Introduction
Leishmania are parasitic protozoa, which are transmitted by the bite of a female phlebotomine sand fly. Depending on the species of parasite, Leishmania can cause a spectrum of diseases, ranging from painful and disfiguring skin lesions (cutaneous leishmaniasis, CL, and mucocutaneous leishmaniasis) to fatal visceral infection of the spleen and liver (visceral leishmaniasis). In the mammalian host, Leishmania parasites reside within professional phagocytic cells of the monocyte-macrophage lineage as amastigote forms. When they are taken up by a sand fly they multiply and undergo a series of transformations as various flagellated promastigote forms; culminating in the differentiation to a mammal-infective stage, the metacyclic promastigote (reviewed in Bates, Reference Bates1997).
It is becoming increasingly clear that transmission by sand fly bite has considerable bearing on the infection and is vastly more efficient than needle-based infection of parasites alone (reviewed by Serafim et al., Reference Serafim, Dey, Nakhasi, Valunzuela, Kamhawi, Wikel, Aksoy and Dimopoulos2017). From a sand fly bite metacyclic promastigotes are delivered into the skin of a host in the context of a unique inflammatory environment (Belkaid et al., Reference Belkaid, Kamhawi, Modi, Valenzuela, Noben-Trauth, Rowton, Ribeiro and Sacks1998; Kamhawi et al., Reference Kamhawi, Belkaid, Modi, Rowton and Sacks2000). This is generated by the complex combination of parasite dose (Kimblin et al., Reference Kimblin, Peters, Debrabant, Secundino, Egen, Lawyer, Fay, Kamhawi and Sacks2008), dose composition (i.e. proportion of metacyclic and non-metacyclic promastigotes) (Giraud et al., Reference Giraud, Martin, Yakob and Rogers2019), damage caused by the bite (Giraud et al., Reference Giraud, Lestinova, Derrick, Martin, Dillon, Volf, Műller, Bates and Rogers2018), sand fly-associated bacteria (Dey et al., Reference Dey, Joshi, Oliveira, Pereira, Guimarães-Costa, Serafim, de Castro, Coutinho-Abreu, Bhattacharya, Townsend, Aslan, Perkins, Karmakar, Ismail, Karetnick, Meneses, Duncan, Nakhasi, Valenzuela and Kamhawi2018; Giraud et al., Reference Giraud, Martin, Yakob and Rogers2019) and the introduction of the vector saliva and other parasite-released molecules (Titus and Ribeiro, Reference Titus and Ribeiro1988; Belkaid et al., Reference Belkaid, Kamhawi, Modi, Valenzuela, Noben-Trauth, Rowton, Ribeiro and Sacks1998; Rogers et al., Reference Rogers, Ilg, Nikolaev, Ferguson and Bates2004; Volfova et al., Reference Volfova, Hostomska, Cerny, Votypka and Volf2008; Atayde et al., Reference Atayde, Aslan, Townsend, Hassani, Kamhawi and Olivier2015).
In the sand fly, Leishmania secrete high molecular weight proteophosphoglycans [filamentous proteophosphoglycan, fPPG; secreted acid phosphatase and a number of poorly defined lower molecular weight proteophosphoglycans (Montgomery et al., Reference Montgomery, Curtis and Handman2002)], which condense in the limited volume of the sand fly anterior midgut to form a gel-like obstruction, termed the Promastigote Secretory Gel (PSG) (Ilg et al., Reference Ilg, Stierhof, Craik, Simpson, Handman and Bacic1996; Stierhof et al., Reference Stierhof, Bates, Jacobson, Rogers, Schlein, Handman and Ilg1999; Rogers et al., Reference Rogers, Chance and Bates2002, Reference Rogers, Ilg, Nikolaev, Ferguson and Bates2004). PSG, has been noted for many natural and experimental Leishmania-sand fly combinations (reviewed by Rogers, Reference Rogers2012) and the blocked fly phenotype has been hypothesized to contribute to the transmission of leishmaniasis by encouraging the regurgitation of parasites (Shortt and Swaminath, Reference Shortt and Swaminath1928). The role of PSG was first demonstrated in 2004 using the experimental, yet highly transmissible, combination of Leishmania mexicana and Lutzomyia longipalpis (Rogers et al., Reference Rogers, Ilg, Nikolaev, Ferguson and Bates2004). Blocked sand flies were shown to regurgitate an average of 1000 parasites and a proportion of the PSG during transmission. Co-inoculation of this dose of L. mexicana metacyclic promastigotes with PSG promoted the survival and growth of amastigotes, which strongly aggravated skin infections in mice (Rogers et al., Reference Rogers, Ilg, Nikolaev, Ferguson and Bates2004, Reference Rogers, Kropf, Choi, Dillon, Podinovskaia, Bates and Müller2009). Using this sand fly in a natural parasite-vector combination, PSG was also shown to exacerbate cutaneous and visceral infection of Leishmania infantum in mice (Rogers et al., Reference Rogers, Corware, Müller and Bates2010).
