1. Introduction
1.1. Background
The effect of dietary advanced glycation end products (AGE) and advanced lipoxidation end products (ALE) in health has been a matter of controversy. In 2007, a call for an interdisciplinary debate was made by Professor Henle in the Molecular Nutrition and Food Research Journal to answer the following question: ‘are dietary advanced glycation end products a risk to human health?’ Henle indicated that physiological consequences of Maillard compounds in foods must be carefully discussed(Reference Henle1). Arguments based on papers published in high-impact journals made Šebeková & Somoza(Reference Šebeková and Somoza2) argue for the motion that dietary AGE are a health risk. On the contrary, Ames(Reference Ames3) provided evidence against the motion. No final conclusion was drawn from the debate, which may indicate the need for a more comprehensive examination on this issue. Although it is now widely recognised that dietary AGE are major contributors to the body’s AGE pool, it still remains unclear and controversial whether habitual dietary AGE per se exert negative health effects in humans(Reference Šebeková and Brouder Šebeková4). In the last 20 years, data have been accumulating adding support to the motion that AGE play a significant pathophysiological role, especially in relation to ageing and metabolic disorders(Reference Uribarri, del Castillo and de la Maza5). Since the accumulation of AGE in the human body appears to be progressive throughout life, a preventive action against glycation by dietary adjustments may be warranted(Reference Šebeková and Brouder Šebeková4,Reference Guilbaud, Niquet-Leridon and Boulanger6) . In 2016, Guilbaud et al. (Reference Guilbaud, Niquet-Leridon and Boulanger6) provided dietary strategies to limit the exposure to food AGE. The aim of the present review is to illustrate how the current nutritional recommendations for healthy eating made by the Spanish Society of Community Nutrition (SENC; Sociedad Española de Nutrición Comunitaria) and Harvard University may reduce the body’s AGE/ALE pool.
1.2. What are advanced glycation/lipoxidation end products?
AGE/ALE are formed in thermally processed foods and in the human body. The Maillard reaction and lipid peroxidation are considered the main pathways of AGE/ALE formation (Fig. 1)(Reference Šebeková and Brouder Šebeková4). The Maillard reaction, also called non-enzymic browning or glycation, is a series of non-enzymic reactions that take place during heat processing of foods between the carbonyl group of reducing sugars and the free amino groups of amino acids, peptides or proteins. This reaction is of great importance in food chemistry since it has an effect on colour, texture, aroma and flavour precursors in foods(Reference Silván, van de Lagemaat and Olano7). AGE are also physiologically formed and accumulated in body fluids and tissues of healthy subjects during ageing and they are increased in patients affected by metabolic disorders, particularly in diabetic patients(Reference Šebeková and Brouder Šebeková4).

Fig. 1. Pathways for advanced glycation/lipoxidation end products (AGE/ALE). Structure of the main AGE/ALE reported in foods and in the human body. 3-DG, 3-deoxyglucosulose; CEL, N ϵ-(carboxyethyl) lysine; CHO, carbohydrate; CML, N-carboxymethyl lysine; GO, glyoxal; MG-H1, methylglyoxal-derived hydroimidazolone 1; MGO, methylglyoxal.
The Maillard reaction starts with the condensation of free amino groups of amino acids, peptides or proteins with the carbonyl group of reducing sugars. Lysine and arginine residues of proteins are the most important source of reactive amino groups(Reference Corzo-Martínez, Corzo, Villamiel and Simpson8). When the starting sugar is an aldose (for example, glucose), a relatively stable intermediate called an Amadori product (1-amino-1-deoxi-2-ketose) is formed after a loss of a molecule of water, the formation of a Schiff’s base and Amadori rearrangement. If the starting sugar is a ketose (for example, fructose), an analogous compound called a Heyns compound is formed. Then, the breakdown of Amadori/Heyns compounds and the formation of highly reactive α-dicarbonyl intermediate compounds (for example, glyoxal (GO), methylglyoxal (MGO), diacetyl, 1-deoxyglucosulose or 3-deoxyglucosulose) take place(Reference Vistoli, De Maddis and Cipak9). These α-dicarbonyls form stable peptide-bound amino acid derivates with lysine and arginine side chains of proteins leading to the formation of AGE such as N-carboxymethyl lysine (CML), N ϵ-(carboxyethyl)lysine (CEL), pentosidine, pyrraline and MGO-derived hydroimidazolone 1 (MGH1) (Fig. 1)(Reference Palimeri, Palioura and Diamanti-Kandarakis10,Reference Sadowska-Bartosz and Bartosz11) .
ALE are produced in food and in vivo by carbonyl formed on oxidised lipid and amino compounds(Reference Delgado-Andrade and Fogliano12). The reactive carbonyl species arising from lipid peroxidation are quite heterogeneous and can be divided into three main classes: (1) the α,β-unsaturated aldehydes (4-hydroxy-2-nonenal and 4-hydroxy hexenal and nonenal and acrolein); (2) di-aldehydes (malondialdehyde and GO); and (3) cheto-aldehydes (MGO, 4-oxo-nonenal and the isoketals also called levuglandins). When these compounds are formed from adducts and cross-links generated by the non-enzymic reaction of dicarbonyls produced by lipid peroxidation, the term ALE is used (Fig. 1)(Reference Vistoli, De Maddis and Cipak9). Recently, thirty-five aldehydes and ketones derived from fish oil, the n-3 fatty acid-rich source, by liquid chromatography–tandem MS (LC–MS/MS) have been described. Authors have found different patterns of carbonyl compound generation between n-3 and n-6 fatty acids using a lipidomic approach. They pinpointed that GO resulted from oxidation of oleic acids and EPA, while MGO has a low association with fatty acids(Reference Suh, Niu and Hung13). New dietary ALE (dALE) may be described in the future which may be better chemical indicators of the degree of progress of the reaction during fish processing than those described in Fig. 1 and Table 1.
Table 1. Nutritional composition and advanced glycation end product (AGE) content of foods and beverages present in the Healthy Eating Pyramid and Plate (Fig. 3)*
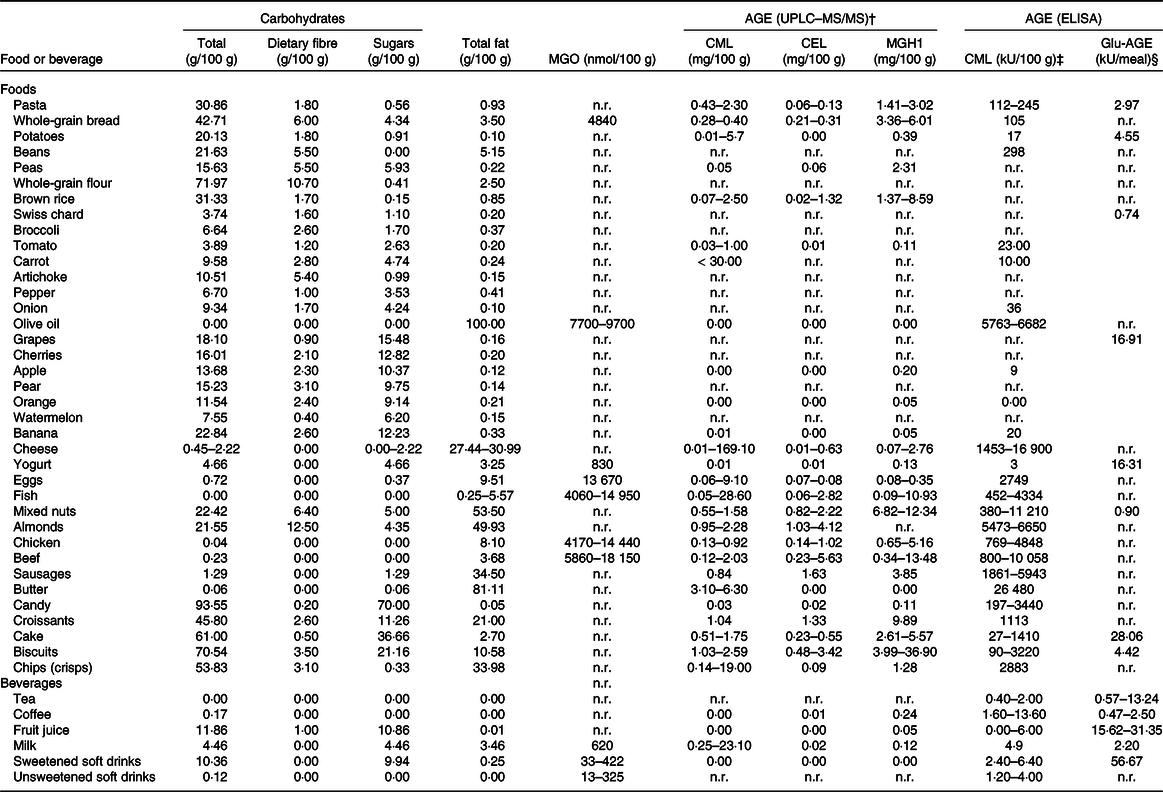
UPLC–MS/MS, ultra-performance liquid chromatography–tandem MS; MGO, methylglyoxal; CML, N-carboxymethyl lysine; CEL, N ϵ -(carboxyethyl)lysine; MGH1, methylglyoxal-derived hydroimidazolone 1; Glu-AGE, glucose-derived AGE (for example, pentosidine); n.r., not reported.
