Introduction: early data and similarities
Equids (horses, donkeys, mules and hinnies) originated about 55 million years ago in North America from a browsing species named Hyracotherium (Librado and Orlando, Reference Librado and Orlando2021), which had the size of a large dog. All living species of equids belong to the genus Equus, composed of 2 lineages, caballine and non-caballine animals, that are split into 3 phylogenetic clades. The domestic horse (Equus ferus caballus) and the Przewalski's horse (Equus ferus przewalskii) belong to the first clade (caballine horses), while zebras and wild asses belong to the other 2 clades (non-caballine horses) (Cucchi et al., Reference Cucchi, Mohaseb, Peigné, Debue, Orlando and Mashkour2017). The modern horse has been intensely raised and selected (kinship) for their athletic potential focusing on morphological parameters of body weight (BW), withers height and also sports performance (Brown-Douglas et al., Reference Brown-Douglas, Pagan, Stromberg and Pagan2009; Schrurs et al., Reference Schrurs, Blott, Dubois, van Erck-Westergren and Gardner2022; Dall'Anese et al., Reference Dall'Anese, Silva Junior, Abrahao, Dias de Castro, Brandão, Yoshitani, Knopp and Molento2023).
It is commonly believed that donkeys are short horses with long ears; however, these 2 animal species are different not only in their physical features and behaviour but also in their genetic and physiological characteristics (Lizzaraga et al., Reference Lizzaraga, Sumano and Brumbaugh2004). The chromosome number of horses and donkeys is 64 and 62, respectively, making their hybrids (mules and hinnies/asses) infertile animals. There are approximately 175 different breeds of donkeys worldwide (DAD-IS, 2017; https://www.fao.org/dad-is), characterized by their ability to survive in mountainous and semi-arid environments with scarce water availability (Burden and Thiemann, Reference Burden and Thiemann2015). Donkeys can survive even having a water loss of 20–30% of their weight (Matthews and van Loon, Reference Matthews and van Loon2019), whereas horses are less tolerant of water deprivation (Matthews et al., Reference Matthews, Taylor and Hartsfield1997). Furthermore, even with the availability of water, the urine output of donkeys is lower than horses (Grosenbaugh et al., Reference Grosenbaugh, Reinemeyer and Figueiredo2011). Contrary to what happens in horses, donkeys have a greater ability to digest fibres of low-nutritional value. These animals are characterized by their lower energy requirement (about 50–75%) than that needed by horses of the same size (Smith and Burden, Reference Smith, Burden, Geor, Coenen and Harris2013).
Different responses to pain and fear by donkeys, compared to horses, led to the belief that donkeys could have superior tolerance to distress. For this reason, they are considered stoic animals that do not need regular veterinary care, vaccinations or anthelmintic treatments (Molento and Vilela, Reference Molento and Vilela2021). However, donkeys may respond to discomfort and pain more subtly than horses. As an example, sick donkeys (i.e. parasites and bacterial infections) often show dullness and depression but the clinical diagnosis of the disease is performed only at an advanced stage (Burden and Thiemann, Reference Burden and Thiemann2015).
Parasitic infections are the most important limiting factors for equids’ health and performance, as all common parasites of horses infect donkeys (Matthews and Burden, Reference Matthews and Burden2013). In comparison, scientific publications on parasites in horses are 20 times higher than those in donkeys (Molento and Vilela, Reference Molento and Vilela2021). Figure 1 shows a map of the main parasite studies only in donkeys with their geographical location. In this review, the differences between the main helminthic diseases affecting donkeys and horses, and the different treatment and management strategies will be examined. Some recommendations will be also highlighted.

Figure 1. Choropleth map of the main epidemiological studies on major helminth infection in donkeys. Numbers in square brackets [*] represent the references reported in Table 1. Africa: Egypt [1–2], Ethiopia [3–38], Kenya [39–40], Morocco [41], Nigeria [42–43], Sudan [44–45], South Africa [46–47], Uganda [48–49]; America: Mexico [50–52]; Asia: India [53–57], Iran [58–62], Iraq [63], Mongolia [64]; Europe: Denmark [65], Germany [66–68], Italy [69–73], Macedonia and Thessalia-Greece [74], Portugal [75–76], Serbia [77], Turkey [78–82], Ukraine [83–84].
Table 1. Epidemiological studies of major helminth infections in donkeys

+, positive animals; [*], see Fig. 1.
Helminth infections in horses and donkeys: head to head!
Horses and donkeys not only share similar parasites but also hold high helminth biodiversity (Gianfaldoni et al., Reference Gianfaldoni, Barlozzari, Mancini, Di Domenico, Maestrini and Perrucci2020; Sousa et al., Reference Sousa, Anastácio, Nóvoa, Paz-Silva and Madeira de Carvalho2021). Both species can act as reservoirs for each other; however, in donkeys parasitized with a high helminth burden, clinical signs such as diarrhoea and poor body condition are less common than in horses (Matthews and Burden, Reference Matthews and Burden2013). Therefore, donkeys appear to be healthy even in the presence of a high-parasitic load (Maestrini et al., Reference Maestrini, Molento, Mancini, Martini, Angeletti and Perrucci2020). The prevalence of major helminth infections in donkeys is reported in Table 1.