As it appears that PSG can manipulate both the sand fly and mammalian hosts for efficient transmission, we aimed to see if this was relevant to other natural models of leishmaniasis. Therefore, we recovered, characterized and tested the infection-modulating properties of PSG from a variety of Leishmania-sand fly combinations responsible for CL in the field.
Materials and methods
Infection of sand flies
Leishmania major strain FV39 clone 5 (RHO/SU/59/P) and Leishmania tropica from Sanilurfa, Turkey (MHOM/1999/TR/SU23) were originally isolated from human cutaneous lesions and have been routinely passaged through mice. Promastigotes of these parasites were cultured as previously described (Rogers et al., Reference Rogers, Chance and Bates2002), resulting in an enriched population of metacyclic promastigotes as determined by morphological analysis of Giemsa-stained parasites. Briefly, 1 × 106 BALB/c lesion amastigotes per mL were transformed into promastigotes in M199 culture medium (Hank's modified with L-glutamine) supplemented with 10% heat-inactivated foetal calf serum (FCS, v/v), 1 × Basal Medium Eagle vitamins (v/v), 1% penicillin-streptomycin (v/v), 400 mm sodium bicarbonate (all Sigma UK), pH 7.2, at 26 °C for 3 days. Following this, promastigotes were sub-passaged to 5 × 105 mL in fresh M199 promastigote medium and allowed to undergo metacyclogenesis by 10–13 days post-culture. The cultures were assessed daily to select those with the highest proportion of metacyclic promastigotes. Using this culture method typically yielded 80–85% metacyclic promastigotes. Five-day-old female sand flies were infected with amastigotes through an artificial membrane feeding system at 2 × 106 amastigotes per mL in fresh rabbit blood and maintained in a 12 h light:dark cycle at 28 °C, 80% relative humidity with free access to 12.5% sucrose.
Preparation of vector saliva and parasite PSG
Sand fly saliva was obtained from 6–8 day-old female uninfected flies by piercing individual salivary glands in ice-cold PBS, followed by centrifugation at 3000 rpm for 10 min to remove any salivary gland epithelia or debris (Rogers et al., Reference Rogers, Ilg, Nikolaev, Ferguson and Bates2004). Pools of 10 flies were processed at a time to prevent degradation of the saliva and a minimum of 60 flies processed to supply saliva for the co-infections. For L. mexicana and L. infantum we have observed that PSG accumulates within the Lu. longipalpis midgut from day 7 onwards (Rogers and Bates, Reference Rogers and Bates2007). Therefore, PSG plugs were isolated from the midguts of day 10–13 infected flies in PBS to ensure that all parasites had reached maturity i.e. completed metacyclogenesis. PSG was obtained by dissecting the gut along its length and removing the PSG plug manually using 30 gauge insulin syringe needles. Each PSG plug was transferred to a tube in 5 µL of the dissection medium. Parasites were removed by centrifuging twice at 3000 rpm for 10 min followed by two spins at 7,000 rpm for 10 min. The average yield of PSG for each sand fly-Leishmania combination used is presented in Table 1. Saliva and PSG were filter-sterilized (0.2 µm pore diameter) and stored in aliquots at −40 °C until use. The protein content of PSG and saliva was determined using the BCA method and standardized to 1 µg per injection. As a negative control for the Western Blot, the luminal content of 30 age-matched, bloodfed uninfected flies was sampled by dissecting each gut in the same way as an infected fly then sucking 5 µL of dissection medium near the incision. These were pooled and processed as above.
