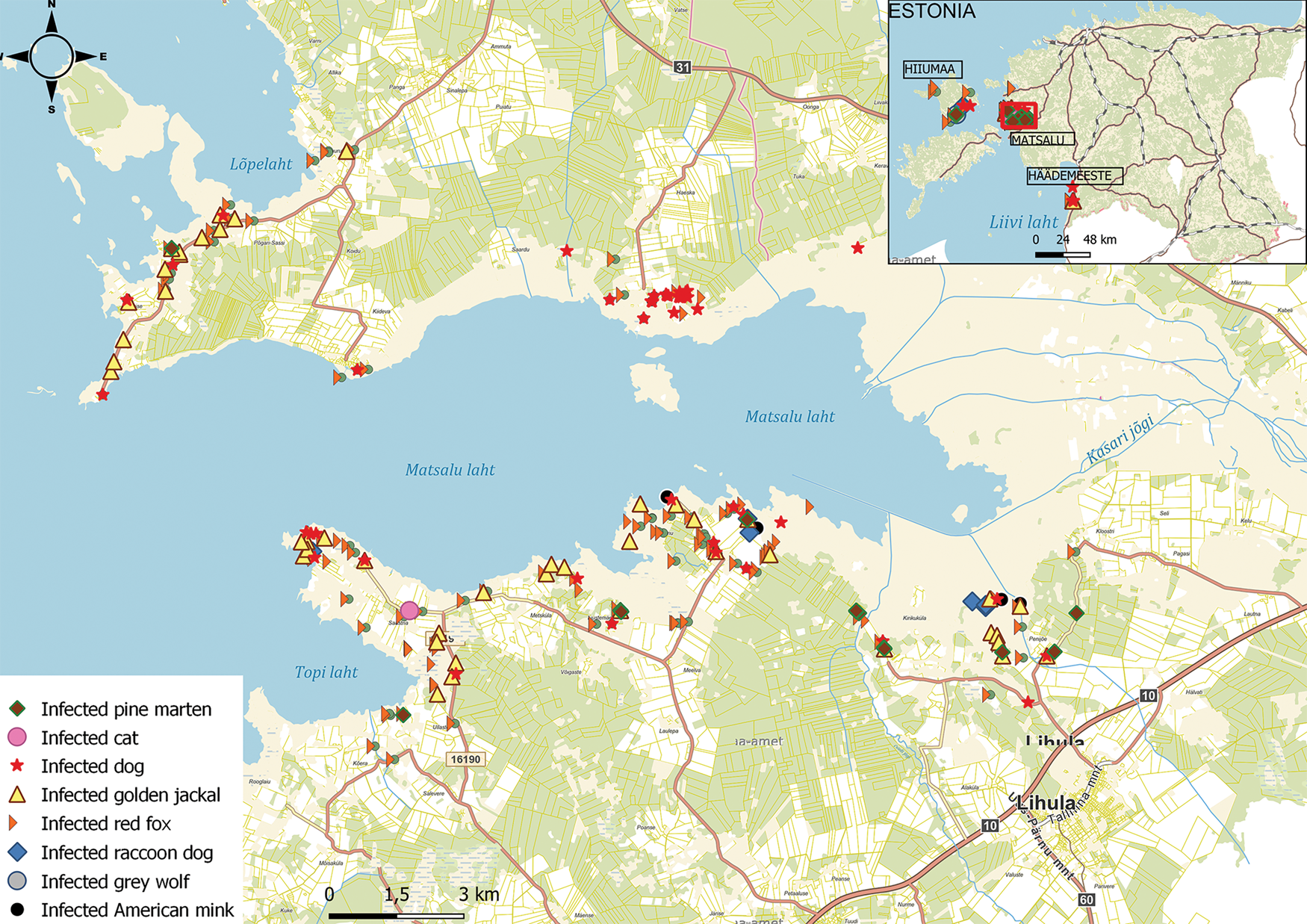
Fig. 1. Collection sites of predator scats infected with parasitic helminths (n = 287) in western part of Estonia. Samples are mainly from the Matsalu National Park (enlarged), but also from Hiiumaa and Häädemeeste. Dog data are from Tull et al. (Reference Tull, Valdmann, Rannap, Kaasiku, Tammeleht and Saarma2022).
Molecular identification of mammalian predators
Scats of different canid species are sometimes difficult to distinguish and to avoid mixing the data of various species, a genetic analysis was conducted for correct identification of mammalian species. Genomic DNA was isolated from scats using QIAamp Fast DNA Stool Mini Kit (Qiagen, Hilden, Germany) according to manufacturer's protocol. A hypervariable fragment of the mitochondrial DNA (mtDNA) control region that enables distinguishing wolves and dogs in Estonia, was PCR-amplified and sequenced as described in Plumer et al. (Reference Plumer, Talvi, Männil and Saarma2018). This mtDNA fragment allows also distinguishing other predators (Tull et al., Reference Tull, Valdmann, Rannap, Kaasiku, Tammeleht and Saarma2022). In brief, a 351 base-pair (bp) fragment of the mtDNA control region was PCR-amplified using 0.25 μM of primers Canis1F and Canis3R. The reaction mixture (20 μl in total), contained 2 μL of DNA, 1 × Phusion HF buffer, 0.4 mm dNTP and 0.4 U Phusion HS II polymerase (Thermo Fisher Scientific, Waltham, USA). The following PCR cycling parameters were used: 30 s at 98°C, then 10 cycles: 10 s at 98°C, 30 s at 68°C (with touchdown of −0.8°C per cycle), 45 s at 72°C; then 35 cycles: 10 s at 98°C, 30 s at 60°C, 45 s at 72°C and finally 2 min at 72°C. PCR products were purified with 1 U of both FastAP and ExoI (Thermo Fisher Scientific). Purified PCR products were sent for sequencing to the core laboratory of the Institute of Genomics at the University of Tartu.
Sequences of both DNA chains were aligned with CodonCode Aligner v.9.0.2 (CodonCode Corp; Centerville, MA, USA) to produce consensus sequences and corrected using BioEdit v.7.2.5 (Hall, Reference Hall1999). The final alignment was 245 bp and the dataset was further aligned with homologous wolf (Hindrikson et al., Reference Hindrikson, Männil, Ozolins, Krzywinski and Saarma2012; Plumer et al., Reference Plumer, Talvi, Männil and Saarma2018), red fox and golden jackal (Tull et al., Reference Tull, Valdmann, Rannap, Kaasiku, Tammeleht and Saarma2022) sequences from Estonia.