Intestinal strongyles: epidemiology, diagnostic and innovative research
As in horses and also in donkeys, the most common nematode parasites are intestinal strongyles (large and small strongyles) (Buono et al., Reference Buono, Veronesi, Pacifico, Roncoroni, Napoli, Zanzani, Mariani, Neola, Sgroi, Piantedosi, Nielsen and Veneziano2021; Dias de Castro et al., Reference Dias de Castro, Oliveira Júnior, Costa Perez, Carvalho, De Souza Ramos, Ferraz and Molento2022). In both species, parasitic infections are caused mainly (>90%) by small strongyles (Buono et al., Reference Buono, Veronesi, Pacifico, Roncoroni, Napoli, Zanzani, Mariani, Neola, Sgroi, Piantedosi, Nielsen and Veneziano2021; Jota Baptista et al., Reference Jota Baptista, Sós, Szabados, Kerekes and Madeira de Carvalho2021; Nielsen et al., Reference Nielsen, Gee, Hansen, Waghorn, Bell and Leathwick2021). A recent meta-analysis reported that Cylicocyclus nassatus, Cylicostephanus longibursatus and Cyathostomum catinatum represent about 55% of the specimens in horses (Bellaw and Nielsen, Reference Bellaw and Nielsen2020). The most common genera for donkeys were Cylicostephanus spp. and Cylicocyclus spp. (Maestrini et al., Reference Maestrini, Molento, Mancini, Martini, Angeletti and Perrucci2020; Perrucci et al., Reference Perrucci, Salari, Maestrini, Altomonte, Guardone, Nardoni, Molento and Martini2021).
In horses (Nielsen et al., Reference Nielsen, Gee, Hansen, Waghorn, Bell and Leathwick2021) and donkeys (Perrucci et al., Reference Perrucci, Salari, Maestrini, Altomonte, Guardone, Nardoni, Molento and Martini2021), small strongyle infections (cyathostominosis) are often asymptomatic. However, cyathostomins can cause colic, reduced nutrient absorption, diarrhoea and weight loss, and in some cases intestinal infarction and death in horses (Molento, Reference Molento2005; Steuer et al., Reference Steuer, Loynachan and Nielsen2018; Walshe et al., Reference Walshe, Mulcahy, Crispie, Cabrera-Rubio, Cotter, Jahns and Duggan2021). In donkeys, clinical signs due to parasitic infections are somewhat rare (Getachew, Reference Getachew2006; Burden et al., Reference Burden, Du Toit, Hernandez-Gil, Prado-Ortiz and Trawford2010). It is known that a high-parasitic fecal egg count (FEC) (>1000 eggs per gram – EPG) may cause quantitative and qualitative milk reduction in asymptomatic lactating jennies (Perrucci et al., Reference Perrucci, Salari, Maestrini, Altomonte, Guardone, Nardoni, Molento and Martini2021). Small strongyles can be responsible for a clinical manifestation known as larval cyathostominosis in horses. This demonstration is caused by the synchronous emergence of the encysted larvae from the mucosa of the colon and caecum causing severe typhlocolitis. Although it is quite uncommon in both animal species, in the horse, the fatality rate of this syndrome can reach 50% (Love et al., Reference Love, Murphy and Mellor1999). Even though larval cyathostominosis has been reported in working donkeys (Oryan et al., Reference Oryan, Kish and Rajabloo2015), the fatality rate of this syndrome is not known for the species. Matthews and Burden (Reference Matthews and Burden2013) consider that the fatality would be lower than that for horses. Although horse foals (<1 year), yearlings (1–2 years) and young horses (3–5 years) are responsible for the majority of intestinal strongyle egg excretion (Lester et al., Reference Lester, Morgan, Hodgkinson and Matthews2018; Scala et al., Reference Scala, Tamponi, Sanna, Predieri, Dessì, Sedda, Buono, Cappai, Veneziano and Varcasia2020), in donkeys this phenomenon still needs to be determined. Until recently, no differences in parasite FEC have been observed among age categories (da Costa et al., Reference da Costa, Vileila and Feitosa2018; Maestrini et al., Reference Maestrini, Molento, Mancini, Martini, Angeletti and Perrucci2020; Buono et al., Reference Buono, Veronesi, Pacifico, Roncoroni, Napoli, Zanzani, Mariani, Neola, Sgroi, Piantedosi, Nielsen and Veneziano2021). Moreover, it has been reported that intestinal strongyle FEC is higher in donkeys than in horses (Mezgebu et al., Reference Mezgebu, Tafess and Tamiru2013). This trend is not quite implicit but perhaps it could be due to different reasons, such as the lower frequency of anthelmintic treatment in donkeys than in horses (Buono et al., Reference Buono, Veronesi, Pacifico, Roncoroni, Napoli, Zanzani, Mariani, Neola, Sgroi, Piantedosi, Nielsen and Veneziano2021). Another interesting observation is the smaller amount of feces produced by donkeys when compared to an average-sized horse. Assuming a similar infection density, horses that produce a higher amount of feces (15–20 kg day−1) than donkeys (6–10 kg day−1) would have a dilution of eggs on EPG, showing a lower FEC than donkeys (M. Molento, personal observation). More data need to be gathered (i.e. performance, EPG, necropsy) to demonstrate this biological condition.
Large strongyles encompass Strongylus spp., Triodontophorus spp., Craterostomum acuticaudatum, Oesophagodontus robustus and Bidentostomum ivaschkini (Lichtenfels et al., Reference Lichtenfels, Kharcenko and Dvojnos2008). Of these, the most pathogenic are those belonging to genus Strongylus (Cav et al., Reference Cav, Vidyashankar and Nielsen2013). Three species of Strongylus spp. infect horses and donkeys (Strongylus vulgaris, Strongylus equinus and Strongylus edentatus). In donkeys, Strongylus asini has also been reported, whereas horses are less susceptible to this nematode (Malan et al., Reference Malan, de Vos, Reineke and Pletcher1982). Strongylus asini is morphologically more similar to S. vulgaris than S. equinus and S. edentatus; however, the internal transcribed spacer-2 sequence of S. asini proved to be more similar to S. equinus and S. edentatus (Hung et al., Reference Hung, Jacobs, Krecek, Gasser and Chilton1996). In donkeys and zebras, S. asini develops in the lumen of the portal vein, having a comparable life cycle to S. vulgaris (Malan et al., Reference Malan, de Vos, Reineke and Pletcher1982). Generally, large strongyles are less abundant than small strongyles in horses and donkeys and these parasites are less common in farms that make constant use of macrocyclic lactones (MLs) [i.e. ivermectin (IVM), abamectin, moxidectin (MOX)]. It has been reported that the prevalence of S. vulgaris may increase if selective therapy is adopted in horses (Tydén et al., Reference Tydén, Enemark, Franko, Höglund and Osterman-Lind2019) and in donkeys (Sousa et al., Reference Sousa, Anastácio, Nóvoa, Paz-Silva and Madeira de Carvalho2021).