Table 1. The average amount of PSG recovered from sand flies used in this study

P. papatasi and P. duboscqi were infected with L. major and PSG extracted from day 12–13 p.i. P. arabicus was infected with L. tropica and PSG was extracted 12–13 p.i. Lu. longipalpis was infected with L. major and L. tropica and the PSG extracted 8 days p.i. For reference, Lu. longipalpis infected with L. mexicana yielded an average 0.88 ± 0.14 µg protein per infected fly at 8–9 days p.i. nd, not done.
Western blotting
PSG was separated by SDS PAGE using a 10% acrylamide/bis-acrylamide gel (Sigma) with an extended stacking gel (4% acrylamide/bis-acrylamide) as previously described (Rogers et al., Reference Rogers, Ilg, Nikolaev, Ferguson and Bates2004). Gels were transferred to activated nitrocellulose and probed with the LT15 (1:3000) monoclonal antibody against phosphoglycan disaccharide repeats [PO4-6Galβ1-4Manα1-]x (Ilg et al., Reference Ilg, Stierhof, Craik, Simpson, Handman and Bacic1996). Blots were developed using a biotinylated anti-mouse IgG secondary antibody and HRP immunoprecipitation (ABC Elite, VIP, Vector Labs).
Infection of mice
Anaesthetized, 20 g, 6–8 week old female BALB/c mice were infected either by the intradermal injection of 1 × 103 L. major into the dorsal surface of the footpad, or 1 × 106 L. tropica metacyclic promastigotes into the shaved rump, in a total volume of 20 µL. Under restraint, Vernier callipers were used to measure thickness of infected and uninfected footpads of mice to calculate lesion swelling (L. major), or the average diameter of rump lesions to calculate their area (L. tropica). At the end of experiments, mice were euthanized allowing parasite burdens to be determined. Burdens were determined by direct counting of amastigotes from footpad homogenates. All procedures involving animals were approved by a local Animal Welfare Committee and performed in accordance with the United Kingdom Government (Home Office), Czech Ministry of Health and EC regulations.
Results
PSG from L. major, L. tropica and L. mexicana bear similar Gal(β1-4)Man(α1)-PO4 containing glycans
The backbone of many O/N-linked Leishmania oligosaccharides contains phosphate-linked galactose-mannose disaccharide (Gal-Man-P) repeats (Ilg, Reference Ilg2000). Using Western blotting, the PSG from L. major and L. tropica extracted from the experimental vector Lu. longipalpis, were probed for Gal(β1-4)Man(α1)-PO4 repeating epitopes (Fig. 1). The absence of a reaction with the contents of uninfected midguts confirmed the specificity of the antibody for parasite glycans. All infected flies yielded PSGs containing a very high molecular weight smear retained in the 4% stacking gel that is likely to be the fPPG fraction. This gives the PSG much of its structure as a 3D matrix (Stierhoff et al., Reference Stierhof, Bates, Jacobson, Rogers, Schlein, Handman and Ilg1999) and confers the disease-exacerbating properties of PSG for L. mexicana (Rogers et al., Reference Rogers, Ilg, Nikolaev, Ferguson and Bates2004). In the experimental vector, Lu. longipalpis, the amount of fPPG was similar for L. mexicana and L. major, which were greater than the amount of fPPG produced by L. tropica. Notably, this is an experimental model so the precise amounts of fPPG in PSG from natural vectors may vary lipophosphoglycan (LPG) also contains Gal-Man-P repeats, however, based on the expected molecular weight (30–50 kDa for non-metacyclic and 70–90 kDa for metacyclic promastigotes), LPG was not detected in any of the PSG preparations.