Molecular identification of food objects
For the identification of birds, mammals, reptiles and fish, a 303 bp fragment of mtDNA cox1 gene was PCR-amplified with primers AVS2F and AVS3R as described in Tull et al. (Reference Tull, Valdmann, Rannap, Kaasiku, Tammeleht and Saarma2022). PCR reactions were carried out in a total volume of 20 μL with 1× Phusion HF Buffer (Thermo Fisher Scientific), 0.2 mm dNTP, 0.25 μ m of each primer and 0.4 U Phusion Hot Start II DNA Polymerase and 2 μL of purified DNA. The PCR mixture was initially denatured at 98°C for 30 s, followed by 10 touchdown cycles for 10 s at 98°C, 20 s at 60°C (reducing the temperature 1°C per cycle) and 30 s at 72°C, followed by 30 cycles of 10 s at 98°C, 20 s at 50°C and 30 s at 72°C. In case the PCR was negative due to highly degraded DNA, we performed a second analysis by PCR-amplifying a shorter, 183 bp fragment of mtDNA 12S rRNA gene, using primers Ave12F and Ave12R, described in Oja et al. (Reference Oja, Soe, Valdmann and Saarma2017). PCR products were checked using 2% 1×TAE gel-electrophoresis and visualized under UV radiation using ethidium bromide.
PCR products were purified, sequenced and nucleotide BLAST (https://blast.ncbi.nlm.nih.gov/Blast.cgi) was used to identify various taxa, such as reptiles, fish and birds.
Morphological analysis of food objects
The analysis was done as described in Valdmann and Saarma (Reference Valdmann and Saarma2020). Shortly, fecal samples were processed according to standard laboratory procedures (Reynolds and Aebischer, Reference Reynolds and Aebischer1991). Non-mammal remains (e.g., birds) recovered in predator scats were identified in comparison with reference materials. Mammal remains were identified by examining the cuticular pattern and the medulla of the hairs using reference manuals (Teerink, Reference Teerink1991; Tóth, Reference Tóth2017) and hairs collected from hunted animals.
Parasite identification and prevalence
Helminth occurrence was determined using the concentration flotation technique (NaCl and glucose solution, specific gravity 1.2–1.3 g cm−3) (Roepstorff and Nansen, Reference Roepstorff and Nansen1998), followed by helminth egg counting in McMaster chambers until 100 eggs per parasite taxa. Identification of helminth taxa was based on morphological characteristics (Bowman, Reference Bowman2013). Most of the parasite taxa were identified at the genus level, except Toxocara canis, Toxascaris leonina and Uncinaria stenocephala. Although we attempted genetic identification of species among the isolated eggs of Taeniidae, it was not successful, possibly due to degradation of DNA.
Helminth prevalence was defined as the number of predator host infected with particular helminth taxa divided by the number of hosts examined for that helminth taxa. The relative infection intensity was determined as the count of eggs up to 100 per taxa in a sample.
Spatial analysis
Maps were created using the QGIS (v3.24) to visualize the geographical locations of predator scats and to calculate the average distance between private houses (henceforth privates) and all the collected scat samples (QGIS Development Team, 2022). This buffer distance around scat samples was considered as the average free-ranging area of mammalian predators from privates (Fig. 1). Another buffer was generated by joining together the first buffer with the infected scats layer situated inside the buffer zone to count privates in the potential hazard zone. The map layers originated from public WMS services (Estonian Land Board, 2022).
Statistical analysis
The dependent variables consisted of (co)infection prevalence and infection intensity. The independent variable consisted of food items (data not given; a separate manuscript on the diet of predators is in preparation). Food objects were divided into 5 categorical variables: game (mammals), bird, dog food, plant material and rodent.
Since multiple testing was performed between (co)infection and different food groups, it is possible to obtain false-positive results (Type I error) in a set of tests. The Holm–Bonferroni method, which is more powerful compared to Bonferroni procedure, was applied to prevent Type I error rates when performing multiple tests (Aickin and Gensler, Reference Aickin and Gensler1996).
Proportions were compared with SAS Studio (3.8) software using chi-squared tests of independence (PROC FREQ) to determine independent variables associated with overall (co)infection and single taxa prevalence (SAS Institute Inc, 2022). If 1 or more cells in the 2 × 2 contingency tables had expected values of less than 5, Fisher's exact test was used. Generalized linear models (GLM) package ‘glmmTMB’ (Brooks et al., Reference Brooks, Kristensen, Benthem, Magnusson, Berg, Nielsen, Skaug, Maechler and Bolker2017; R Core Team, 2022) was used to evaluate consumption of various food objects associated with individual and overall helminth prevalence and with the overall coinfection prevalence. It was also estimated how red fox and golden jackal diet associates with prevalence (binomial error distribution) and intensity (negative binomial error distribution) of individual helminth taxa. Models were compared using the Akaike information criterion corrected for small samples (AICc) (Burnham et al., Reference Burnham, Anderson and Burnham2002). Package ‘MuMIn’ (Barton, Reference Barton2019) was used for conducting model selection and model averaging. Only models with the highest Akaike weight wi(AIC) (ΔAICc < 2) were described as the model with the highest Akaike weights provide a continuous measure of strength of evidence. It is especially important to assess the weight of evidence in favour of the best model when a binary decision is made and the other candidate models (with higher AIC values) are simply discarded (Wagenmakers and Farrell, Reference Wagenmakers and Farrell2004). Furthermore, the weights (wi) of the same factors presented in 1 model set were summed for calculating the relative variable importance (RVI).
The non-parametric Mann–Whitney U test was used to compare the mean parasite richness between 3 larger canids (red fox, golden jackal, dog). All presented results of statistical tests had P value < 0.05.
To measure the overlap of helminths between 2 host species, the Pianka's index (Pianka, Reference Pianka1973) was calculated using the following formula:

where pij and pik are proportions of parasite taxa in the hosts j and k respectively, expressed as percentage.