In horses, the overwhelming majority of intestinal strongyle egg excretion is concentrated in certain animals. In general, 15–30% of adult horses shed approximately 80% of eggs (80:20 distribution rule) (Tzelos and Matthews, Reference Tzelos and Matthews2016; Lester et al., Reference Lester, Morgan, Hodgkinson and Matthews2018), and this pattern is known as overdispersion. In donkeys, this behaviour has been less investigated and the levels of egg excretion are quite different, as about 40% of adult donkeys can shed approximately 80% of eggs, suggesting an 80:40 distribution rule (Buono et al., Reference Buono, Veronesi, Pacifico, Roncoroni, Napoli, Zanzani, Mariani, Neola, Sgroi, Piantedosi, Nielsen and Veneziano2021). These differences in the overdispersion of intestinal strongyle eggs between horses and donkeys have important practical implications. In horses, the anthelmintic treatment of 20% of the animals is adequate to limit pasture contamination, whereas in donkeys the number of treated animals to obtain the same result would be considerably higher (Buono et al., Reference Buono, Veronesi, Pacifico, Roncoroni, Napoli, Zanzani, Mariani, Neola, Sgroi, Piantedosi, Nielsen and Veneziano2021).
As selective therapy and other on-farm management (i.e. reproduction) focuses on individual animal performance, it has been shown that invasion of the intestinal mucosa by parasites activates a defensive mechanism involving the solute-like carrier family 11a1 (SLC11a1) gene, also known as NRAMP1 (Pires et al., Reference Pires, de O. Ganzella, Minozzo, Dias de Castro, Moncada, Klassen, Ramos and Molento2021). The authors were looking to determine the DNA methylation profile of this gene in cyathostomin-infected horses correlating with FEC. First, they have shown that in the core of this gene, there were 2 cytosines adjacent to guanine (CpG) islands. The data presented a positive epigenetic correlation between the hypermethylation of island 2 of CpG of the gene and FEC. This information can help explain the differences in FEC detected among animals raised under similar conditions. Further research is being undertaken, looking to elucidate the host-specific aspects and the involvement of certain genes across different horse categories (foals, yearlings and adult horses) that may be related to host resilience to parasitic infections. In the future, epigenetic processes shall be used as biomarkers to identify and target animals for anthelmintic treatments, as well as other breeding purposes (Pires et al., Reference Pires, de O. Ganzella, Minozzo, Dias de Castro, Moncada, Klassen, Ramos and Molento2021). Epigenetic studies are still rare for horses and no such data have been produced for donkeys. As seen above, cyathostomin infections may be measured by individual FEC to be used in selective anthelmintic therapy. The difference in FEC is a phenotypic condition that might be explained by the genetic effects of horses. A new research approach was used by Dias de Castro et al. (Reference Dias de Castro, Oliveira Júnior, Costa Perez, Carvalho, De Souza Ramos, Ferraz and Molento2022) that focused on genome-wide association studies (GWASs) using the Illumina Equine 70K BeadChip to correlate genomic variants and FEC of 90 thoroughbred horses. The GWAS used a panel containing 65 157 single-nucleotide polymorphism (SNP) markers. The analysis revealed 33, 21, 30, 21 and 19 genes related to FEC, packed cell volume, eosinophils, neutrophils and lymphocyte count, respectively. The data demonstrate a good correlation between important phenotypic health traits and the potential-specific SNP markers.
Molento and Vilela (Reference Molento and Vilela2021) have proposed a susceptible–infected–recovered (SIR) predicted model for cyathostomins under limited (low host infection rate), moderate and severe (high host infection rate) infectivity scenarios for donkeys. The process data confirmed that once the infection level is low, disease recovery would be much faster than at the high-risk level. The larval numbers on pasture, parasite challenge and development in the host, parasite lifespan and the return of host susceptibility shall be considered when interpreting the complexity of the host–parasite model. The SIR model simulations should be taken into consideration when proposing herd management and health control programmes for donkeys and horses in distinct climatic zones (Molento and Vilela, Reference Molento and Vilela2021).
Considering the widespread anthelmintic resistance (AR), the use of selective therapy has been advocated to control parasitic infections, avoiding parasite selection. In horses, a cut-off of 200–500 EPG has been adopted (Nielsen et al., Reference Nielsen, Pfister and von Samson-Himmelstjerna2014), whereas in donkeys there are no specific indications, and a value of 300 EPG has been suggested (Matthews and Burden, Reference Matthews and Burden2013). However, considering that donkeys with >1000 EPG are often asymptomatic this safe FEC limit could be increased. As drug treatment is a human-made perturbation, the problem is that the lack of efficacy maintains the parasitic infection, increasing the risk of heavy parasitic infections of equids.
The diagnosis of intestinal strongyles is routinely based on copromicroscopic examination (Dias de Castro et al., Reference Dias de Castro, Abrahão, Buzatti, Molento, Bastianetto, Rodrigues, Lopes, Silva, Green de Freitas, Conde and de Almeida Borges2017) and fecal culture for larval identification (Bevilaqua et al., Reference Bevilaqua, Rodrigues and Concordet1993; Lichtenfels et al., Reference Lichtenfels, Kharcenko and Dvojnos2008; Madeira de Carvalho et al., Reference Madeira de Carvalho, Fazendeiro and Afonso-Roque2008; Santos et al., Reference Santos, Dias de Castro, Giese and Molento2016, Reference Santos, Madeira de Carvalho and Molento2018) to differentiate between large and small strongyles. However, larval cultures can result in false negative for S. vulgaris but real-time polymerase chain reaction (PCR) (Nielsen et al., Reference Nielsen, Peterson, Monrad, Thamsborg, Olsen and Kaplan2008), conventional PCR and other PCR-based methods (Gasser et al., Reference Gasser, Stevenson, Chilton, Nansen, Bucknell and Beveridge1996; Hung et al., Reference Hung, Gasser, Beveridge and Chilton1999) are available for S. vulgaris diagnosis in horses and donkeys (AbouLaila et al., Reference AbouLaila, Allam, Roshdey and Elkhatam2020).