* Data for carbohydrate and fat content were obtained from the National Nutrient Database for Standard Reference Legacy Release of the United States Department of Agriculture (USDA) Agricultural Research Service, while those corresponding to AGE were from † Scheijen et al. (Reference Scheijen, Clevers and Engelen17), ‡ Uribarri et al. (Reference Uribarri, Woodruff and Goodman32) and § Takeuchi et al. (Reference Takeuchi, Takino and Furuno16).
The Maillard reaction and lipid peroxidation are considered the main sources of AGE/ALE, but other mechanisms such as auto-oxidation of monosaccharides, high fructose and ascorbic acid levels, and environmental stress (such as drought or excessive light) also contribute to their formation in plants(Reference Šebeková and Brouder Šebeková4). CML is a commonly used marker compound to evaluate both the progress of the Maillard reaction and lipid peroxidation in foods and a biomarker measured in diabetes and CVD(Reference Tamanna and Mahmood14,Reference Nguyen, van der Fels-Klerx and van Boekel15) . Most of the databases of AGE content in foods include information on CML, which makes the comparison of results published in different studies easier. Moreover, the chronic intake of CML leads to an increase in its content in organs and tissues(Reference Guilbaud, Niquet-Leridon and Boulanger6). However, other AGE besides CML have been proposed as better chemical indicators in foods such as CEL or MGH1 even when it is hard to find information of their content in foods (Fig. 1 and Table 1)(Reference Takeuchi, Takino and Furuno16,Reference Scheijen, Clevers and Engelen17) . The robustness of the methods used for CML analysis is also a matter of controversy. Either liquid chromatography–MS (LC-MS) or ELISA has been widely used to measure this compound. The correlation between values obtained from both methods has been described by different authors and it can be said that, in general, a reasonable relationship between CML determined by ELISA and HPLC electrospray ionisation ion-trap tandem mass spectrometry (HPLC-ESI-ITMS/MS) or ultra-performance liquid chromatography–tandem MS (UPLC–MS/MS) has been established(Reference Srey, Haughey and Connolly18,Reference Gómez-Ojeda, Jaramillo-Ortíz and Wrobel19) .
There are several factors that affect the rate of the Maillard reaction such as pH, temperature, water activity and chemical composition of the food system(Reference Corzo-Martínez, Corzo, Villamiel and Simpson8). The reaction rate generally increases with pH and temperature. Water activity (intermediate moisture = 0·5–0·8) is another important factor influencing the development of the Maillard reaction. The reaction occurs less readily in foods with high water activity due to the dilution of the reactants. However, at low water activity values the mobility of reactants is limited despite their presence at increased concentrations. With regard to the nature of sugars and amino acids, pentoses (for example, ribose) react more readily than hexoses (for example, glucose), which are more reactive than disaccharides (for example, lactose). On the other hand, lysine is the most reactive amino acid(Reference Corzo-Martínez, Corzo, Villamiel and Simpson8).
AGE/ALE (such as CEL and CML) are involved in the pathogenesis of several diseases such as diabetes and its complications, CVD, neurological disorders and normal ageing(Reference Lopez-Moreno, Quintana-Navarro and Camargo20–Reference Münch, Westcott and Menini23). Thus, preventing AGE and ALE formation/accumulation may control significantly the pathogenesis of diabetes complications(Reference Uribarri, del Castillo and de la Maza5). The inhibition of AGE formation might follow several mechanisms involving, for example, aldose reductase, the inhibition of the formation and scavenging of free radicals by different antioxidant mechanisms, reactive dicarbonyl trapping, sugar autoxidation inhibition and amino group binding(Reference Mesías, Navarro and Martínez-Saez24). AGE formation may also be inhibited by modulating the glycaemic response through partially inhibiting digestive enzymes such as α-amylase, β-glucosidase, sucrase, and delay sugar transport across the intestinal barrier(Reference Kerimi, Gauer and Crabbe25,Reference Dueñas Martin, Iriondo-DeHond and del Castillo26) . The search for natural products that can inhibit AGE formation has recently been an objective of worldwide research(Reference Nagai, Shirakawa and Ohno27,Reference Starowicz and Zieliński28) .
Dietary AGE/ALE have been described as a major contributor to the body AGE/ALE pool, which is associated with the development of different pathologies(Reference Clarke, Dordevic and Tan29). Consequently, the aim of the present review is to discuss how a so-called ‘healthy eating behaviour’ may help to reduce the AGE/ALE pool (Fig. 2). In this sense, we propose the Healthy Eating Pyramid of the SENC and the Healthy Eating Plate proposed by Harvard University as recommendations for reducing dietary contribution to the body’s AGE/ALE pool. These strategies involve recommendations for healthy nutrition (see Healthy foods and Healthy beverages sections) and the improvement of food quality (see Food Reformulation and Food processing and culinary practices sections) to reduce the rate of the Maillard reaction. All of them are shown in Fig. 3 and discussed in the following sections.

Fig. 2. Healthy nutrition for reducing the sources of advanced glycation/lipoxidation end product (AGE/ALE) precursors to reduce the risk of pathologies associated with their accumulation.