Results
Based on the 624 scat samples collected from the coastal areas in Western Estonia, genetic analysis identified predator species for 315 samples. Among these, the analysis based on morphological examination of parasite ova revealed that 91.1% (n = 287) were infected (Table 1; Supplementary Material Fig. S1). Out of the infected scats, 38.3% (n = 110) harboured a single helminth taxon and 61.7% (n = 177) were coinfected with 2 or more helminth taxa (Supplementary Material Fig. S2). Majority of predator scats harboured eggs of Taeniidae (84.1%), followed by Eucoleus spp./Trichuris spp. (50.2%), U. stenocephala (20.3%), T. canis (8.9%), T. leonina (1.3%) (Table 1; Fig. 2). Eggs of Toxocara cati were found only in a single cat sample (0.3%) (Table 1).
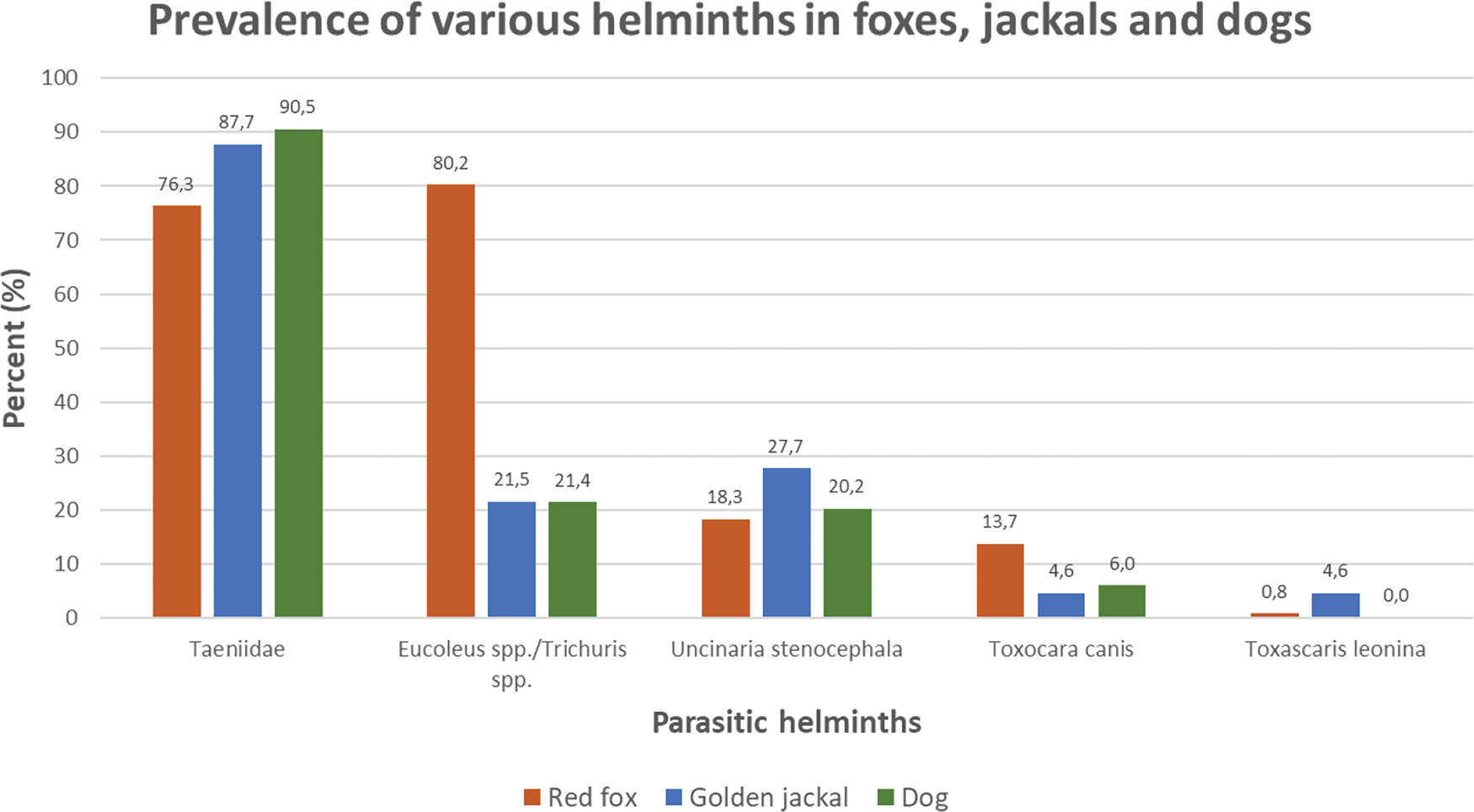
Fig. 2. Prevalence of different parasitic helminths in scats of the 3 most abundant mammalian predator species: red fox (n = 131), golden jackal (n = 65) and dog (n = 84).
Table 1. Prevalence of zoonotic helminths in scats of different mammalian predators (n = 315) of which 287 were infected
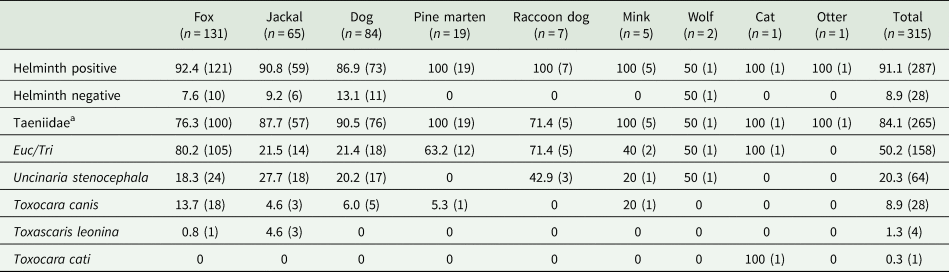
Dog data are from Tull et al. (Reference Tull, Valdmann, Rannap, Kaasiku, Tammeleht and Saarma2022). The first number is per cent (%) and the number of samples (n) is in parentheses. Euc/Tri: Eucoleus spp./Trichuris spp.
a Note that all helminth taxa listed in the table are of zoonotic potential. However, when some of the taeniids (e.g., Echinococcus spp., Taenia hydatigena, T. crassiceps) are zoonotic and a serious threat to human health, there are some that are not zoonotic (e.g., T. pisiformis, T. cervi).