Parascaris spp.
Generally, equine ascarids refer to only 1 species: Parascaris equorum. However, in the literature other 2 species have been described: Parascaris univalens and Parascaris trivalens. These parasite species differ in having 1 (P. univalens), 2 (Parascaris bivalens also named P. equorum) (Goday and Pimpinelli, Reference Goday and Pimpinelli1986) or 3 (P. trivalens) pairs of chromosomes (Li, Reference Li1937). Hybrids between P. univalens and P. equorum have been described; however, they are sterile (Goday and Pimpinelli, Reference Goday and Pimpinelli1986). Parascaris univalens and P. equorum are quite similar morphologically and they differ only in their spicula, which appear distally truncated in P. univalens and rounded in P. equorum respectively (Biocca et al., Reference Biocca, Nascetti, Iori, Constantini and Bullini1978). Different ascarid populations were karyotyped and identified as P. univalens suggesting that this ascarid species and not P. equorum is the predominant one in horses (Martin et al., Reference Martin, Hoglund, Bergstrom, Lindsjo and Tydén2018, Reference Martin, Svansson, Eydal, Oddsdóttir, Ernback, Persson and Tydén2021a). There are few phylogenetic analyses for donkeys; however, a recent study on mitochondrial genes COX1 and NADH1 showed that Parascaris spp. isolated from donkeys formed a distinct clade compared to those collected from mountain zebras and domestic horses (Peng et al., Reference Peng, Shen, Zhang, Li, Wang, Zhai, Hou and Li2019). Furthermore, a recent whole-genome analysis performed in horses, donkeys and zebras showed that P. univalens found in horses belong to distinct clades than those reported in donkeys and zebras (Han et al., Reference Han, Lan, Lu, Zhou, Li, Lu, Wang, Li, Du, Guan, Zhang, Sahu, Qian, Zhang, Zhou, Guo, Chai, Wang, Liu, Liu and Hou2022).
Parascaris spp. in horses represents the most important parasite infecting young animals, causing coughing, nasal discharge, lethargy, poor appetite, diarrhoea and colic (Clayton and Duncan, Reference Clayton and Duncan1978). In working equids, poor body condition has been associated with ascarid infection (Getachew et al., Reference Getachew, Trawford, Feseha and Reid2010; Seyoum et al., Reference Seyoum, Tesfaye and Derso2015). However, horse foals and donkeys bred under optimal farm management programmes may not show this pattern (Bellaw et al., Reference Bellaw, Pagan, Cadell, Phethean, Donecker and Nielsen2016; Buono et al., Reference Buono, Veronesi, Pacifico, Roncoroni, Napoli, Zanzani, Mariani, Neola, Sgroi, Piantedosi, Nielsen and Veneziano2021; Nielsen et al., Reference Nielsen, Gee, Hansen, Waghorn, Bell and Leathwick2021). In horses, the most important ongoing complication of this parasitosis is small intestine impaction and intussusception, which often requires surgery with a poor prognosis (Tatz et al., Reference Tatz, Segev, Steinman, Berlin, Milgram and Kelmer2012), but there is no evidence of clinical complications in donkeys.
In horses, ascarid eggs are excreted mainly by foals and yearlings (<1.4 years old) (Hautala et al., Reference Hautala, Näreaho, Kauppinen, Nielsen, Sukura and Rajala-Schultz2019; Scala et al., Reference Scala, Tamponi, Sanna, Predieri, Luisa, Knoll, Sedda, Dessì, Cappai and Varcasia2021), whereas in donkeys a high prevalence of Parascaris spp. can be seen regardless of the age of the animals (Getachew et al., Reference Getachew, Innocent, Trawford, Feseha, Reid and Love2008b, Reference Getachew, Trawford, Feseha and Reid2010). These differences could be justified by the poor farming conditions of the donkeys that may not have developed an acceptable degree of immunity, associated with the lack of suitable nutrition (Getachew et al., Reference Getachew, Trawford, Feseha and Reid2010). A recent paper showed that even if ascarid eggs were reported in all age groups, a significantly higher prevalence was found in younger donkeys confirming that, as in horses, the main contaminators of roundworm eggs are juvenile animals (Buono et al., Reference Buono, Veronesi, Pacifico, Roncoroni, Napoli, Zanzani, Mariani, Neola, Sgroi, Piantedosi, Nielsen and Veneziano2021).
The most common diagnostic method in horses and donkeys is by FEC. However, it is not possible to find eggs during the prepatent period and FEC may not correlate well with the worm burden (Nielsen et al., Reference Nielsen, Baptiste, Tolliver, Collins and Lyons2010). No statistical association has been seen between FEC and adult worms in horses (Fabiani et al., Reference Fabiani, Lyons and Nielsen2016). Transabdominal ultrasound may be used in routine diagnostics, but veterinarians must have sufficient practice to identify adult Parascaris spp. worms in foals (Nielsen et al., Reference Nielsen, Donoghue, Stephens, Stowe, Donecker and Fenger2016).
The control of Parascaris spp. represents a key point in breeding farms and all foals under the age of 6 months should be considered potentially infected and at risk of developing acute clinical signs. Therefore, selective therapy is not recommended for juvenile animals if the stud has a history of Parascaris spp. infection. For this reason, anthelmintic treatments must be administered to horses and donkeys younger than 1 year of age. Moreover, it is important to evaluate the presence of other intestinal parasites (i.e. strongyles, Strongyloides westeri, Anoplocephalidae) and the efficacy of the anthelmintic against Parascaris spp. (Nielsen, Reference Nielsen2016a). The widespread resistance of Parascaris spp. and small strongyles towards MLs and tetrahydropyrimidines (THPs)/benzimidazoles (BZDs), respectively, has complicated the pharmacological control of these helminths considering that in case of mixed parasitic infections, the efficacy of any active agents could be reduced (Molento et al., Reference Molento, Antunes, Bentes and Coles2008; Morris et al., Reference Morris, Colgan, Leathwick and Nielsen2019).