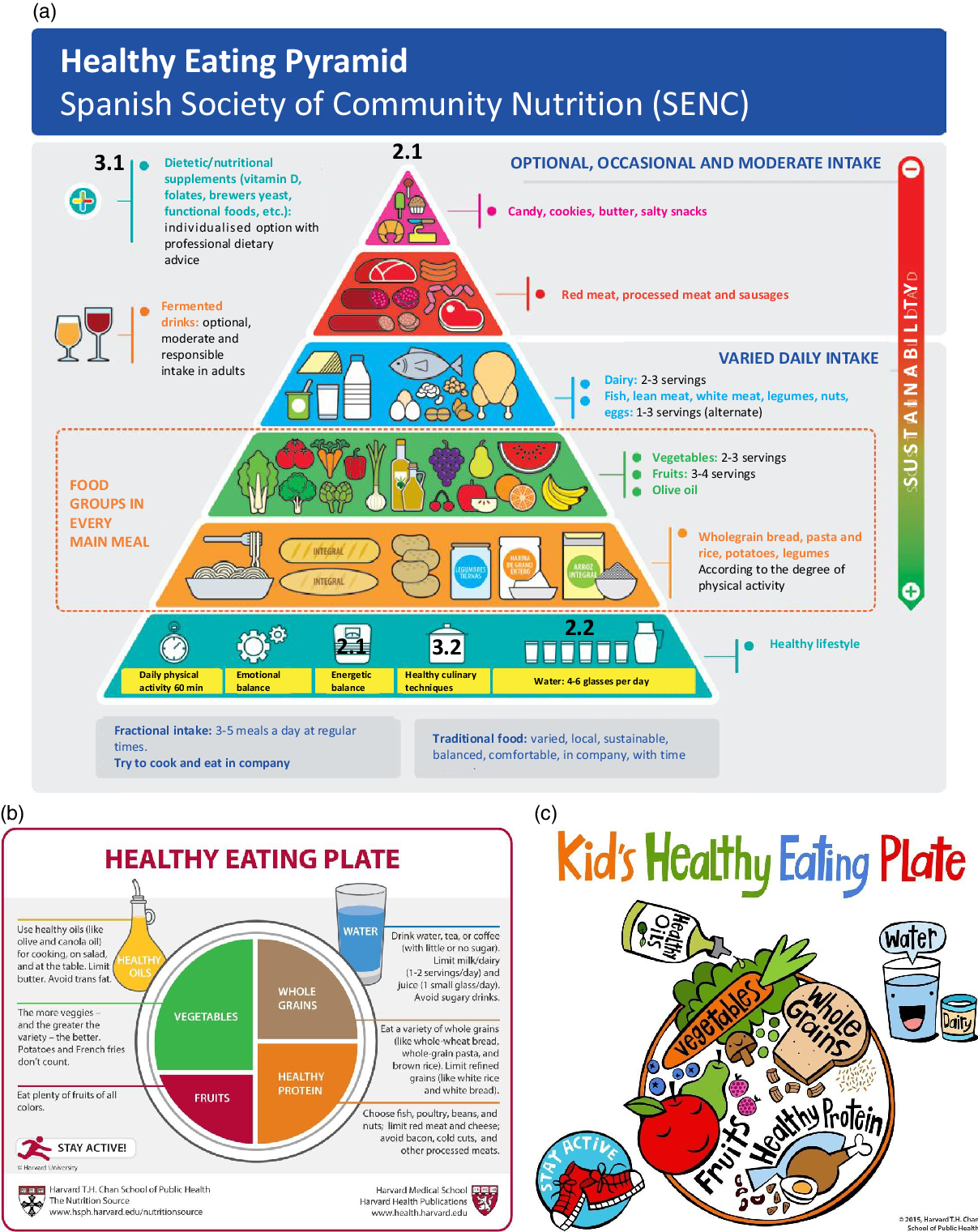
Fig. 3. Healthy eating recommendations: (a) the translated Healthy Eating Pyramid proposed by the Spanish Society of Community Nutrition (SENC, 2018), (b) the Healthy Eating Plate (Copyright© 2011, Harvard University) and (c) The Kid’s Healthy Eating Plate. The Harvard School of Public Health created both Healthy Eating Plates. Copyright © 2015 Harvard T.H. Chan School of Public Health. For more information about The Kid’s Healthy Eating Plate, please see The Nutrition Source, Department of Nutrition, Harvard T.H. Chan School of Public Health (hsph.harvard.edu/nutritionsource/kids-healthy-eating-plate). Numbers in (a) and (b) indicate the different strategies proposed in the present review: 2.1, healthy nutrition; 2.2, healthy hydration; 3.1, food reformulation; and 3.2, culinary practices.
2. Healthy nutrition
2.1. Healthy foods
Dietary intake of foods with a high AGE content seems to predispose to inflammation and deteriorating biological effects, whereas AGE restriction may have an opposite, beneficial impact(Reference Palimeri, Palioura and Diamanti-Kandarakis10). AGE/ALE have been implicated in the pathogenesis of type 2 diabetes and a diet low in these compounds has been shown to improve insulin resistance in individuals with type 2 diabetes(Reference Uribarri, Cai and Ramdas30). To date, there are two databases with the content of CML measured by ELISA in more than 500 food items to know which foods possess high AGE content(Reference Goldberg, Cai and Peppa31,Reference Uribarri, Woodruff and Goodman32) . Takeuchi et al. (Reference Takeuchi, Takino and Furuno16) have assessed by ELISA the concentrations of various AGE in beverages and foods that are commonly consumed in Japan. In addition, there is another more recent database based on data obtained by ultra-performance liquid chromatography–tandem MS(Reference Scheijen, Clevers and Engelen17). Data on content of AGE in foods and beverages, determined using selective analytical methods for individual reaction products by several research groups, are publicly accessible at the web page of the Technical University of Dresden, Germany (https://lemchem.file3.wcms.tu-dresden.de//index.php). Altogether, these databases provide an overview of the presence of AGE/ALE in foods, the consumers’ exposure to these compounds and they can be used as a guide for selecting the strategies for an effective reduction of their dietary intake.
Table 1 shows the content of carbohydrates, fat, MGO and AGE content of foods and beverages present in the Healthy Eating Pyramid and Plate (Fig. 3). Both healthy eating recommendations indicate which foods are healthier and how often they should be consumed. In addition, the pyramid (Fig. 3(a)) considers which culinary practices are more adequate for healthy nutrition. The plate (Fig. 3(b)) recommends drinking water and tea or coffee with no added sugar, and to avoid sugary drinks. These recommendations agree with those proposed by the SENC in their Healthy Hydration Pyramid(Reference Aranceta Bartrina and Arija Val33).
In general, the consumption of low-sugar and low-fat food reduces the availability of substrates of the Maillard reaction, leading to a lower contribution to the body’s AGE/ALE pool. The European Food Safety Authority (EFSA) states that ‘a claim that a food is low in sugars, and any claim likely to have the same meaning for the consumer, may only be made where the product contains no more than 5 g of sugars per 100 g for solids or 2·5 g of sugars per 100 ml for liquids’ and that ‘a claim that a food is low in fat, and any claim likely to have the same meaning for the consumer, may only be made where the product contains no more than 3 g of fat per 100 g for solids or 1·5 g of fat per 100 ml for liquids (1·8 g of fat per 100 ml for semi-skimmed milk)’.
As can be observed in Table 1, foods rich in solid fats, fatty meats, full-fat dairy products and highly processed foods tend to be the richest dietary sources of AGE(Reference Lopez-Moreno, Quintana-Navarro and Camargo20,Reference Monnier34) ; whereas low-fat foods containing complex and simple carbohydrates such as legumes, vegetables, fruits and whole grains and low-fat milk products tend to be relatively low in AGE(Reference Scheijen, Clevers and Engelen17). These carbohydrate-rich foods possess low AGE content since they are composed of complex carbohydrates, mainly dietary fibre, which modulates carbohydrate metabolism, decreasing free sugar bioavailability that contributes to the formation of endogenous AGE. In addition, clinical trials have proved the effect of glycation inhibitor compounds (trans-resveratrol and hesperetin from grapes and citrus) that are present in vegetables and fruits(Reference Xue, Weickert and Qureshi35). Recently, further information on the hesperedin effect on glucose and fructose transport, sucrase activity and glycaemic response to orange juice in a cross-over trial on healthy volunteers has been published(Reference Kerimi, Gauer and Crabbe25). The intake of nutritional supplements or functional foods, which may contain glycation inhibitors and/or modulators of the glucose response, is recommended in the healthy diet proposed by the SENC (Fig. 3)(36).
The bottom of the Heathy Eating Pyramid reported by SENC corresponds to foods recommended on a daily basis such as whole grains, fruits and vegetables and low-fat milk, which are foods with low AGE levels. A certain content of AGE/ALE is present in raw foods of animal and plant origin(Reference Šebeková and Brouder Šebeková4,Reference Takeuchi, Takino and Furuno16,Reference Scheijen, Clevers and Engelen17) . The amount of protein-bound CML or CEL in fruits, vegetables, butter, oils and some beverages is negligible or even under the detection limit of the ultra-performance liquid chromatography–tandem MS (UPLC–MS/MS) method (Table 1)(Reference Scheijen, Clevers and Engelen17). Takeuchi et al. (Reference Takeuchi, Takino and Furuno16) also found very low amounts of AGE in these foods when determined by ELISA. However, in raw meat the amounts of AGE/ALE adducts vary for different animal species, meat cut and meats of different suppliers. For instance, raw pork contains about 50 % less free CML and CEL compared with beef; and in chicken meat, levels of free CML are about the same as in beef, while those of free CEL are on average 11-fold higher(Reference Sun, Tang and Wang37).