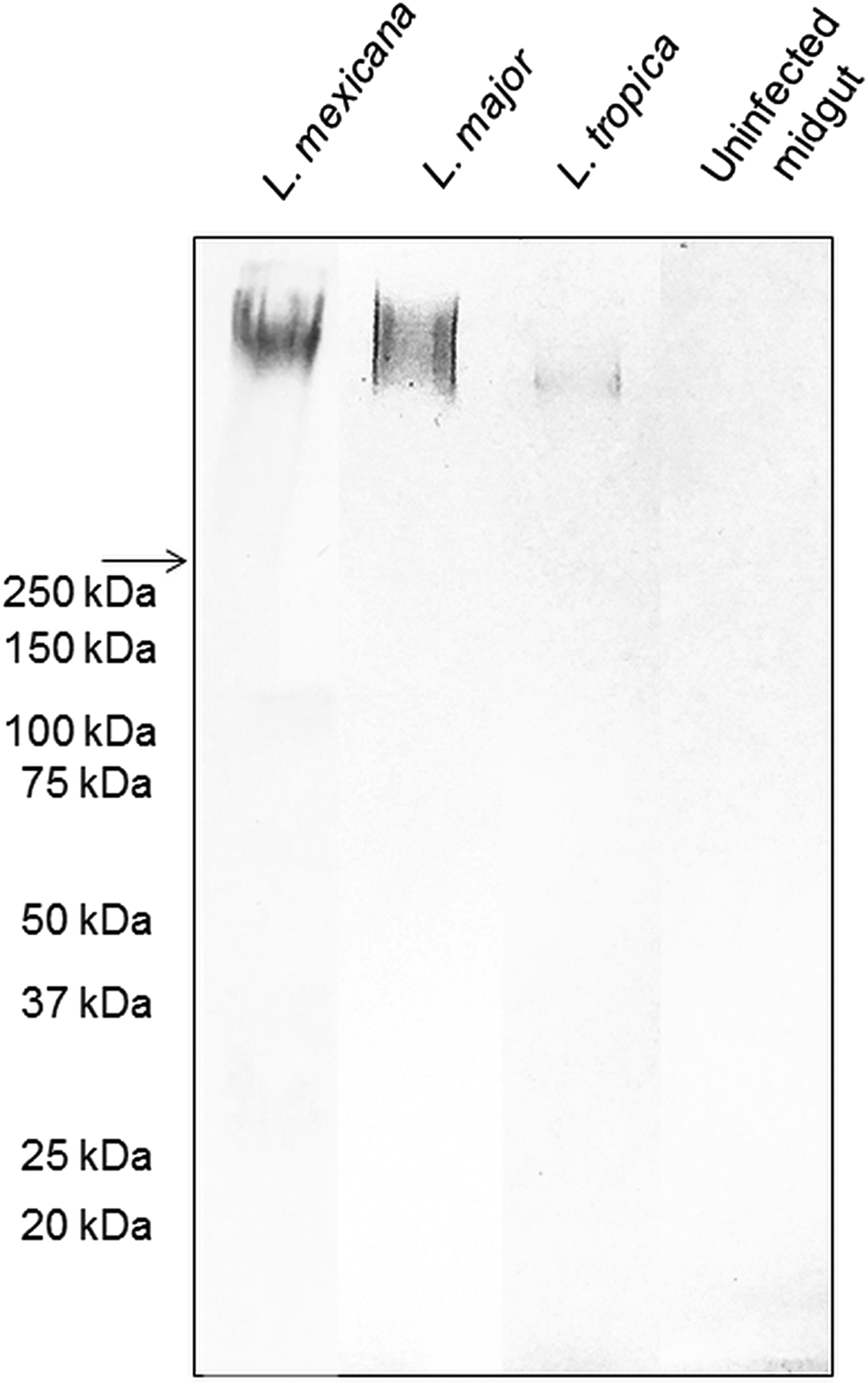
Fig. 1. The composition of Leishmania glycans within PSG from the permissive vector Lu. longipalpis. Western Blot analysis of L. major, L. tropica and L. mexicana PSG probed with LT15, a mAb which recognizes the Gal-P-Man disaccharide backbone of all Leishmania phosphoglycans (Ilg et al., Reference Ilg, Stierhof, Craik, Simpson, Handman and Bacic1996). Each lane represents the equivalent of one sand fly's PSG extracted from mature infections (L. major and L. tropica, day 12–13 p.i.; L. mexicana day 8 p.i.). The last lane represents the luminal content of one uninfected sand fly midgut probed with LT15. Arrow indicates a junction between stacking and resolving gel. MWM, molecular weight markers.