Dictyocaulus arnfieldi
Dictyocaulus arnfieldi is the lungworm parasite of equids that infects the respiratory tract. Animals became infected by ingesting the infective larvae during grazing. The donkeys represent the competent host, and they are permissive of the entire life cycle of this nematode, whereas in horses, D. arnfieldi rarely develops into an adult, although the transmission between horses has been reported (Matthews, Reference Matthews and Lekeux2002).
Donkeys can be infected by a high number of adult helminths without showing clinical signs (Matthews and Burden, Reference Matthews and Burden2013) or only slight hyperpnoea and harsh lung sounds (Matthews, Reference Matthews and Lekeux2002). In donkeys, persistent infections are very common and patent infections can persist for at least 5 years. In this regard, donkeys can act as reservoirs and main pasture contaminators, representing an important risk factor for co-grazing horses (Beelitz et al., Reference Beelitz, Gobel and Gothe1996). Horses may show signs of persistent cough, tachypnoea, chronic pneumonia and pulmonary oedema. Infections can easily develop secondary bacterial infections. In horses, patency is shorter than in donkeys which can range from 6 weeks to 8 months, in foals and older horses, respectively (Matthews, Reference Matthews and Lekeux2002). Furthermore, young horses are at a major risk of infection than adult horses and are also characterized by a higher larval output (Jenkins et al., Reference Jenkins, Backwell, Bellaw, Colpitts, Liboiron, McRuer, Medill, Parker, Shury, Smith, Tschritter, Wagner, Poissant and McLoughlin2020).
Considering the clinical signs that can occur in horses (light coughing), it is advisable to pay attention to those animals that share the same pasture with donkeys. It has been reported that donkeys co-grazing with horses are less infected than those that do not co-graze. Thus, the diagnosis of donkeys that share pasture with horses is essential for controlling this parasite and for reducing the risk of infection in horses (Buono et al., Reference Buono, Veronesi, Pacifico, Roncoroni, Napoli, Zanzani, Mariani, Neola, Sgroi, Piantedosi, Nielsen and Veneziano2021). In infected donkeys, the diagnosis is performed through the finding of first-stage larvae (L1), whether free or inside the eggs, in the feces by modified Baermann technique (Rode and Jorgensen, Reference Rode and Jorgensen1989). Considering that temperature fluctuations during the first 48 h following fecal collection could adversely affect the recovery of L1, it is mandatory to perform the parasitological examination of fresh samples (Rode and Jorgensen, Reference Rode and Jorgensen1989).
Horse lungworm infections do not often reach patency and fecal diagnosis is more difficult than in donkeys. Trans-tracheal aspirates can be useful for demonstrating the increase in eosinophil numbers and endoscopic examination may reveal the presence of larvae in the airways, lymphoid follicular hyperplasia and exudate in the trachea and bronchioles (Dixon et al., Reference Dixon, Railton and McGorum1995). Furthermore, an enzyme-linked immune sorbent assay (ELISA) test can demonstrate the presence of antibodies from 5 weeks after infection, and it could be useful during high-risk seasonal (late winter) infections (Tagesu, Reference Tagesu2018). Resistance to MLs was determined in Dictyocaulus viviparus of cattle (Molento et al., Reference Molento, Depner and Mello2006) but no reports have confirmed the expected high efficacy in donkeys and horses.
Anoplocephala spp.
Tapeworm infection represents a serious worldwide parasitic disease of equids caused by the species Anoplocephala perfoliata, Anoplocephala magna that infect horses and donkeys and Paranoplocephala mamillana that occurs only in horses. These tapeworm species differ from each other in size, location and pathogenicity (Nielsen, Reference Nielsen2016b). Anoplocephala magna and P. mammillana are less frequent and also have an uncertain or marginal pathogenic role (Gasser et al., Reference Gasser, Williamson and Beveridge2005). Some biological behaviour of A. perfoliata includes preferential adhesion sites, consisting of the caecum wall and the ileo–caeco–colic ostium. The parasite has the tendency to cluster with numerous specimens in this region causing serious clinical intestinal disorders (Edwards, Reference Edwards1986; Fogarty, Reference Fogarty1994; Trotz-Williams et al., Reference Trotz-Williams, Physicl-Sheard, McFarlane, Pearl, Wayne Martin and Peregrine2008). The significant association between heavy parasitic burdens (greater than 100 specimens) of A. perfoliata and both medical (i.e. spasmodic) and surgical colics of the ileo–caecal–colic tract (i.e. ileocaecal, caecal–caecal and caecal–colic intussusceptions, ileal impaction) has been demonstrated (Proudman and Edwards, Reference Proudman and Edwards1993, Reference Proudman, French and Trees1998; Reinemeyer and Nielsen, Reference Reinemeyer and Nielsen2009; Veronesi et al., Reference Veronesi, Diaferia and Fioretti2009; Pavone et al., Reference Pavone, Veronesi, Genchi, Piergili Fioretti, Brianti and Mandara2011) in horses. The pathogenesis and the clinical impact of this infection in donkeys are still limited. However, colic might be considered an occasional and exceptional onset of infection in both hosts. Animals in good health can tolerate a high-parasitic load, and tapeworm infections are usually suspected due to vague clinical signs – such as weight loss, the opacity of the coat and generic digestive disorders, such as constipation alternating with moderate diarrhoea.