On top of the pyramid there are candies, cookies, highly processed foods, such as packaged meats and snack-type foods; and fats, including butter, full-fat cheeses and fried foods, which are nor recommended and the intake of them should be moderate or restricted. Many commercially processed foods such as dry mixes or canned soups, which often contain larger amounts of protein and carbohydrates, undergo Maillard reaction during heat processing and they continue to brown during storage(Reference Uribarri, Woodruff and Goodman32). These guidelines recommended by the SENC are also consistent with recommendations by organisations such as the American Heart Association(Reference Lichtenstein, Appel and Brands38), the American Institute for Cancer Research(39) and the American Diabetes Association(40).
Šebeková & Brouder Šebeková(Reference Šebeková and Brouder Šebeková4) have summarised the information so far published regarding the bioavailability of dietary AGE. Ames(Reference Ames3) reported that up to 80 % of dietary Amadori rearrangement products are not absorbed but are degraded by the gut microflora. However, the majority of the AGE in foods that are absorbed is rapidly excreted by the kidneys. Ames(Reference Ames3) concluded there is no solid evidence obtained using human subjects for a positive relationship between the intake of dietary AGE and the up-regulation of pro-inflammatory markers or changes in the colonic microflora(Reference Ames3). On the other hand, Hellwig et al. (Reference Hellwig, Auerbach and Müller41) have showed for the first time that Escherichia coli strains are able to degrade CML to defined metabolites with the biogenic amine N-carboxymethylcadaverine as the main product. Some clinical studies have demonstrated an effect of probiotics on early glycation since the glycated Hb (HbA1c) content was decreased after a long-term exposure to Lactobacillus strains(Reference Ejtahed, Mohtadi-Nia and Homayouni-Rad42). To the best of our knowledge, a protective effect of probiotics against the accumulation of AGE in the body has not been demonstrated. A pilot study performed by Yacoub et al. (Reference Yacoub, Nugent and Cai43) found that short-term restriction of dietary AGE intake can significantly decrease circulating MGO and CML levels in renal failure patients on maintenance peritoneal dialysis and further suggested that this dietary intervention may have an effect on the gut microbiota. Dietary AGE restriction altered the bacterial gut microbiota with a significant reduction in Prevotella copri and Bifidobacterium animalis relative abundance and increased Alistipes indistinctus, Clostridium citroniae, Clostridium hathewayi, and Ruminococcus gauvreauii relative abundance(Reference Yacoub, Nugent and Cai43). In rats the intake of dietary AGE reduced gut microbiota abundance and diversity, potentially because of their resistance to digestive enzymes, adversely altering gut microbiota composition. Dietary AGE increased protein fermentation and reduced the fermentation of carbohydrates, as evidenced by increased levels of the putrefactive toxic metabolites ammonia and branched-chain fatty acids at the expense of beneficial metabolites like SCFA(Reference Qu, Yuan and Zhao44). There are mixed reports on the effects of high-AGE diets on the production of SCFA(Reference Snelson and Coughlan45). Furthermore, dietary AGE damaged the colonic epithelial barrier, enabling more endotoxins to enter the systemic circulation. These results should be taken into consideration in food processing and nutritional guidelines for modern individuals, especially in a situation in which high-temperature-produced fried and grilled foods are over-consumed. The healthy influences of dietary AGE on gut microbiota are still a matter of debate and larger studies are needed to confirm the effect of dietary AGE on the microbiota.
In summary, these are the main recommendations for a low-AGE/ALE diet:
(1) Be wary of browning.
(2) Eat as much varied natural fresh food as possible.
(3) Reduce the intake of highly processed food.
(4) Eat more foods with low glycaemic index and that are low in fat.
(5) Eat more often at home freshly prepared and minimally processed foods.
All these recommendations agree with those proposed in Fig. 3.
2.1.1. Healthy baby food
The previous recommendations are directed at the adult population. The Kid’s Healthy Eating Plate guidelines adapted from Harvard’s Healthy Eating Plate are shown in Fig. 3(c), which are in line with those proposed for adults(46). However, the body’s AGE/ALE pool is already influenced by the dietary patterns adopted during infancy and may have an impact on the predisposition or susceptibility to particular non-communicable degenerative diseases in adulthood(Reference Šebeková, Simon Klenovics, Brouder Šebeková, Preedy, Watson and Zibadi47). During the first months of life, newborns need a diet of breast milk or infant formula, which is followed by the incorporation of complementary baby foods. Both infant formulas and processed baby foods fall into the category of ‘foods for specific groups’(48).
Infant formulas are cows’ milk-based products, which are adapted to simulate the composition of human milk. Their nutritional value is compromised as some of their main components (the reducing saccharide lactose and whey proteins containing lysine residues) are precursors of Maillard reaction products(Reference Contreras-Calderón, Guerra-Hernández and García-Villanova49,Reference Li, Han and Fu50) . The industrial heat treatments of these foods to ensure microbiological safety and prolonged shelf life result in the formation of substantial amounts of AGE(Reference Birlouez-Aragon, Pischetsrieder and Leclère51). Their content exceeds those present in human breast milk up to 670-fold(Reference Birlouez-Aragon, Pischetsrieder and Leclère51–Reference Pischetsrieder and Henle53) and is dependent on the composition, processing, consistency (liquid, powdered) and type (hydrolysed, non-hydrolysed) of the infant formulas(Reference Šebeková, Simon Klenovics, Brouder Šebeková, Preedy, Watson and Zibadi47). CML is the main Maillard reaction product found in infant formula, but several others have also been identified (CEL, pyrraline, pentosidine, oxalic acid monolysinylamide, MGH1, etc.)(Reference Pischetsrieder and Henle53). Therefore, breast-feeding, either mother’s own milk or banked donor milk, is highly recommended(Reference Arnold54).
Processed baby foods represent a wide range of food products intended to fulfil the particular requirements of infants while they are being weaned(48). These include processed cereal-based foods, which have to be reconstituted with milk or other liquids, as well as puréed foods including a wide range of ingredients. AGE content in these foods largely depends on their composition, which defines the presence of Maillard reaction product precursors, and their industrial processing (thermal treatment and storage time). Bosch et al. (Reference Bosch, Sanz and Montilla55) identified the presence of CML (69–118 mg/100 g protein) in infant foods containing skimmed milk and mixed cereals after 9 months of storage at 37°C. The incorporation of honey increases CML levels compared with only cereal–milk-based infant foods and those with added fruits(Reference Birlouez-Aragon, Pischetsrieder and Leclère51,Reference Bosch, Sanz and Montilla55) . Homogenised jarred foods based on proteins and vegetables are the main sources of the daily intake of the Maillard reaction product 5-hydroxymethylfurfural (HMF) in babies(Reference Calabretti, Calabrese and Campisi56). Consequently, it is recommended to feed babies with homemade foods freshly prepared and appropriately cooked(Reference Calabretti, Calabrese and Campisi56) (with low cooking temperatures, or adding phenolic antioxidants or acids to lower AGE levels).
2.2. Healthy beverages
The School of Public Health of Harvard University and the WHO have indicated that sugar-sweetened beverages are a major contributor to obesity and diabetes development(57,58) . Given the demonstrated adverse effects of such beverages on health and the lack of any health benefits(Reference Curhan and Forman59), there is overwhelming evidence to avoid their consumption for reducing the beverage contribution to the body’s AGE/ALE pool, which is associated with the development of chronic diseases.
Data reported by Aragno & Mastrocola(Reference Aragno and Mastrocola60) suggest that the glycation process following high sugar intake may play a central role in the development of metabolic disturbances(Reference Aragno and Mastrocola60). The most recent reports on the effects of high sugar consumption and diet-derived AGE on human health suggest the need to limit the dietary sources of AGE, including added sugars, to prevent the development of metabolic diseases and related co-morbidities (Table 1)(Reference Aragno and Mastrocola60).