Vast majority of red fox scat samples were infected (92.4%; Table 1; Supplementary Material Fig. S1). The most common infection was with Eucoleus spp./Trichuris spp. (80.2%), followed by Taeniidae (76.3%) and U. stenocephala (18.3%) (Table 1; Fig. 2). Among dog scats, 86.9% had helminth ova and the most common taxa were Taeniidae (90.5%), followed by Eucoleus spp./Trichuris spp. (21.4%) and U. stenocephala (20.2%). The golden jackal samples had comparably high proportion of infected scats (90.8%) with the red fox and dog. Golden jackal scats contained most frequently eggs of Taeniidae (87.7%), followed by U. stenocephala (27.7%) and Eucoleus spp./Trichuris spp. (21.5%). The non-parametric Mann–Whitney U test showed that red foxes had significantly higher parasite richness than dogs (P < 0.0001) and also higher than golden jackals (P < 0.0001). There was no significant difference between dogs and golden jackals.
Among all coinfected scats (n = 177), di-infections were the most frequent (75.1%), followed by tri-infections (22.6%) (Supplementary Material Fig. S3). Di-infection with Taeniidae and Eucoleus spp./Trichuris spp. dominated (52.5%), followed by di-infection with Taeniidae and U. stenocephala (14.1%), and tri-infection of Taeniidae, Eucoleus spp./Trichuris spp. and U. stenocephala (14.1%) (Fig. S4).
Red fox had the highest coinfection rate (80.2%) (Supplementary Material Fig. S2) of which the most prominent was coinfection with Taeniidae and Eucoleus spp./Trichuris spp. (62.9%) (Table 2; Fig. 3). The golden jackal had significantly lower coinfection rate (50.8%) of which the most frequent was coinfection with Taeniidae and U. stenocephala (36.7%). Among the scats of rural dogs, 39.7% harboured more than 1 helminth taxon. The most prevalent coinfection in dogs occurred between Taeniidae and U. stenocephala (41.4%).
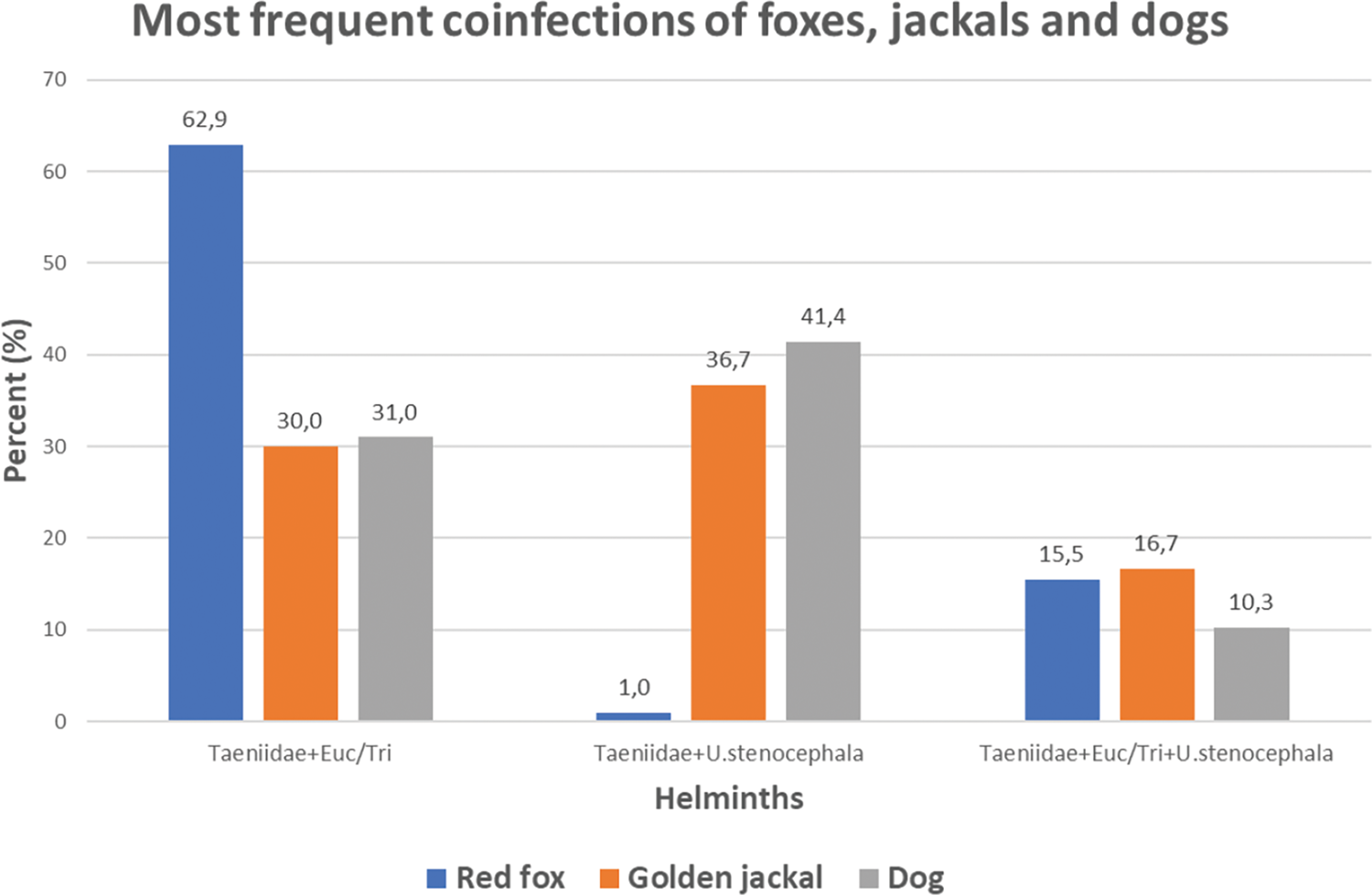
Fig. 3. Three most frequent helminth coinfections among coinfected scats of red foxes (n = 97), golden jackals (n = 30) and rural dogs (n = 29). Euc/Tri – Eucoleus spp./Trichuris spp.