Anoplocephalidae infections are considered a typical parasitosis of equids on pasture, although infections can occur in stabled animals, through the feeding of forage contaminated with oribatid mites, the intermediate hosts of the life cycle. In most of the developed countries, the prevalence of infection has been increasing over time, due to the absence of efficacy of the most used modern anthelmintics (i.e. IVM and MOX). The parasite also reduces the competitive pressure of further parasites of the digestive tract such as strongyles (Bello and Abell, Reference Bello and Abell1999; Gasser et al., Reference Gasser, Williamson and Beveridge2005). The worldwide prevalence of infection in horses ranges from 18 and 82% with the highest positivity rates observed in humid and rainy areas that favours the development of the intermediate hosts (i.e. Sweden, UK, Germany, etc.) (Fogarty, Reference Fogarty1994). Infection occurs in animals of all ages and patent infections can be described starting between the 16th and 20th weeks of life (Gasser et al., Reference Gasser, Williamson and Beveridge2005). However, there were some reports showing the highest prevalence and intensity of infection in animals younger than 3 years of age (Campigli et al., Reference Campigli, Fichi, Rondolotti, Pellegrini, Tambini, Ming, Traversa and Perrucci2009). In donkeys, epidemiological studies have reported high prevalences in Africa (>80%) (Matthews and Burden, Reference Matthews and Burden2013), and low in Europe (<10%) (Buono et al., Reference Buono, Veronesi, Pacifico, Roncoroni, Napoli, Zanzani, Mariani, Neola, Sgroi, Piantedosi, Nielsen and Veneziano2021).
Tapeworm infection in equids can be detected by direct parasitological methods based on qualitative tools. Differently from the other Cyclophyllidea, the search for proglottids in feces is of scant diagnostic value. The proglottids are rarely detectable since before they are released with feces they are broken down directly in the intestine (Nielsen, Reference Nielsen2016b). The traditional techniques of concentration by flotation show an overall poor sensitivity (between 11 and 40%) for A. perfoliata infection. This is due to the inconstant egg output even in massive infestations (>100 specimens), and cannot be considered predictive of colic risk (Williamson et al., Reference Williamson, Beveridge and Gasser1998; Abbott and Barret, Reference Abbott and Barret2008). To improve the sensibility of detection, specific techniques have been developed in horses that can be used also in donkeys, i.e. Proudman's test (flotation concentration test) (Proudman and Edwards, Reference Proudman and Edwards1992) and the Cornell–Wisconsin test (sediment concentration test) (Egwang and Slocombe, Reference Egwang and Slocombe1982). These techniques can attain sensitivities between 61 and 92% for parasitic loads greater than 20 specimens (Williamson et al., Reference Williamson, Beveridge and Gasser1998). Immuno-diagnostic assays have also been developed and validated in horses for the detection of antibodies (immunoglobulin G) stimulated by somatic or excretory/secretory antigens of A. perfoliata with a mean sensitivity of 68% and a specificity of 95% (Proudman and Trees, Reference Proudman and Trees1996a). A semi-quantitative capture antigen-ELISA test, using a purified 12/13 kDa antigen of A. perfoliata, is available in the UK and USA and can be used both for large-scale epidemiological screening and in the clinic as an early diagnostic test to prevent colic episodes (Proudman and Trees, Reference Proudman and Trees1996b). In addition, a commercially available ELISA test has also been validated for the detection of antibodies in saliva with a sensitivity of 83% and a specificity of 85% (Lightbody et al., Reference Lightbody, Davis and Austin2016, Reference Lightbody, Matthews, Kemp-Symonds, Lambert and Austin2018). Such assay seems to be more useful than those conducted on sera to evaluate the efficacy of drug treatment, since post-treatment antibody levels in saliva decrease faster than in the blood. A coproantigen test in ELISA was also validated to detect the excreted/secreted proteins of A. perfoliata, showing a sensitivity of 74% and specificity of 92% (Kania and Reinemeyer, Reference Kania and Reinemeyer2005) but is not currently available. Neither of these immunological tests has been validated in donkeys but several experiments have been conducted showing good sensibility (Getachew et al., Reference Getachew, Innocent, Proudman, Trawford, Feseha, Reid, Faith and Love2012).
Anthelmintics in horses and donkeys
Donkeys are characterized by the greater activity of some cytochrome p450 isoenzymes than horses, giving them a greater ability to metabolize certain drugs (Peck et al., Reference Peck, Mealey, Matthews and Taylor1997). For this reason, there may be differences in the drugs’ disposition and availability, which may require higher concentrations or shorter dosing intervals than those used in horses to obtain effective drug concentrations for optimum parasite control (Horspool et al., Reference Horspool, Taylor and McKellar1994; Mealey et al., Reference Mealey, Matthews, Peck, Ray and Taylor1997; Grosenbaugh et al., Reference Grosenbaugh, Reinemeyer and Figueiredo2011).
The most common anthelmintic drugs used in equids are BZDs, THPs, MLs and praziquantel (PZQ) (Gokbulut and McKellar, Reference Gokbulut and McKellar2018). Only a few drugs are registered for use in donkeys (none exclusive). Thus, extra-label administration of products registered for horses or ruminants is the norm. Although there is less than a hand-full data on the pharmacokinetics and pharmacodynamics of anthelmintic drugs in donkeys and mules (Gokbulut et al., Reference Gokbulut, Aksit, Smaldone, Mariani and Veneziano2014, Reference Gokbulut, Aksit, Santoro, Roncoroni, Mariani, Buono, Rufrano, Fagiolo and Veneziano2016a), anthelmintic treatment is performed using the same doses and regimens suggested for horses without considering species, breed, nutritional status or individual (height and weight) differences (Lizzaraga et al., Reference Lizzaraga, Sumano and Brumbaugh2004; Veneziano et al., Reference Veneziano, Di Loria, Masucci, Di Palo, Brinati and Gokbulut2011, Reference Veneziano, Galietti, Mariani, Di Loria, Piantedosi, Neola, Guccione and Gokbulut2013) leading to a reduction in effectiveness (Molento et al., Reference Molento, Antunes, Bentes and Coles2008), and an increased risk of toxic signs due to overdosing (Grosenbaugh et al., Reference Grosenbaugh, Reinemeyer and Figueiredo2011). Therefore, there is a great need for more data on the donkey as well as new horse anthelmintic drugs and treatment strategies to support welfare (Senior, Reference Senior2013).