Fructose is widely employed as a sweetener in drinks. Fructose is one of the most rapid and effective glycating agents when compared with other sugars(Reference Aragno and Mastrocola60). Previous studies showed a larger accumulation of CML in plasma, liver, muscle and hippocampus for mice fed a high-fructose diet compared with mice fed a standard diet(Reference Guilbaud, Niquet-Leridon and Boulanger6). Observational research found that the intake of food and beverage sources of high-fructose corn syrup (HFCS) were associated with elevated serum and urinary CML. The authors explained that one possible explanation is that the source of the elevated CML is the intestine, not the food(Reference Gugliucci and Menini61–Reference DeChristopher63). Foods and beverages with high fructose:glucose ratios (HFCS-sweetened foods and beverages, agave syrup, crystalline fructose, apple juice, and apple juice blends) may promote the intestinal in situ formation of readily absorbed, pro-inflammatory extracellular, newly identified, fructose-associated AGE, an overlooked source of immunogenic AGE(Reference DeChristopher63).
Dietary AGE are derived from numerous precursors; they include a broad range of compounds with different structures and molecular weights (Fig. 1)(Reference Takeuchi, Takino and Furuno16). Table 1 provides data on AGE/ALE and their precursors in recommended beverages and in those that should be avoided for healthy nutrition and to decrease their contribution to the body’s AGE/ALE pool. Takeuchi et al. (Reference Takeuchi, Takino and Furuno16) assessed the concentrations of various AGE (glucose-derived AGE (Glu-AGE), fructose-derived AGE (Fru-AGE), CML and glyceraldehyde-derived AGE (Glycer-AGE)) in a total of 1650 beverages and foods that are commonly consumed in Japan. The authors reported that Glu-AGE, Fru-AGE, CML and Glycer-AGE in the examined beverages exhibited concentrations of > 85, 2–12, < 3 % and trace amounts, respectively. The results of their study indicated that some lactic acid bacteria beverages, carbonated drinks, sugar-sweetened fruit drinks, sports drinks, mixed fruit juices, confectionery (snacks), dried fruits, cakes, cereals and prepared foods contain markedly higher Glu-AGE levels than other classes of beverages and foods. The lactic acid bacteria beverages containing large amounts of Glu-AGE were all produced via processes in which HFCS and skimmed milk were mixed and reacted at high temperature. Moreover, beverages that contained artificial sweeteners and carbonated drinks displayed low levels of Glu-AGE(Reference Takeuchi, Takino and Furuno16). Interestingly, tea and coffee did not contain many Glu-AGE or CML. According to the AGE database of the Technical University of Dresden, no CML was reported in brewed coffee while values of 7·8–9·0 mg CML/100 g protein in roasted coffee were found. Scheijen et al. (Reference Scheijen, Clevers and Engelen17) also found nil amounts of CML but 0·01 mg CEL/100 g and 0·24 mg MGH1/100 g in coffee. The daily intake of these beverages is recommended for healthy hydration (Fig. 3) and may contribute to the reduction of endogenous AGE/ALE accumulation because they can modulate the glycaemic response(Reference Kerimi, Gauer and Crabbe25).
In normal rats administered with Glu-AGE-rich beverages an increased hepatic receptor for AGE (RAGE) expression and the enhanced production/accumulation of Glycer-AGE and Glu-AGE were detected. The frequent intake of these beverages and foods may enhance the production/accumulation of Glycer-AGE, leading to Glycer-AGE–RAGE interactions(Reference Sato, Wu and Shimogaito64). In human subjects, it has been demonstrated that roughly 10 % of the AGE in foods and beverages are taken up into the body(Reference Koschinsky, He and Mitsuhashi65). Care should be taken when mixing Glu-AGE-containing products or consuming large amounts of Glu-AGE-containing beverages or foods as this can result in elevated concentrations of Glu-AGE and sugar in the blood and promote the hepatic build-up of Glu-AGE(Reference Takeuchi, Takino and Furuno16).
Despite the French paradox(Reference De Lorgeril, Salen and Paillard66) and the fact that moderate alcohol consumption is recommended within the Mediterranean diet (no more than two drinks per d for men or one drink per d for women)(Reference Aranceta Bartrina and Arija Val33), evidence shows that the ideal situation for health is to not drink at all(67). Alcohol is closely related to about sixty different diagnoses and for almost all there is a close dose–response relationship; so the more you drink, the higher your risk of disease(67).
In addition, AGE and their precursors have also been found in the fermented alcoholic beverages highly consumed in Southern Europe. The main AGE present in beer are pyrraline (0·16–40·00 mg/l) and MG-H1 (0·3–13·7 mg/l)(Reference Hellwig, Witte and Henle68). This beverage also presents AGE precursors such as 3-DG (12·9–136·0 mg/l), among others(Reference Rakete, Klaus and Glomb69). In contrast, red wine presents negligible AGE content(Reference Scheijen, Clevers and Engelen17) but 0·5–1·8 mg/l of MGO(Reference Rodríguez-Cáceres, Palomino-Vasco and Mora-Diez70).
On the other hand, a large number of plants contain bioactive compounds able to inhibit AGE formation in the human body and in processed foods(Reference Bahadoran, Mirmiran and Azizi71). Polyphenols (phenolic acids, flavonoids, stilbenes and lignans) constitute the major group of plant-derived compounds with anti-AGE activity(Reference Peng, Ma and Chen72–Reference Spencer, Abd El Mohsen and Minihane76). Many in vitro experiments demonstrated that polyphenols can inhibit the biosynthesis of AGE through their antioxidant properties, metal-chelating ability, protein interaction, MGO trapping and/or blocking the RAGE(Reference Tan, Wang and Lo77,Reference Chen, Virk and Chen78) .
Tea, Camellia sinensis (Theaceae), the most consumed beverage after water, is an excellent source of flavonoids such as catechins. The most important catechins of green tea are: (–)-epicatechin (EC), (–)epicatechin-3-gallate (ECG), (–)-epigallocatechin (EGC) and (–)epigallocatechin-3-gallate (EGCG)(Reference McKay and Blumberg79). Green tea consumption (drinking) significantly reduces the advanced glycation process, the accumulation of AGE and the cross-linking of tail tendon collagen in diabetes. Catechins have been investigated broadly on their bioactive properties such as anti-inflammation, anticancer and anti-obesity(Reference Babu, Sabitha and Shyamaladevi80). EGCG has demonstrated to be responsible for decreased AGE-stimulated gene expression, the production of TNFα and matrix metalloproteinase-13 (MMP-13) in human chondrocytes(Reference Rasheed, Anbazhagan and Akhtar81). EGCG has also been shown to prevent intracellular AGE formation, trapping reactive dicarbonyl species such as MGO and GO that enhance the production of pro-inflammatory cytokines in monocytes. EGCG was able to attenuate LDL oxidation and glycation under high glucose conditions mimicking diabetes(Reference Peng, Ma and Chao82,Reference Wu, Yeh and Yen83) . Tea also contains quercetin-3-O-rutinoside (rutin), a common dietary flavonoid that is an established antioxidant(Reference Zhang, Zhang and He84). Gut microflora in the large intestine metabolise rutin to a variety of compounds that include quercetin and phenol derivatives with vicinal hydroxyl groups in their structure, such as 3,4-dihydroxyphenylacetic acid (DHPAA) and 3,4-dihydroxytoluene (DHT), which are powerful inhibitors of the formation of CML and fluorescent derivatives (370–440 and 335–385 nm) in histone H1 caused by ADP-ribose, a powerful glycating agent. The plasma concentrations of these rutin metabolites are expected to effectively neutralise the reported plasma concentrations of GO and MGO(Reference Pashikanti, de Alba and Boissonneault85). Rutin was also found to inhibit glucose-induced formation of glycation products in collagen type I in in vitro experiments and to be an effective inhibitor of lipoprotein glycation by increasing the resistance of LDL to high glucose and Cu-mediated oxidation(Reference Cervantes-Laurean, Schramm and Jacobson86,Reference Wu, Lin and Hsieh87) . In addition, as previously mentioned, tea may not contain AGE(Reference Takeuchi, Takino and Furuno16), being a healthy option for its daily intake and for the reduction of the endogenous AGE/ALE pool.