L. major PSG from its natural vectors, Phlebotomus (Phlebotomus) papatasi and Phlebotomus (Phlebotomus) duboscqi, exacerbates cutaneous infections in vivo
Inclusion of sterile L. major PSG from the natural vectors, P. papatasi or P. duboscqi promoted L. major infection in the skin of BALB/c mice (Fig. 2). The presence of L. major PSG during infection resulted in more mice developing a lesion earlier than the controls [10 vs 25 days for infections with L. major PSG from P. papatasi (Fig. 2A and C) and 14 vs 28 days for infections with L. major PSG from P. duboscqi (Fig. 2B and D)]. These infections grew faster than the controls, resulting in significantly larger lesions, which persisted (Fig. 2C and D). The final parasite burdens for these lesions showed that PSG benefited L. major growth, resulting in an average 30-fold higher number amastigotes per lesion compared to saline with metacyclics alone [10-fold for lesions with L. major PSG from P. papatasi (Fig. 2E), and 50-fold for L. major lesions co-inoculated with PSG from P. duboscqi (Fig. 2F)]. Similarly, the saliva from these two natural sand fly vectors significantly exacerbated infection with L. major, resulting in an average 45- to 60-fold higher amastigote burden at the end of the experiment.

Fig. 2. L. major infection in mice is exacerbated by the presence of PSG and saliva from natural vectors. L. major PSG was obtained from infected P. papatasi and P. duboscqi sand flies and saliva collected from uninfected flies (P. papatasi: A, C and E; P. duboscqi: B, D and F). One thousand L. major metacyclic promastigotes were injected i.d. into the footpads of BALB/c mice without (open circles) or with 1 µg sterile PSG (closed squares) or 1 µg sterile saliva (open triangles). (A and B) Proportion of mice presenting with cutaneous lesions over the course of study. (C and D) Footpad lesion evolution showing average lesion thickness + s.e.m. (E and F) Final parasite burdens of lesions from footpad homogenates. Each point represents individual mice and bars represent the average value per group. Infections performed in triplicate and representative data is shown, n = 4–5 mice per group. *: P < 0.05; **: P < 0.005 by Mann Whitney unpaired two-tailed t-test.
L. tropica PSG from its natural vector, Phlebotomus (Adlerius) arabicus, exacerbates cutaneous infection in vivo
The infections with L. tropica significantly benefited from the presence of PSG, recovered from its natural vector, P. arabicus (Fig. 3). Even at a high dose of metacyclic promastigotes (1 × 106), infection of mice with L. tropica in the absence of vector components took 30–33 weeks to generate cutaneous lesions (Fig. 3A and B). The presence of PSG cut this time by 60%, allowing the detection of lesions on the rumps of BALB/c mice by 13 weeks post-infection. The addition of PSG or saliva resulted in lesions that were chronic and non-healing, compared to the control infections that appeared to plateau in size after 43 weeks post-infection (Fig. 3B). Lesions from L. tropica-PSG co-infections grew significantly faster than the controls or those co-inoculated with P. arabicus saliva, resulting in an average 22-fold higher final parasite burdens compared to the controls. By comparison, co-infection with P. arabicus saliva resulted in an average 16-fold higher amastigote burden at the end of the experiment (Fig. 3B and C). Similarly, the proportion of mice, which developed lesions were equally high in the PSG and saliva co-infection groups (PSG: 83%, saliva: 83%, control: 50%) (Fig. 3A).

Fig. 3. L. tropica infection in mice is exacerbated by PSG and saliva from a natural vector. L. tropica PSG was obtained from infected P. arabicus sand flies and saliva collected from uninfected flies. One million L. tropica metacyclic promastigotes were injected i.d. into the rumps of BALB/c mice without (open circles) or with 1 µg sterile PSG (closed squares) or 1 µg sterile saliva (open triangles). (A) Proportion of mice presenting with cutaneous lesions over the course of study. (B) Rump lesion evolution showing total lesion size. (C) Final parasite burdens of lesions from homogenates. Each point represents individual mice. Infections performed in duplicate and representative data is shown, n = 6 mice per group. *: P < 0.05 by Mann Whitney unpaired two-tailed t-test.