Tetrahydropyrimidines
Pyrantel (PYR) is the only molecule of its class, licensed for horses and is available as salt PYR pamoate (syn. embonate), insoluble in water and as PYR tartrate (soluble in water). PYR pamoate is available as paste or granule formulations, and it is poorly absorbed in the gastrointestinal (GI) tract, which increases its persistence in the intestine (Bjorn et al., Reference Bjorn, Hennessy and Friis1996). The mechanism of action of THP is by binding to nicotinic acetylcholine receptors of the nematode muscle cells causing spastic paralysis and subsequent elimination from the host (Martin and Robertson, Reference Martin and Robertson2007).
A pharmacokinetic study showed that PYR pamoate administered to horses at 13.2 mg kg−1 BW was poorly absorbed and the plasma concentration of the parent drug was very low (C max = 0.09 ± 0.02). Moreover, its persistence in feces was 48 h and the highest dry fecal concentration (1.034 mg g−1) was detected at 24 h (Gokbulut et al., Reference Gokbulut, Nolan and McKellar2001a). In donkeys, PYR pamoate paste formulation at 6.94 mg kg−1 BW showed a lower C max and a smaller area under the curve (AUC) than the granule formulation administered at the same dose (Gokbulut et al., Reference Gokbulut, Aksit, Smaldone, Mariani and Veneziano2014) (Table 2). This difference in plasma levels can be attributed to lower intestinal absorption of the paste rather than granule formulation. The pharmacokinetic parameters of PYR in donkeys are quite different from those reported in horses, showing in the latter a lower concentration and a short mean residence time (MRT) than in donkeys and, consequently a lower bioavailability (Gokbulut et al., Reference Gokbulut, Aksit, Smaldone, Mariani and Veneziano2014). These differences were attributed to a different diet as donkeys were fed with hay while horses were kept in grass pastures. The process promoted a decrease in the gut transit time resulting in a lower bioavailability of PYR in horses (Gokbulut et al., Reference Gokbulut, Nolan and McKellar2001a). Moreover, residues of PYR were found in the feces of donkeys and horses for up to 120 h (Gokbulut et al., Reference Gokbulut, Aksit, Smaldone, Mariani and Veneziano2014) and 48 h (Gokbulut et al., Reference Gokbulut, Nolan and McKellar2001a), respectively.
Table 2. Comparative studies on pharmacokinetic parameters of THPs and BZDs in horses and donkeys

Adapted from Gokbulut and McKellar (Reference Gokbulut and McKellar2018).
C max, peak plasma concentration; T max, time to reach peak plasma concentration; AUClast, area under the (zero moment) curve; AUMClast, area under the first moment curve; MRT, mean residence time; T 1/2, terminal half-life; PYR, pyrantel; FBZ, fenbendazole; FBZSO, fenbendazole sulphoxide; FBZSO2, fenbendazole sulphone; ABZSO, albendazole sulphoxide; ABZSO2, albendazole sulphone; MBZ, mebendazole.
In equids, PYR is licensed at a dosage of 6.6 mg kg−1 BW (Gokbulut and McKellar, Reference Gokbulut and McKellar2018) and it is highly effective against adults of small strongyles, S. vulgaris and Parascaris spp. but shows moderate activity against S. edentatus and Oxyuris equi (Mirck, Reference Mirck, Vanden Bossche, Thienpont and Janssens1985). Moreover, a high dose, twice the nematocidal dose of PYR (13.2 mg kg−1 BW) was effective in controlling A. perfoliata infection (Slocombe, Reference Slocombe1979; Höglund et al., Reference Höglund, Nilsson, Ljunström, Hellander, Osterman Lind and Uggla1998). In donkeys, PYR showed high efficacy against cyathostomins and S. vulgaris (Napoli et al., Reference Napoli, Gaglio, Falsone, Ferrara, Brianti and Giannetto2013; Gokbulut et al., Reference Gokbulut, Aksit, Smaldone, Mariani and Veneziano2014; Buono et al., Reference Buono, Roncoroni, Pacifico, Piantedosi, Neola, Barile, Fagiolo, Várady and Veneziano2018). Although it has been reported that PYR-administered high dose used for intestinal strongyles was effective against tapeworms in horses (Höglund et al., Reference Höglund, Nilsson, Ljunström, Hellander, Osterman Lind and Uggla1998), there are still no studies on the effectiveness of PYR pamoate against cestodes of donkeys.
Benzimidazoles and pro-benzimidazoles
The first anthelmintic drug belonging to the BZDs class licensed in 1961 for horses was thiabendazole (Drudge et al., Reference Drudge, Lyons and Tolliver1981). BZDs are characterized by poor water solubility and are administered as paste or drench formulations in horses (Gokbulut and McKellar, Reference Gokbulut and McKellar2018). BZDs bind to the β-tubulin of microtubules, preventing their polymerization, destroying the cellular structure causing the death of the parasite (Martin, Reference Martin1997).
Fenbendazole (FBZ) represents the most common BZD used for horses that belong to the methylcarbamate group. The pharmacokinetics of FBZ is different in donkeys and horses and influencing its efficacy. FBZ is poorly absorbed from the GI tract of horses, and this would explain why higher concentrations are needed to be effective against migrating larvae and encysted larval stages of cyathostomins (McKellar et al., Reference McKellar, Gokbulut, Muzandu and Benchaoui2002). Following oral administration in donkeys, FBZ and its metabolites, FBZ sulphoxide (oxfendazole – FBZSO) and FBZ sulphone (FBZSO2) were not detected in plasma probably due to the lower absorption and greater fecal excretion when compared to horses (Gokbulut et al., Reference Gokbulut, Akar and McKellar2006). Furthermore, FBZ showed a longer gut transit time in donkeys than in horses (Gokbulut et al., Reference Gokbulut, Akar and McKellar2006) (Table 2).