Coffee, Coffea arabiga (Rubiaceae), is also a beverage consumed worldwide. It contains phenolic compounds, mainly caffeoylquinic acids, p-coumaroylquinic, feruoylquinic and dicaffeoylquinic acids, which inhibit protein glycation and dicarbonyl compound formation. Chlorogenic acid (5-caffeoylquinic acid) in addition to other caffeoyl derivative polyphenols contributed to about 70 % of the antioxidant capacity of a coffee extract(Reference Verzelloni, Tagliazucchi and Del Rio88). This compound, also found in the edible parts of some other plants, acts as an anti-AGE agent by metal chelation or by modulation of antioxidant enzyme gene expression(Reference Gugliucci, Bastos and Schulze89). Bioavailability studies carried out in human subjects have indicated that at least six intact major chlorogenic acid compounds are found in human plasma after acute coffee consumption and also that caffeoylquinic acid and dicaffeoylquinic acid isomers are probably differentially absorbed and/or metabolised. In addition, urine does not appear to be a major excretion pathway of intact chlorogenic acid compounds(Reference Monteiro, Farah and Perrone90). Moreover, studies carried out with green coffee samples have shown that the main chlorogenic acid compounds present in the green coffee matrix are also highly bioavailable in human subjects(Reference Farah, Monteiro and Donangelo91). Therefore, chlorogenic acid present in coffee may be able to exert its antiglycative effect in human subjects after absorption. Moreover, Ishizakaa et al. (Reference Ishizakaa, Yamakadoa and Toda92) analysed a large database of individuals who underwent general health screening, and found that coffee intake of three or more cups per d was an independent negative correlate of oxidative stress in men, but not in women(Reference Ishizakaa, Yamakadoa and Toda92). The in vivo antioxidant status associated with coffee consumption and its relation to disease morbidity and mortality need further investigations.
Mate tea, Ilex paraguariensis (Aquifoliaceae), is a South American beverage rich in caffeoyl derivative compounds. A marked inhibition of the formation of AGE and CML was demonstrated in in vitro model systems, which was associated with the presence of caffeic acid and chlorogenic acid(Reference Tsuji-Naito, Saeki and Hamano93). Moreover, chlorogenic acid may interfere with glucose absorption(Reference Ho, Wu and Lin94). It was also shown that extracts of Ilex paraguariensis were able to inhibit AGE-precursor formation in a similar way as synthetic AGE inhibitors, including aminoguanidine or carnosine(Reference Gugliucci and Menini61).
A study in Europe, covering the local plants used in water infusions as aromatic and refreshing hot beverages, showed that the most important plant families used to prepare recreational tea were Asteraceae and Lamiaceae. Tiliaceae had a leading position among the other families, with just a few species represented, showing its importance in European food culture. The majority of the top species are well known for their digestive properties, which is also one of the reasons cited for the selection of plants for teas to accompany meals(Reference Pardo de Santayana, Blanco and Morales95). The most important species according to their use were Thymus serpyllum L. and Melissa officinalis L. (Asteraceae); Achillea millefolium L., Chamaemelum nobile L., Matricaria chamomilla (Lamiaceae) and Tilia cordata (Tiliaceae). In addition, many of the same top species are also cited as having anti-inflammatory properties such as Thymus serpyllum, Achillea millefolium, Chamaemelum nobile and Tilia cordata (Reference Sõukand, Quave and Pieroni96). Different polyphenols (phenolic acids and flavonoids) have been reported in Asteraceae and Tiliaceae. Apigenin glycosides, caffeoylquinic acid and ferulic acid have been reported in A. millefolium, luteolin glycosides and ferulic acid derivatives were reported in A. millefolium and C. nobile and kaempferol glycosides in T. cordata (Reference Wagner and Bladt97,Reference Ali, Gopalakrishnan and Venkatesalu98) . Among the flavonoids mentioned, apigenin and luteolin demonstrated their role as inhibitors of AGE formation in vitro (Reference Peng, Ma and Chen72). Kaempferol is a well-known antioxidant that possesses anti-inflammatory activity. The short-term feeding of aged rats with this compound modulated both AGE accumulation and RAGE expression. Furthermore, kaempferol suppressed age-related NF-κB activation and its pro-inflammatory genes through the suppression of AGE-induced NADPH oxidase activation(Reference Kim, Lee and Kim99). Among phenolic acids, ferulic acid showed antiglycative properties in a bovine serum albumin (BSA) assay(Reference Wu, Yeh and Shih100). This compound could bind to human serum albumin to form complexes, providing unusual protective effects against protein oxidation reducing the formation of CML and fluorescent AGE in vitro (Reference Silván, Assar and Srey101).
The anti-AGE activity of Melissa officinalis extracts has been previously reported. This species contains rosmarinic acid, a dimmer of caffeic acid. The chaperone-like activity of this compound exerted a protective effect against AGE-induced toxicity by suppression of receptor signalling pathways, for example, RAGE antagonists(Reference Miroliaei, Khazaei and Moshkelgosha102).
Topical application of extracts of banabá (Lagerstroemia speciosa), Chinese blackberry (Rubus suavissimus), kuma bamboo grass (Sasa veitchii) and persimmon leaf (Diospyros kaki) for 4 weeks prevented the increase of fluorescent AGE, and increased skin elasticity after 8 weeks(Reference Hori, Yagi and Nomoto103). Further investigation is necessary to determine if oral consumption of herbal teas affects skin. These herbal teas might be used in health foods and cosmetics designed as anti-ageing products for skin and body.
In conclusion, in agreement with the SENC and Harvard University recommendations (Fig. 3), drinking no-added-sugar beverages enriched with inhibitors of the Maillard reaction may be the one of the best nutritional strategies for reducing the formation and accumulation of AGE/ALE in the human body and related diseases.
3. Improvement of food quality
3.1. Food reformulation
A reduced intake of AGE/ALE and the decrease of their formation in the human body is necessary to reduce the risk of non-communicable chronic diseases, which may contribute to sustainable health of the global population(Reference del Castillo, Iriondo-DeHond and Martirosyan104). This term was recently coined by del Castillo et al. (Reference del Castillo, Iriondo-DeHond and Martirosyan104) and it is defined as: ‘a healthy and active ageing avoiding the risk of diseases’. Formulation of dietetic nutritional supplements and novel foods, those called functional foods, is also a strategy for healthy eating, reducing the intake and the formation of AGE in the human body. The recommendations made by the SENC agree with this strategy since nutritional supplements and functional foods are proposed on top of the pyramid under professional advice (Fig. 3).
The EFSA defines food supplements as ‘concentrated sources of nutrients (i.e. mineral and vitamins) or other substances with a nutritional or physiological effect that are marketed in ‘dose’ form (for example, pills, tablets, capsules, liquids in measured doses). A wide range of nutrients and other ingredients might be present in food supplements, including, but not limited to, vitamins, minerals, amino acids, essential fatty acids, fibre and various plants and herbal extracts’. Food supplements are intended to correct nutritional deficiencies, maintain an adequate intake of certain nutrients, or to support specific physiological functions. Guilbaud et al. (Reference Guilbaud, Niquet-Leridon and Boulanger6) propose probiotic supplementation since disruption of the microbiota can lead to physiological disorders and promote the development of some metabolic diseases. However, further studies are needed to study the potential protective effect of probiotics against the accumulation of AGE in the human body (see Healthy foods section).
The EFSA defines functional foods as: ‘A food, which beneficially affects one or more target functions in the body, beyond adequate nutritional effects, in a way that is relevant to either an improved state of health and well-being and/or reduction of risk of disease. A functional food can be a natural food or a food to which a component has been added or removed by technological or biotechnological means, and it must demonstrate their effects in amounts that can normally be expected to be consumed in the diet’(105).