L. major and L. tropica PSG from the experimental vector, Lu. longipalpis, exacerbates cutaneous infection in vivo
The PSG extracted from the experimental vector, Lu. longipalpis promoted L. major and L. tropica infection in BALB/c mice (Fig. 4). Similar to the infections using PSG from their natural vectors, the inclusion of Lu. longipalpis-derived PSG in the inoculum contracted the time to the first appearance of cutaneous lesions by 25 days and 21 weeks for L. major and L. tropica, respectively, compared to 35 days and 33 weeks for infections with metacyclic promastigotes alone (Fig. 4A and B). The resultant lesions also displayed accelerated growth (Fig. 4C and D) and higher final parasite burdens (Fig. 4E and F, L. major: 11-fold; L. tropica: 35-fold more amastigotes recovered from lesions), similar to those observed from the co-inoculation of PSG from their natural vectors. Saliva from Lu. longipalpis promoted cutaneous infection and pathology for L. major resulting in a 43-fold higher amastigote burden at the end of the experiment. For L. tropica, however, Lu. longipalpis saliva appeared to promote lesion size but had little influence over the growth of these parasites in skin (Fig. 4E and F). Collectively, these results show that PSG from Lu. longipalpis can exacerbate L. major and L. tropica to a similar extent as the material collected from their natural vectors.

Fig. 4. L. major and L. tropica infections in mice are exacerbated by PSG and saliva from the experimental vector, Lu. Longipalpis (L. major: A, C and E; L. tropica: B, D and F). L. tropica and L. major PSG was obtained from infected Lu. longipalpis sand flies and saliva collected from uninfected flies. One thousand L. major metacyclic promastigotes or one million L. tropica metacyclic promastigotes were injected i.d. into the footpads or rumps of BALB/c mice without (open circles) or with 1 µg sterile PSG (closed squares) or with 1 µg sterile saliva (open triangles). (A and B) Proportion of mice presenting with cutaneous lesions over the course of study. (C and D) Lesion evolution showing average lesion thickness + s.e.m or total lesion size. (E and F) Final parasite burdens of lesions from tissue homogenates. Each point represents individual mice and bars represent the average value per group. Representative data is shown of duplicate experiments, n = 6 mice per group. *: P < 0.05; **: P < 0.005 by Mann Whitney unpaired two-tailed t-test.
Discussion
This study demonstrates for the first time the infection-enhancing effect of PSG of two related CL Leishmania species from natural and unnatural phlebotomine sand fly vectors.
L. major is the causative agent of zoonotic cutaneous leishmaniasis, endemic in the Middle East and Northern Africa (Maroli et al., Reference Maroli, Feliciangeli, Bichaud, Charrel and Gradoni2013). Its natural vectors include P. papatasi and P. duboscqi (Dvorak et al., Reference Dvorak, Shaw, Volf, Bruschi and Gradoni2018). Cutaneous infections in non-healing strains of mice, such as BALB/c, typically result in strong Th2 polarisation, driven largely by IL-4, allowing uncontrolled parasite growth (Noben-Trauth et al., Reference Noben-Trauth, Kropf and Müller1996; Belkaid et al., Reference Belkaid, Kamhawi, Modi, Valenzuela, Noben-Trauth, Rowton, Ribeiro and Sacks1998). Vector saliva benefits L. major infection in naïve hosts by biasing the local immune environment towards a Th2 phenotype, through increased expression of IL-4 and IL-10 (Belkaid et al., Reference Belkaid, Kamhawi, Modi, Valenzuela, Noben-Trauth, Rowton, Ribeiro and Sacks1998). This immunomodulation promotes the initial infection and allows L. major to persist in skin, even after lesion resolution. The persistence of low numbers of L. major can establish concomitant immunity to reinfection and establish the host as a long-term reservoir of infection (Kimblin et al., Reference Kimblin, Peters, Debrabant, Secundino, Egen, Lawyer, Fay, Kamhawi and Sacks2008).