Table 2. Helminth coinfections among different mammalian predators (n = 177)
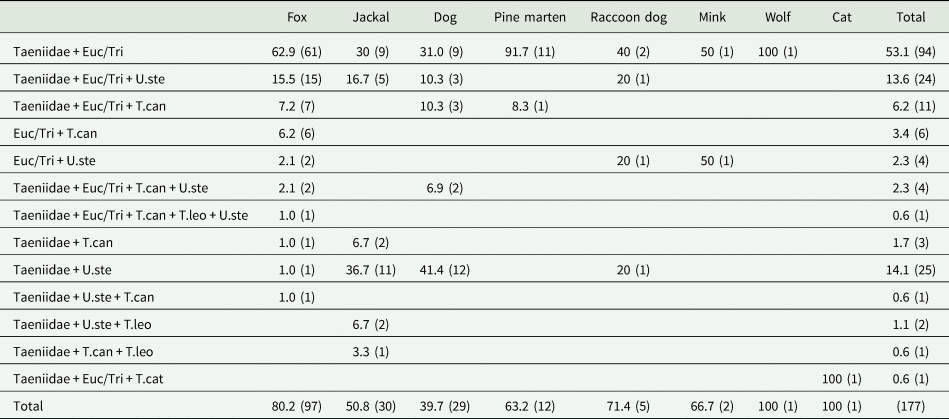
The first number is per cent (%) and the number of samples (n) is in parentheses. Euc/Tri: Eucoleus spp./Trichuris spp; T.can: Toxocara canis; T.cat: Toxocara cati; T.leo: Toxascaris leonina; U.ste: Uncinaria stenocephala.
The best coinfection model (wi = 0.22) showed that predators preying on rodents had significantly higher (2.5×) odds to be coinfected with multiple helminth taxa than predators who had not preyed on rodents (β RODENT = 0.9, s.e. = 0.3, P < 0.0002) (Supplementary Material Table S1). All other model with the ΔAICc < 2 contained factors ‘rodent’ ‘plant’, ‘fish’ and ‘game’. The RVI indicated very strong effect for the factor ‘rodent’ but weak effect towards factors ‘plant’, ‘fish’ and ‘game’.
The infection prevalence model (wi = 0.25) with helminths indicated that predators preying on reptiles had 5.5 times higher odds to be infected with Eucoleus spp./Trichuris spp. (β REPTILE = 1.7, s.e. = 0.7, P = 0.006) than predators that did not prey on reptiles. Similarly, preying on rodents increased significantly the odds (3 times) of being infected with Eucoleus spp./Trichuris spp. (β RODENT = 1.1, s.e. = 0.3, P = 0.00002) among predators compared to those who had not preyed on rodents. The RVI indicated very strong effect for factors ‘rodent’ and ‘reptile’ but weak effect towards other factors like ‘bird’ and ‘game’ (Supplementary Material Table S2).
The best infection intensity model (wi = 0.12) with Eucoleus spp./Trichuris spp. among predators revealed higher infection intensity for predators preying on game (β GAME = 0.6, s.e. = 0.3, P = 0.048). The RVI indicated strongest effect for factor ‘game’ but weaker on ‘reptile’ and ‘bird’ (Supplementary Material Table S3).
Surprisingly, the infection prevalence model (wi = 0.2) indicated that red foxes had up to 4 times higher odds to be infected with T. canis, if they consumed plant material (β PLANT = 1.4, s.e. = 0.6, P = 0.017). In the same model of sets (ΔAICc < 2), only 2 models included factor ‘rodent’ but without significant results. The RVI indicated moderate effect for factor ‘plant’ but weak effects towards factors ‘bird’, ‘insect’, ‘game’ and ‘rodent’ (Supplementary Material Table S4).
Among golden jackals, there were 2 equally good infection models (ΔAICc < 2). The best infection prevalence model (wi = 0.4) with helminths Eucoleus spp./Trichuris spp. revealed that preying on rodents increases significantly (15.5 times) the odds of being infected with Eucoleus spp./Trichuris spp. (β RODENT = 2.6, s.e. = 0.7, P = 0.0002) than not preying on rodents. The RVI indicated very strong effect for factor ‘rodent’ but a weak effect towards factor ‘game’ (Supplementary Material Table S5).
The best infection intensity model (wi = 0.56) with helminths Eucoleus spp./Trichuris spp. revealed 60 times higher infection intensity for golden jackals preying on rodents (β RODENT = 4.1, s.e. = 1.3, P = 0.0008) and 15.5 times higher infection intensity for golden jackals preying on game (β GAME = 2.6, s.e. = 1.2, P = 0.03). The RVI indicated very strong effect for factor ‘rodent’ but a weak effect towards factor ‘game’ (Supplementary Material Table S6).
The average distance between infected scat samples and privates was 578 metres. The minimum distance of an infected predator from a private household was 0 m (residing in the middle of a living land) and maximum distance was 2285 m. Moreover, 160 households were in the buffer zone of ⩽578 m in which the average distance of an infected scat to a private reached as far as 59 m (CL 48.2–70.8). There was no statistical significance (P < 0.05) between the infected predator groups (dog, red fox and golden jackal) in- (⩽578 m) or outside (0–2285 m) the buffer zones.
The overlap of zoonotic helminth fauna, calculated according to the Pianka's index, was highest between rural dogs and golden jackals (0.99) and somewhat lower for golden jackals and red foxes (0.84), as well as rural dogs and red foxes (0.84). The lowest overlap was determined between urban and rural dogs (0.29), followed by urban dogs and red foxes (0.30), and urban dogs and golden jackal (0.34), (Table 3; Supplementary Material Table S7).
Table 3. Helminth overlap between different canids (%)

Discussion
Molecular identification of mammalian predators
It is often problematic to morphologically differentiate mammalian predator scats and therefore using genetic analysis for correct identification of host is important to avoid false interpretations (Davison et al., Reference Davison, Birks, Brookes, Braithwaite and Messenger2002; Laurimaa et al., Reference Laurimaa, Davison, Süld, Plumer, Oja, Moks, Keis, Hindrikson, Kinkar, Laurimäe, Abner, Remm, Anijalg and Saarma2015b; Mumma et al., Reference Mumma, Adams, Zieminski, Fuller, Mahoney and Waits2016; Valdmann and Saarma, Reference Valdmann and Saarma2020; Tull et al., Reference Tull, Valdmann, Rannap, Kaasiku, Tammeleht and Saarma2022). The applied genetic method allowed to distinguish between various predator species, namely the red fox, dog and golden jackal (as the most abundantly represented species in this study), but also raccoon dog, pine marten, American mink (Neovison vison), Eurasian otter (Lutra lutra), grey wolf (Canis lupus) and cat. However, as it was not possible to discern individuals among mammal predators (DNA degradation was too high in scats to apply microsatellite analysis), it is not possible to say how many individuals were involved. Based on the relatively large study area and the abundance of predators (Kaasiku et al., Reference Kaasiku, Rannap and Männil2022), it can be ruled out that the collected scats belong to only a few individuals. Moreover, the aim was not to analyse the parasite prevalence of different individuals, but the general impact of infected mammalian wildlife contaminating the environment with parasite ova.