Bioactive compounds derived from vegetables and food waste have been examined for their activities to inhibit AGE formation and damage mediated by AGE. This activity was generally associated with the presence of polyphenols(Reference Lund and Ray106). The potential of red grape skins recovered from winemaking processes as dietary anti-glycation agents preventing the glyco-oxidative stress associated with type 2 diabetes has been proposed. The anti-glycation potential was determined as the ability to inhibit the formation of AGE both in fructose- and in MGO-mediated protein glycation systems. In this study, for the grape skin extracts, AGE-IC50 values, inhibition of protein glycation by 50 %, were found to be between 9·2 and 15·0 µg gallic acid equivalents (GAE)/ml in the BSA/fructose model system and between 19·3 and 26·1 µg GAE/ml in the BSA/MGO model system. All grape skin samples showed similar anti-glycation ability evaluated with reference to GAE. The anti-glycation effectiveness of the studied samples was higher than that of commercial nutraceutical preparations(Reference Sri Harsha, Gardana and Simonetti107). The authors of the investigation concluded that in spite of differences in cultivar, location of the vineyard and winemaking procedures, these by-products could be used as a source of cost-effective anti-glycation agents either as food ingredients or as nutraceutical preparations. The possibility of using red grape skin extract for preventing AGE-mediated diabetic complications has also been reported by Jariyapamornkoon et al. (Reference Jariyapamornkoon, Yibchok-anun and Adisakwattana108). In addition, the protective ability of phenolics from white grape vinification by-products against structural damage of BSA induced by glycation has been reported(Reference Sri Harsha, Lavelli and Scarafoni109). The anti-glycation activity of this by-product was measured by a BSA/fructose model system. Structural modifications of BSA were investigated by two-dimensional isoelectric focusing sodium dodecyl sulfate–PAGE (IEF/SDS–PAGE) and fluorescence measurements. The antiglycation activity ranged between 250 and 711 mmol aminoguanidine Eq/kg. The in vitro MGO-trapping capacity of six red and seven white grape skin extracts obtained from winemaking by-products was also investigated. The trapping ability for red grape skin extracts correlated significantly with total phenolic content and antioxidant capacity. However, no correlations were observed for white grape skin extracts, which suggests that other compounds were involved in the trapping activity(Reference Sri Harsha, Mesias and Lavelli110). The effects of the addition of different ingredients and grape by-products to muffins on CML content have been studied by Mildner-Szkudlarz et al. (Reference Mildner-Szkudlarz, Siger and Szwengiel111). Muffins enriched with grape by-products (20 %) showed lowering of CML level and no significant changes in the sensory profile. Grape by-products added to the model system with protein-rich ingredients resulted in the weakest inhibitory effects, probably due to the polyphenol–protein binding mechanism.
Naringenin, a flavanone that is the aglycone of narirutin present in citrus fruits and tomatoes, has been shown to inhibit glucose transport across the intestine in rats(Reference Kerimi, Gauer and Crabbe25). Teng et al. (Reference Teng, Li and Yu112) have evaluated how naringenin affects AGE formation in bread crust. They found that, with the increase in naringenin content in bread (0·25–1 %, w/w), the formation of CML and total fluorescent AGE was significantly inhibited (9·67–54·27 and 11·79–35·19 %, respectively). Bread with naringenin showed a lower acrylamide content in bread crust, and enhanced antioxidant and antibacterial activities(Reference Teng, Li and Yu112). Similar results were found in bread with incorporation of quercetin (1·2, 2·4 and 3·6 %)(Reference Lin, Gwyneth Tan and Leong113). Therefore, the use of these ingredients should be considered in the development of functional breads.
Olive mill wastewater is a by-product of the olive oil extraction processes with a high content of hydroxytyrosol, hydroxytyrosol derivatives and molecules containing o-dihydroxyl functions such as verbascoside. Studies have revealed that olive mill wastewater phenolic powder (OMW) is able to trap reactive carbonyl species such as hydroxycarbonyls and dicarbonyls; as well as to inhibit the formation of Amadori products and CML. As a consequence, OMW has been proposed as a functional ingredient able to control the Maillard reaction and to improve the nutritional and sensorial attributes of milk(Reference Troise, Fiore and Colantuono114). The antiglycative capacity of olive mill wastewater was evaluated in vitro by BSA-glucose and BSA-MGO assays, formation of Amadori products and direct trapping of reactive dicarbonyls (MGO and GO). Data confirmed that the direct trapping of dicarbonyl compounds is the main route explaining the antiglycative action rather than of the already known antioxidant capacity. Results support further investigations to evaluate the technological feasibility of using olive mill wastewater powders as antiglycative ingredients in foods or in pharmacological preparations in the future(Reference Navarro, Fiore and Fogliano115).
The potential of an aqueous coffee silverskin extract (WO 2013/004873) on the pathogenesis of diabetes has been also investigated(Reference Iriondo-DeHond, Fernandez-Gomez and Martínez-Sáez116). The bioactive compounds present in coffee silverskin extract affect several pathways involved in chronic diseases(Reference Mesías, Navarro and Martínez-Saez24). They are able to inhibit in vitro AGE formation through the interaction of CGA and its derivatives with protein backbone(Reference Fernandez-Gomez, Ullate and Picariello117).
In addition, fermentation by-products as pineapple stem waste obtained during bromelain manufacturing process and buckwheat hull tea have also been described as inhibitors on the formation of AGE(Reference Ye, Ng and Nagai118–Reference Zielinska, Szawara-Nowak and Zielinski120).
The effect of sugarcane molasses extract (well-known source of phenolic compounds) on the formation of CML and CEL has been studied in glucose–lysine model reaction systems. This extract showed significant inhibitory effects. The antiglycative activities of sugarcane molasses extract was associated with its trapping abilities of reactive carbonyl species, such as GO and MGO. The authors emphasised the potential health effects of sugarcane molasses and the possibility of using byproducts as effective ingredients to reduce dietary Maillard reaction end products(Reference Yu, Xu and Yu121).
In vitro and in vivo studies have revealed that loss of antiglycative activity occurs in association with a decrease in phenolic compounds during digestion. Monteiro et al. (Reference Monteiro, Farah and Perrone90) evaluated the drug-kinetic profile in both human plasma and urine and the apparent bioavailability of chlorogenic acid from the ingestion of an extract of green decaffeinated coffee was close to 33 %. These results agree with those obtained in other in vivo studies by Olthof et al. (Reference Olthof, Hollman and Katan122) who found that absorption of chlorogenic acid was 33 (sd 17) % and of caffeic acid 95 (sd 4) %. Preliminary results obtained by Medrano and co-workers (Xavier et al. (Reference Xavier, Miraballes and Pardo123)) showed that the reduction in the phenolic compound content during studies of simulated digestion in extracts of blueberry (Vaccinium corymbosum) and Marcela (Achyrocline satureioides) correlated with antiglycative and antioxidant activities when confronting OH, OOH and ABTS (2,2′-azino-bis(3-ethylbenzothiazoline-6-sulfonic acid)) radicals. Therefore, encapsulation may be a potential strategy to avoid this loss in antiglycative activity after in vivo digestion. Recent research has shown the feasibility of using liposomal encapsulation to protect phenolic compounds like quercetin. Encapsulation prevented quercetin degradation by 80 % during storage(Reference Xavier, Miraballes and Pardo123). Other research studied encapsulated green tea antioxidants in liposomes, which maintained the former’s activity during digestion(Reference Rashidinejad, Birch and Sun-Waterhouse124). Rosmarinic acid and epigallocatechin gallate were found to have significant inhibitory effects when studied at different concentrations as natural inhibitors of Maillard browning in bakery rolls and applesauce(Reference Favreau-Farhadi, Pecukonis and Barrett125). Consequently, the use of encapsulation would be a promising alternative for the incorporation of antiglycative compounds in nutraceuticals or functional foods.
Another strategy that should be considered in the reformulation of novel foods for a lower intake of dietary AGE is the reduction of sugar or fat content. In this sense, the reactants of the Maillard reaction would be less available. In 2004, the WHO challenged the private sector to improve the food supply and reduce levels of energy density, trans-fat, saturated fat, Na and added sugar in their products. A recent study showed that four disciplines are needed for food reformulation: Food Technology, Nutrition & Health, Legislation and Consumer Perspectives(Reference van Gunst, Roodenburg and Steenhuis126). Reformulation by lowering the salt, saturated fat and sugar levels has a proven association with the reduction of the risk of chronic diseases(127).
In summary, reformulation may be another strategy for the reduction of dietary AGE intake. The reduction of sugar and fat, and the addition of inhibitors from food by-products might be a possible strategy to achieve sustainable health(Reference del Castillo, Iriondo-DeHond and Martirosyan104).