L. tropica overlaps in much of its range with L. major and is the causative agent of anthroponotic CL, endemic in the Middle East, Mediterranean basin, Central Asia, and East Africa. The primary vector of L. tropica is Phlebotomus (Paraphlebotomus) sergenti (Kamhawi et al., Reference Kamhawi, Belkaid, Modi, Rowton and Sacks2000; Myskova et al., Reference Myskova, Svobodova, Beverley and Volf2007; Volf and Myskova, Reference Volf and Myskova2007), although other sand fly species have been shown to transmit, including P. arabicus in northern Israel (Jacobson et al., Reference Jacobson, Eisenberger, Svobodová, Baneth, Sztern, Carvalho, Nasereddin, El Fari, Shalom, Volf, Votypka, Dedet, Pratlong, Schonian, Schnur, Jaffe and Warburg2003; Svobodová et al., Reference Svobodová, Volf and Votýpka2006a, Reference Svobodova, Votypka, Peckova, Dvorak, Nasereddin, Baneth, Sztern, Kravchenko, Orr, Meir, Schnur, Volf and Warburg2006b) and Ethiopia (Gebre-Michael et al., Reference Gebre-Michael, Balkew, Ali, Ludovisi and Gramiccia2004). L. tropica is responsible for cutaneous lesions which are typically drier, longer lasting, require longer to heal and are often more difficult to treat compared to L. major; resulting in considerable scarring (Klaus and Frankenburg, Reference Klaus and Frankenburg1999). In mouse models, the persistence of L. tropica is also Th2-dependent, requiring manipulation of IL-10 and TGF-β signalling (Anderson et al., Reference Anderson, Lira, Kamhawi, Belkaid, Wynn and Sacks2008; Kobets et al., Reference Kobets, Havelková, Grekov, Volkova, Vojtíšková, Slapničková, Kurey, Sohrabi, Svobodová, Demant and Lipoldová2012). Similar to L. major, chronic lesions of L. tropica in mice harbour sufficient numbers of parasites to be transmitted to their natural vector (Anderson et al., Reference Anderson, Lira, Kamhawi, Belkaid, Wynn and Sacks2008).
Components of the infectious sand fly bite greatly influence the course of leishmaniasis in mammalian hosts (Serafim et al., Reference Serafim, Dey, Nakhasi, Valunzuela, Kamhawi, Wikel, Aksoy and Dimopoulos2017). This was first identified over thirty years ago, when it was found that the presence of P. papatasi sand fly saliva during L. major infection greatly promoted cutaneous lesion evolution and parasite growth (Titus and Ribeiro, Reference Titus and Ribeiro1988). However, sand fly saliva alone does not fully explain the efficiency or unique immune profile of Leishmania transmission (Serafim et al., Reference Serafim, Dey, Nakhasi, Valunzuela, Kamhawi, Wikel, Aksoy and Dimopoulos2017). Other factors, including the biting behaviour of the infected sand fly, the low dose delivered by bite, the wound damage associated with the sand fly bite and the presence of other parasite factors generated within the sand fly prior to transmission are likely to have a bearing on natural Leishmania infection. Understanding the contribution of these factors will allow us to generate more physiologically relevant models of Leishmania infection in the future. In this regard, we extend our previous findings with L. mexicana and L. infantum and show that the glycan-rich PSG from L. major and L. tropica, extracted from their natural vectors, can potently accelerate and exacerbate cutaneous infection in mice.
Using the experimental L. mexicana–Lu. longipalpis CL model of transmission we have previously shown that PSG promotes cutaneous infection by accelerating wound healing in skin of the mammalian host (Giraud et al., Reference Giraud, Lestinova, Derrick, Martin, Dillon, Volf, Műller, Bates and Rogers2018). PSG recruited host macrophages to the site of infection and manipulated their physiology by upregulating the expression of arginase 1, an enzyme responsible for polyamine production and a signature of macrophage alternative (M2) activation (Rath et al., Reference Rath, Müller, Kropf, Closs and Munder2014). This effect appears to be under the control of insulin growth factor 1 pathway, as part of the wound healing process, resulting in the enhanced survival of Leishmania and their growth inside macrophages (Giraud et al., Reference Giraud, Lestinova, Derrick, Martin, Dillon, Volf, Műller, Bates and Rogers2018). Using this parasite-vector model, we have also shown that the PSG-blockage impacts on the blood-feeding ability of the sand fly and promotes transmission by manipulating the sand fly feeding behaviour (Rogers and Bates, Reference Rogers and Bates2007). It is likely that both L. major and L. tropica would benefit from the ability of PSG to promote M2 macrophage activation, as polyamines are essential for their intracellular growth in mice (Green et al., Reference Green, Meltzer, Hibbs and Nacy1990; Badirzadeh et al., Reference Badirzadeh, Taheri, Taslimi, Abdossamadi, Heidari-Kharaji, Gholami, Sedaghat, Niyyat and Rafati2017). Moreover, arginase 1 activity is closely associated with acute lesions of L. major and L. tropica in humans (Mortazavi et al., Reference Mortazavi, Sadeghipour, Taslimi, Habibzadeh, Zali, Zahedifard, Rahmati, Kamyab, Ghandi, Zamanian, Reza Tohidinik, Muller, Kropf and Rafati2016).