Zoonotic helminths of wild predators and rural dogs as a potential One Health concern
The study revealed a very high occurrence of helminth eggs (91.1%) in scats of mammalian predators in Western Estonia. Moreover, a considerable overlap of zoonotic helminth fauna between jackals and rural dogs (99%), as well as between foxes and rural dogs (84%) was found. This poses a potential risk to human health in rural areas, considering that nearly 160 privates were situated from an infected environmental scat on average up to 59 m. It is remarkable how similar the prevalence of different zoonotic helminths was between rural dogs and golden jackals, but also with red foxes (Fig. 2), whereas the comparison between urban and rural dogs, red foxes and jackals revealed much lower helminth overlap (Table 3). It is important to note that all recorded helminth taxa are potentially zoonotic (Table 1). Most taeniid species in dogs have zoonotic importance (e.g., Taenia spp., Echinococcus spp., Dibothriocephalus latus, Dipylidium caninum). Larvae of U. stenocephala can penetrate the skin and migrate in the top layer of skin, a condition called cutaneous larva migrans (CDC, 2022. The feline and canine bronchial capillarid Eucoleus aerophilus is causing pulmonary capillariasis also in humans and it has been suggested that this zoonotic parasite may be mis- and underdiagnosed in humans (Lalošević et al., Reference Lalošević, Stanojev-Jovanović, Lalošević, Pozio and Klem2008). Although the prevalence of T. canis and Toxascaris leonina was relatively low, both can cause toxocariasis in humans (Eslahi et al., Reference Eslahi, Badri, Khorshidi, Majidiani, Hooshmand, Hosseini, Taghipour, Foroutan, Pestehchian, Firoozeh and Riahi2020; Rostami et al., Reference Rostami, Riahi, Holland, Taghipour, Khalili-Fomeshi, Fakhri, Omrani, Hotez and Gasser2019). Human infections with T. leonina seem to be rare (Beaver and Bowman, Reference Beaver and Bowman1984; Rausch and Fay, Reference Rausch and Fay2011; Hoberg et al., Reference Hoberg, Galbreath, Cook, Kutz and Polley2012).
The high prevalence and overlap with zoonotic helminths of rural dogs with jackals and foxes raises a potential One Health concern in rural areas, since rural dogs provide a direct transmission link of zoonotic helminths from sympatric wildlife predators to dogs and from dogs to humans. The high overlap of parasite faunas among canids in rural areas suggests that rural dogs share roaming territories and food habits with jackals and foxes. As the current study provides evidence that rural areas are under continuous helminth contamination, relevant healthcare institutions should focus more on rural areas to diagnose helminth infections among companion animals (especially dogs) and in general human population. The potential One Health concern is supported by a previous study of Lassen et al. (Reference Lassen, Janson, Viltrop, Neare, Hütt, Golovljova, Tummeleht and Jokelainen2016), who tested antibodies against different zoonotic parasites (T. canis, Ascaris lumbricoides, Echinococcus spp., Taenia solium, Toxoplasma gondii, Trichinella spiralis) among different human subgroups in Estonia. It was concluded that considerable infection pressure occurs already in childhood and zoonotic parasitic infections are underdiagnosed or underreported in Estonia. The seroprevalence of T. canis, a zoonotic helminth recorded also in our study, was 12.1% and the seroprevalence was higher in animal caretakers than in the general population. Similarly, Remm and Remm (Reference Remm, Remm, Schærström, Jørgensen, Kistemann and Sivertun2014) found that dog owners had a significantly higher risk of being infected with Toxocara spp. Moreover, their study revealed another statistically significant relationship, namely that the prevalence was higher among those who do not wash their own garden fruit and vegetables (13.6%), compared to those who did (6.2%).
The overall infection prevalence models indicated that preying on diverse paratenic or intermediate hosts increases significantly the infection risk with helminths. If mammalian predators preyed on rodents or reptiles, the infection with Eucoleus spp./Trichuris spp. was 3–5.5 times higher. Helminths such as Eucoleus spp. and Trichuris spp. are geohelminths, maturing in the soil up to several weeks before becoming infective. Generally, mammalian predators become infected by ingesting E. aerophilus or T. vulpis eggs from the environment (attached to plant material or distributed in water and soil), but infection may also occur when consuming invertebrates (earthworms), Norway rats (Rattus norvegicus) or reptiles (Rataj et al., Reference Rataj, Lindtner-Knific, Vlahović, Mavri and Dovč2011; Rothenburger et al., Reference Rothenburger, Himsworth, Lejeune, Treuting and Leighton2014; Traversa et al., Reference Traversa, Frangipane di Regalbono, Di Cesare, La Torre, Drake and Pietrobelli2014; Wolf et al., Reference Wolf, Vrhovec, Failing, Rossier, Hermosilla and Pantchev2014). The high burden of helminth ova in scats of wild mammals and dogs, most of them situated near privates, indicates that rural environment poses a potential risk to human health. It is also worth noting that the parasite burden of dogs and cats in rural areas in Estonia is much higher compared to urban areas (Tull et al., Reference Tull, Moks, Laurimaa, Keis and Süld2020, Reference Tull, Valdmann, Rannap, Kaasiku, Tammeleht and Saarma2022).