3.2. Food processing and culinary practices
The rate of formation and the diversity of generated AGE during food cooking also depend on food processing and culinary practices. CML levels in foods can be reduced by modifying the food processing parameters affecting the degree of progress of the Maillard reaction such as heating time, temperature and the type of cooking procedure, or using inhibitors during the culinary preparation(Reference Sharma, Kaur and Thind128). Methods of water-based cooking processes, such as boiling and steaming, as well as shorter cooking times and lower cooking temperatures are recommended to achieve the goal.
Thermal processing is an important part of modern food preparation that can increase palatability, digestibility, reduces microbial content and prolongs shelf life. Heat, however, accelerates significantly the reaction between carbonyl groups and amino acids leading to the formation of numerous Maillard reaction product intermediates with different molecular sizes and composition(Reference Poulsen, Hedegaard and Andersen129). As a rule, the rate of the Maillard reaction at least doubles when the temperature is increased by 10°C(Reference Nursten130). Therefore, many actions can be easily taken to lower the dietary AGE consumption, particularly during cooking. In this sense, we should avoid preparing food with high heating temperature and long-time cooking by cooking methods such as grilling, frying, deep fat frying and roasting that are known to produce higher levels of AGE in food(Reference Uribarri, Woodruff and Goodman32). In contrast, methods of preparation that are water-based, such as boiling, and steaming, as well as shorter cooking times and lower cooking temperatures will tend to generate lower amounts of AGE(Reference Uribarri, Woodruff and Goodman32). The SENC also considers these culinary practices (Fig. 3) for healthy eating.
Scheijen et al. (Reference Scheijen, Clevers and Engelen17) have found high AGE levels in food items which had been prepared by exposure to heat, industrial conserving or products containing chocolate, nuts or grains, whereas food products with short or no heat processing, low protein and/or carbohydrate content, or high water content had low or negligible AGE content. In their study, groundnuts, groundnut butter, rusk, biscuits, cereals, toast and high-heat-processed meats, such as red cooked beef, canned beef steak and black pudding, had the highest levels of CML and CEL, while coffee, fruits, vegetables, butter, olive oil and red wine had negligible AGE content(Reference Scheijen, Clevers and Engelen17). Nuts, and particularly almonds, are sometimes consumed after roasting (which is typically between 140 and 180°C). Roasting may cause changes to nut lipids and phytochemicals and generate AGE through the Maillard reaction(Reference Zhang, Huang and Xiao131).
The presence of reactive dicarbonyls in food ingredients and heat-treated foods has been ascribed as an important contributor to AGE formation(Reference Degen, Hellwig and Henle132). A body of evidence demonstrated that culinary processing promotes a higher level of dicarbonyls in processed foods when compared with that reported in raw materials(Reference Lingnert, Grivas and Jägerstad133). Processing at high temperatures triggers more dicarbonyl production, which in turn, also affects CML generation(Reference Nguyen, van der Fels-Klerx and van Boekel134).
Another factor that affects the rate of the Maillard reaction, as previously mentioned in the introduction, is water activity. Dehydration increases the contact and chemical reaction between food ingredients. Therefore, dry heat cooking has been found to promote formation of dietary AGE and the levels can be increased up to 100-fold above that found in uncooked food for most food categories(Reference Uribarri, Woodruff and Goodman32). Similarly, oxidation is also an important factor in increasing the CML production during food processing, being even more important than the change in the moisture content, as recently reported(Reference Xu, Yue and Kang135).
Another important factor affecting the formation of AGE is the initial pH of the reactants and the buffering capacity of the system, which influence both the rate and the direction of the pathway of the Maillard reaction. The rate of the Maillard reaction is considered to be low at acidic pH and being maximal when pH 10 is reached(Reference Sharma, Kaur and Thind128). Therefore, AGE formation seems to be reduced by the use of acidic marinades such as lemon juice or vinegar(Reference Uribarri, Woodruff and Goodman32).
The cooking process can also influence other factors, which can in turn indirectly modulate AGE formation by modifying the concentrations of phytochemicals such as polyphenols (phenolic acids, flavonoids, stilbenes and lignans). They constitute the major group of plant-derived compounds with anti-AGE activity. For instance, culinary preparation plays a significant role in the final polyphenol content in processed foods, which can act as Maillard reaction inhibitors. The quercetin content of tomatoes and onions can be reduced by up to 80 % from boiling, 65 % from microwaving, and 30 % from frying(Reference Pandey and Rizvi136). Furthermore, many components commonly found in foods such as guanidines, flavonoids and phenolic acids can serve as scavengers of the 1-2-dicarbonyl compounds such as MGO and GO, leading to a decrease in the amount of AGE(Reference Thornalley137–Reference Peng, Cheng and Ma140). In a sponge cake model, the authors tried to determine the effects of the addition of AGE/ALE inhibitors and different types of sugar and cooking oil on CML and CEL formation. Cakes baked using glucose produced the highest level of CML (2·07 (sd 0·24) mmol/mol lysine); cakes containing oil generated greater concentrations of CML than sucrose; whereas the cake baked using fructose produced the highest concentration of CEL (25·1 (sd 0·15) mmol/mol lysine). The addition of ferulic acid and thiamin in cakes reduced CML and CEL formation(Reference Srey, Hull and Connolly141).
The presence of transition metals during cooking is also an important factor that contributes to AGE formation(Reference Corrales Escobosa, Wrobel and Yanez Barrientos142). Although there is scarce or almost no information about the possible enhancer effect of metal cooking utensils in AGE formation, their generation might be facilitated when Cu utensils or those made with other metals are employed at high temperatures for a long period of time. Further research should be conducted.
In summary, the recommendations of culinary practices for low AGE formation, in foods for the adult population and for children, are as follows:
(1) Use of lower cooking temperatures rather than high cooking temperatures.
(2) Steaming, stewing and poaching are better cooking methods than frying, grilling and roasting.
(3) Phenolic antioxidants can inhibit the formation of AGE.
(4) Addition of acids (for example, vinegar, lemon juice) to food lowers AGE levels.
4. Conclusions
There is evidence supporting an important contribution of diet to the endogenous pool of AGE/ALE, which increases oxidative stress and inflammation and leads to the occurrence of chronic diseases such as diabetes and obesity. Therefore, we propose the Healthy Eating Pyramid and Plate as recommendations for the reduction of AGE/ALE in foods and their formation in the human body, which are as follows:
(1) Healthy nutrition
(a) Reduce the intake of highly processed food and eat more foods with low glycaemic index and low fat.
(b) Drink no-added-sugar beverages enriched with inhibitors of the Maillard reaction.
(2) Improvement of food quality
(a) Food reformulation: Reduction of sugar and fat, and the addition of inhibitors of the Maillard reaction and/or modulators of the glycaemic response such as phenols.
(b) Culinary practices: Steaming, stewing and poaching are recommended. Phenolic antioxidants and acids may be added to inhibit the formation of AGE/ALE during food preparation.
Recommendations should be considered in order to reduce the body’s pool of AGE/ALE and the subsequent risk of non-communicable chronic diseases.
Acknowledgements
This review was written on behalf of the Iberoamerican AGE Group: Rosana Filip (Argentina), Marisa Passarelli (Brazil), Sergio Salazar Villanea (Costa Rica), Armando Rojas Rubio, Ileana Gonzalez and Maria Pia de la Maza (Chile), Ma. Eugenia Garay-Sevilla, Armando Gomez Ojeda, Claudia Luevano Contreras, Maciste H. Macias Cervantes, Katarzyna Wrobel and Kazimierz Wrobel (Mexico), Manuel Portero-Otin and Ma. Dolores del Castillo (Spain), Alejandra Medrano (Uruguay) and Jaime Uribarri (USA). The authors thank the University of Guanajuato for holding the 3rd Iberoamerican Symposium of AGE, ‘AGEs in nutrition and metabolic diseases and functional foods as anti-glycation agents’ that took place from 24 to 26 October 2018 (http://agesenlasalud.com/).
The SUSCOFFEE (AGL 2014-57239-R) and ALIBIRD-CM (S2013/ABI-2728) projects funded the present review. The project ‘Nuevos conocimientos para la sostenibilidad del sector cafetero’ funded by CSIC (201970E117) also funded the present review.
There are no conflicts of interest.