Compared to L. major, L. tropica is a poorly studied parasite because of a lack of a reliable model of infection. In rats, L. tropica does not produce lesions despite surviving in the skin and being able to infect naïve sand flies for more than a year post-infection (Svobodová et al., Reference Svobodová, Votýpka, Nicolas and Volf2003). In mice, although lesions can be generated, they are notoriously difficult and slow to establish. Lira et al., showed that experimental infections were possible in mice, requiring large doses (106) of highly enriched metacyclic promastigotes (Lira et al., Reference Lira, Méndez, Carrera, Jaffe, Neva and Sacks1998). We extend their findings by revealing that both vector saliva and PSG from infected vectors can substantially accelerate their model of cutaneous L. tropica infection in mice, thereby highlighting the probable role of vector-derived products for the establishment of L. tropica infection and improving the current model of infection. In the future, it would be worth testing the exacerbatory role of PSG on L. tropica infection in the ear dermis, as this site of infection has been shown to produce reliable infections with lower numbers (103–105) of parasites (Anderson et al., Reference Anderson, Lira, Kamhawi, Belkaid, Wynn and Sacks2008). In this study, it is striking that the growth of L. tropica in BALB/c mice appears to be much less vigorous than L. major but both species can benefit substantially from either the vector saliva or PSG. How this general mechanism of parasite survival contributes to L. tropica pathogenesis will require careful further study but is likely to be part of a complex of regulatory mechanisms, which subtly control both the Th1 and Th2 arms of the adaptive immune response.
PSG from natural Leishmania-sand fly combinations significantly contributes to the progression of the infection in skin of the mammalian host. From these findings, we can now add the parasite PSG to the list of vector-derived products that exacerbate L. major infection and significantly improve the currently intractable model of L. tropica infection (Kobets et al., Reference Kobets, Havelková, Grekov, Volkova, Vojtíšková, Slapničková, Kurey, Sohrabi, Svobodová, Demant and Lipoldová2012). Taken collectively, this reinforces the hypothesis that PSG is a common component of sand fly transmission and a virulence factor to Leishmania sand fly vector combinations found in nature. This has implications for interpreting the pathogenesis of Leishmania infection and the choice of an appropriate challenge for anti-leishmanial drug and vaccine screening.
Acknowledgements
We thank Patricia Aiyenuro and Shahida Begum for assistance maintaining the Lu. longipalpis sand fly colony in London and Anna Dostálová and Jitka Myšková for maintaining colonies of P. papatasi, P. duboscqi and P. arabicus sand flies in Prague. These results were presented as part of the 2018 British Society for Parasitology Autumn Symposium on Parasite Glycobiology.
Financial support
This work was supported by the Biotechnology and Biological Sciences Research Council (M.E.R., David Phillips Fellowship, BB/H022406/1); the Wellcome Trust (M.E.R., grant number GR078223MA) and the Czech Ministry of Education (ERA funds (CZ.02.1.01/0.0/0.0/16_019/0000759) to P.V).
Ethical standards
All animal and sand fly infections in the UK were carried out in accordance with the UK Animal Scientific Procedure Act (ASPA) 1986, which transposes European Directive 2010/63/EU into UK national law. Animal studies are approved by the UK home office in granting Project licence 70/8427 under the Animal Scientific Procedure Act and all protocols had undergone appropriate local ethical review procedures by the Animal Welfare and Ethical Review Board (AWERB) of LSHTM.