Helminth coinfections
The overall coinfection rate of all mammalian predators was 61.7% (Supplementary Material Fig. S2) and the most dominant was the di-infection with Taeniidae and Eucoleus spp./Trichuris spp. (Supplementary Material Fig. S4). This can be explained by the highest infection rates with these parasite taxa (Table 1) and both taxa originate most likely from small rodents. The most coinfected host was the red fox (80.2%), while jackals and rural dogs had lower coinfection rates, 50.8 and 39.7%, respectively (Table 2; Supplementary Material Fig. S2). Di-infections dominated in foxes, jackals and dogs, followed by tri-infections. The rates of these 2 infection types were surprisingly similar in all 3 major predator species (Supplementary Material Fig. S3), which can be explained by relatively similar food habits. It is worth noting that fox scats were coinfected with up to 5 parasite taxa simultaneously. In a previous study in Estonia, foxes were found with up to 12 different parasite taxa and the highest proportion of foxes had 7 taxa (Laurimaa et al., Reference Laurimaa, Süld, Davison, Moks, Valdmann and Saarma2016b). However, this study was based on analysis of different internal organs (not scats), which can reveal also parasites that cannot be identified in scats. Thus, the absolute infection and coinfection rates in all mammal species in this study are likely to be higher. Nevertheless, analysis of scats is the most direct way to evaluate the environmental contamination by parasite ova. Moreover, this approach is non-invasive.
A relatively high similarity of helminth coinfections in jackals and dogs was found: di-infections with Taeniidae + U. stenocephala and Taeniidae + Eucoleus spp./Trichuris spp., but also tri-infection with Taeniidae + Eucoleus spp./Trichuris spp. + U. stenocephala (Table 2; Fig. 3). This is most likely due to sharing overlapping feeding areas and prey objects, facilitating analogous endoparasite transmission. Lanszki et al. (Reference Lanszki, Heltai and Szabó2006) have suggested analogous scenario for red foxes and jackals. However, there are also marked differences in coinfections (Table 2; Fig. 3). Foxes have about twice as high coinfection rate with Taeniidae and Eucoleus/Trichuris compared to jackals and dogs. Moreover, when foxes are virtually lacking di-infection with Taeniidae and U. stenocephala, it was found in nearly 40% of scats of jackals and dogs. This can be explained by high rate of simultaneous coinfection with Taeniidae and Eucoleus/Trichuris (Table 2; Fig. 3) by eating small rodents as the host for both helminth taxa. Small rodents are one of the most frequent prey of foxes. The high coinfection prevalence with zoonotic parasites and overlap in dogs, foxes and jackals is worryingly high, representing high risk for human health in rural areas.
Helminths of the red fox
A very high proportion of fox scats analysed were infected with parasitic helminths (92.4%; Table 1). The main helminth taxa found were Eucoleus spp./Trichuris spp., Taeniidae, U. stenocephala and T. canis. Somewhat similar results have been reported by Saeed et al. (Reference Saeed, Maddox-Hyttel, Monrad and Kapel2006) in Denmark and Bružinskaitė-Schmidhalter et al. (Reference Bružinskaitė-Schmidhalter, Šarkūnas, Malakauskas, Mathis, Torgerson and Deplazes2012) in Lithuania. In a previous study of fox parasitic helminths in Estonia that was based on examination of internal organs, 17 helminth taxa were found, including 10 zoonotic and the helminth prevalence was 100%, i.e., all analysed individuals were infected (Laurimaa et al., Reference Laurimaa, Süld, Davison, Moks, Valdmann and Saarma2016b). The highest infection rate was observed for trematode Alaria alata (90.7%), followed by nematodes Eucoleus aerophilus (87.6%) and U. stenocephala (84.3%), all zoonotic helminths. The prevalence of zoonotic taeniid Echinococcus multilocularis (that causes alveolar echinococcosis in humans) was 31.5%. Laurimaa et al. (Reference Laurimaa, Süld, Davison, Moks, Valdmann and Saarma2016b) found also tapeworms with zoonotic potential not registered in this study, namely Mesocestoides spp., Dibothriocephalus sp. and E. multilocularis. In the current study it was not possible to genetically distinguish between species of Taeniidae, most likely due to DNA degradation of ova by UV radiation in spring season. Since coastal areas offer a wide variety of intermediate or reservoir hosts that are essential for transmission of the above-mentioned taeniids, it is important to determine tapeworm species in further studies to estimate the zoonotic potential and proportions of these helminths in foxes, but also in other potential definitive hosts such as jackals, dogs and raccoon dogs.
The prevalence of T. canis in fox scats was rather low (6.0%; Table 1) compared to other countries, for example Germany (43.8%; Waindok et al., Reference Waindok, Raue, Grilo, Siebert and Strube2021), Lithuania (40.5%; Bružinskaitė-Schmidhalter et al., Reference Bružinskaitė-Schmidhalter, Šarkūnas, Malakauskas, Mathis, Torgerson and Deplazes2012) or Denmark (59.4%; Saeed et al., Reference Saeed, Maddox-Hyttel, Monrad and Kapel2006). The previous fox study in Estonia by Laurimaa et al. (Reference Laurimaa, Süld, Davison, Moks, Valdmann and Saarma2016b) found T. canis in 29.6% of samples. The difference could be due to lower environmental contamination of T. canis in the coastal area. However, there could be another explanation. In Estonia, the food of red foxes includes rodents, mainly in autumn when the abundance of small rodents is the highest due to ripened crops on the fields. During spring, red foxes switch from rodents to food sources like birds, reptiles, hares and amphibians, but also fish in coastal areas (Kauhala et al., Reference Kauhala, Laukkanen and Rége1998; Laurimaa et al., Reference Laurimaa, Süld, Davison, Moks, Valdmann and Saarma2016b; Kaasiku et al., Reference Kaasiku, Rannap and Männil2022). The change in the food composition in spring may also reflect a shift in endoparasite species. For example, the relatively low prevalence of T. canis in this study could be a result of switching from rodents to other prey items, whereas the infection prevalence of taeniids and Eucoleus spp./Trichuris spp. is relatively high due to high fish, reptile and amphibian abundance.
It is worth noting that the infection prevalence model indicated a relation between consumed plant material and infection with T. canis, namely the infection with T. canis increased when the diet consisted more of plant material. One explanation could be the self-medication with plants to lower the burden of helminths. Laurimaa et al. (Reference Laurimaa, Moks, Soe, Valdmann and Saarma2016a) has also suggested a self-medicating behaviour, but for raccoon dog. They found significantly more consumed plants among raccoon dogs with higher helminth prevalence.
Helminths of the golden jackal
The first presence of golden jackals in Estonia was reported in 2013 and it has been suggested that the species migrated naturally to Estonia, having the genetic origin in the Caucasian population (Rutkowski et al., Reference Rutkowski, Krofel, Giannatos, Ćirović, Männil, Volokh, Lanszki, Heltai, Szabó, Banea, Yavruyan, Hayrapetyan, Kopaliani, Miliou, Tryfonopoulos, Lymberakis, Penezić, Pakeltytė, Suchecka and Bogdanowicz2015). As a result of such migration, jackals as a definitive host for a number of helminths can increase transmission risk of parasitic species, including those of zoonotic potential (i.e., Echinococcus spp., T. canis) (Ilić et al., Reference Ilić, Becskei, Petrović, Polaček, Ristić, Milić, Stepanović, Radisavljević and Dimitrijević2016; Lalošević et al., Reference Lalošević, Lalošević, Simin, Miljević, Čabrilo and Čabrilo2016; Gherman and Mihalca, Reference Gherman and Mihalca2017). In Estonia, Jõgisalu et al. (Reference Jõgisalu, Põldmaa and Männil2019) have found, based on analysis of internal organs, that the most prevalent endoparasites of jackals are A. alata and U. stenocephala. In the current study, the golden jackals were mostly infected with Taeniidae and U. stenocephala, while it was lower for Eucoleus spp./Trichuris spp., T. canis and T. leonina. The infection prevalence and intensity of helminths is largely impacted by preying on rodents. However, coastal areas provide during the spring season not as many rodents as in autumn. Moreover, in springtime a myriad of intermediate and paratenic hosts are available, including amphibians, reptiles, fish and insects, capable of infecting jackals with a wide diversity of endoparasites. Gherman and Mihalca (Reference Gherman and Mihalca2017) have reported a total of 194 parasite species distributed by jackals, a majority of these parasites are shared with dogs or cats. Due to the relatively high infection prevalence with Taeniidae and U. stenocephala, the importance of golden jackal as a definitive host for zoonotic Echinococcus spp., hookworms and T. canis should be highlighted because of the hazards to public health, and further investigations should focus more on diagnosing these zoonotic parasites at a species level. This requires relatively fresh scats and a more refined genetic methodology.
Helminths of other mammal species
The other mammalian predators infected with helminths were the pine marten, raccoon dog, mink, wolf, cat and otter (Table 1). The American mink and raccoon dog are non-native mammal species in Estonia (similar to the golden jackal), capable of transmitting endoparasites. Raccoon dogs were predominantly infected with Taeniidae and Eucoleus/Trichuris (both 71.4%). Former studies in Estonia, based on examination of internal organs, have shown that the overall prevalence of cestodes among raccoon dogs was 30.5% (Laurimaa et al., Reference Laurimaa, Süld, Davison, Moks, Valdmann and Saarma2016b), more than 2 times lower compared to the current study, which could be due to various reasons (difference in sampling locations, time, etc.). Regarding Taeniidae, raccoon dogs can act also as a vector for the tapeworm E. multilocularis (1.6%; Laurimaa et al., Reference Laurimaa, Süld, Moks, Valdmann, Umhang, Knapp and Saarma2015c), albeit to a much lower extent compared to the red fox (31.5%; Laurimaa et al., Reference Laurimaa, Moks, Soe, Valdmann and Saarma2016a).
Interestingly, according to our data, mink and pine marten distribute Toxocara spp. ova, which may suggest that they can act as definitive hosts for Toxocara spp., although Kołodziej-Sobocińska et al. (Reference Kołodziej-Sobocińska, Dvorožňáková, Hurníková, Reiterová and Zalewski2020) have indicated that American mink serves rather as a paratenic hosts for T. canis in the wild. It is possible that eggs of unembryonated T. canis were swallowed from the contaminated environment and passed via scats again to the environment. Nevertheless, if mink and marten serve as a paratenic host for T. canis, this zoonotic parasite can spread to definitive hosts such as red foxes, golden jackals or dogs. In Poland, Borecka et al. (Reference Borecka, Gawor and Zieba2013) reported that pine martens were infected with zoonotic T. cati in the Tatra National Park. The single cat sample in this investigation was also infected with T. cati, whereas a recent study by Tull et al. (Reference Tull, Moks and Saarma2021) found that 45.6% of analysed rural cat samples (among shelter cats) were infected with this parasite.
To conclude, the study revealed the role of wild mammals, especially the red fox and golden jackal, in contaminating rural environment with eggs of zoonotic helminths. The importance of the golden jackal, a new mammal species in Estonia and several other European countries, as a vector of zoonotic helminths and a potential hazard to public health, should be emphasized. Considering that habitats of wild predators such as the red fox and golden jackal overlap to some extent with free-ranging dogs, endoparasite transmission may occur, being potentially also hazardous to humans who interact with infected dogs. Moreover, humans can also get infection from the contaminated environment. Based on the results, it should be recommended that free-ranging of dogs in rural areas is limited as much as possible and that dogs are regularly treated with appropriate anthelmintics. Moreover, further parasitological investigations of jackals and other wild mammals have to be conducted to reveal the full spectrum of zoonotic helminths at a species level. It is worth noting that human infection with zoonotic parasites is likely underdiagnosed and the zoonotic potential of many helminth parasites is therefore not fully revealed. As many parasitic diseases of wildlife are a One Health concern, a coordinated work between healthcare institutions, veterinarians and parasitologists are required to diagnose helminth infections among wild mammals, companion animals and in general human population in Estonia.
Supplementary material
The supplementary material for this article can be found at https://doi.org/10.1017/S0031182022001032
Data availability
Data is available upon request.
Acknowledgements
The authors thank Agnes Kapanen and Kristjan Põldma for their valuable help.
Author's contributions
AT, US and HV conceived and designed the study. AT, HV, ET, TK, RR and US collected samples. AT performed statistical analyses. US and ET performed genetical analysis. AT wrote the first draft. US, HV, ET, TK and RR contributed to writing and editing.
Financial support
This work was supported by research funding (grant PRG1209) from the Estonian Ministry of Education and Research; Environmental Investment Centre (project 14338).
Conflict of interests
None.
Ethical standards
There is no ethical issue involved in this study.