In horses, FBZ is licensed at a dosage of 7.5 mg kg−1 BW and it is effective against adult large and small strongyles, O. equi. FBZ appears to be the best option for controlling P. equorum in foals, characterized by large ascarid burdens (Reinemeyer and Nielsen, Reference Reinemeyer and Nielsen2017). Moreover, in horses, a 5-day regimen of FBZ at 10 mg kg−1 BW was effective for controlling the larval stage of small strongyles in enteric mucosa (Duncan et al., Reference Duncan, Bairden and Abbott1998). However, a reduced efficacy against early third-stage larvae (EL3), late L3 (LL3) and L4 were reported probably due to the presence of BZD-resistant cyathostomin populations (Reinemeyer et al., Reference Reinemeyer, Prado and Nielsen2015; Bellaw et al., Reference Bellaw, Krebs, Reinemeyer, Norris, Scare, Pagano and Nielsen2018). FBZ is registered for use in donkeys, and it seems to have the same spectra of action as in the horse (Gokbulut and McKellar, Reference Gokbulut and McKellar2018).
In donkeys, following oral administration of FBZ, 10 mg kg−1 BW, the drug was not detected in plasma probably due to the lower absorption and greater fecal excretion of the drug faster than observed in horses (Gokbulut et al., Reference Gokbulut, Akar and McKellar2006). The lack of absorption of FBZ supports its ineffectiveness against D. arnfieldi even when administered at high doses (50 mg kg−1 BW) (Taylor and Craig, Reference Taylor and Craig1993).
Mebendazole (MBZ) is an anthelmintic drug belonging to the methylcarbamate group and pharmacokinetic studies have shown that the peak plasma concentration and AUC were lower than those of albendazole (ABZ); however, T 1/2 and MRT were longer than FBZSO and ABZ when administered at 10 mg kg−1 BW (Gokbulut et al., Reference Gokbulut, Akar and McKellar2006, Reference Gokbulut, Aksit, Santoro, Roncoroni, Mariani, Buono, Rufrano, Fagiolo and Veneziano2016a) (Table 2).
The suggested dose of MBZ is 5–10 mg kg−1 BW for horses (McKellar and Scott, Reference McKellar and Scott1990), and when administered at 8.8 mg kg−1 BW it was effective against large and small strongyles (Colglazier et al., Reference Colglazier, Enzie and Kates1977). In donkeys, MBZ oral paste administered at 10 and 20 mg kg−1 BW was effective against cyathostomins. Moreover, at 10 mg kg−1 BW, there was no residue found in the milk of donkeys, apart from what was observed when MBZ was administered at 20 mg kg−1 BW. The data suggest that MBZ at 10 mg kg−1 BW proved to be effective for controlling cyathostomins in donkeys and its milk-withdrawal period is zero (Gokbulut et al., Reference Gokbulut, Aksit, Santoro, Roncoroni, Mariani, Buono, Rufrano, Fagiolo and Veneziano2016a). Furthermore, MBZ administered at 20 mg kg−1 BW for 5 consecutive days was effective in treating D. arnfieldi infection in donkeys (McKellar and Scott, Reference McKellar and Scott1990).
ABZ belongs to the methylcarbamate group, and it is recommended orally in horses at a dosage of 5 mg kg−1 BW. The pharmacokinetics parameters of ABZ and its metabolites (albendazole sulphoxide – ABZSO and albendazole sulphone – ABZSO2) are shown in Table 2. In experimentally infected ponies, ABZ administered at 50 mg kg−1 BW twice a day for 2 days was effective in controlling S. vulgaris larvae in the cranial mesenteric artery showing few toxic signs in 3 of 11 ponies (Georgi et al., Reference Georgi, Rendano, King, Bianchi and Theodorides1980). Furthermore, when administered at 50 mg kg−1 BW twice a day for 4 days and at 25 mg kg−1 BW 3 times a day for 5 days resulted in a faster larval kill but more toxic symptoms and death occurred in 3 of 6 ponies (Georgi et al., Reference Georgi, Rendano, King, Bianchi and Theodorides1980). An extra-label pellet formulation of ABZ licensed for ruminants and administered to horses at a dose of 7.5 mg kg−1 BW showed a reduced efficacy against small strongyles. However, considering that there are no precise indications on the evaluation of the formulation used, it is not possible to state the certain presence of AR (Salas-Romero et al., Reference Salas-Romero, Gomez-Cabrera, Molento, Lyons, Delgado, González, Arenal and Nielsen2017). ABZ suspension (25 mg mL−1) was administered in a single oral dose at 10 mg kg−1 BW and 2 oral doses 14 days apart at 10 mg kg−1 BW in donkeys and both doses showed high efficacy against adult stages of large and small strongyles until the end of the study period (Imam et al., Reference Imam, Seri, Hassan, Tigani, Zolain and Abakar2010).
Macrocyclic lactones
MLs (avermectins and milbemycins) are a class of natural and semisynthetic drugs of which IVM, MOX, doramectin (DRM) and eprinomectin (EPM) are the most commonly administered in equids (Gokbulut and McKellar, Reference Gokbulut and McKellar2018). MLs are characterized by a high activity against endo- (i.e. nematodes) and ectoparasites (i.e. mites, flies, lice) in humans and animals and thus are also defined as endectocides (Gokbulut and McKellar, Reference Gokbulut and McKellar2018). Apart from that, although IVM and MOX have a similar mechanism of action by binding to glutamate and gammabutyric acid-gated chloride channels, these drugs have profound pharmacological differences (Dent et al., Reference Dent, Davis and Avery1997; Feng et al., Reference Feng, Hayashi, Beech and Prichard2002) and must be further studied in donkeys.
In horses, the kinetic of IVM was evaluated in several studies showing significant differences probably due to the distinct methods of application. A larger AUC and a longer MRT in donkeys than in horses were reported (Gokbulut et al., Reference Gokbulut, Boyacioglu and Karademir2005). These data suggest that in the GI tract of donkeys, IVM had a longer persistence and greater absorption. However, Marriner et al. (Reference Marriner, Mckinnon and Bogan1987) reported a larger AUC and a higher C max in horses than in donkeys and these differences could be due also to diet, breed and anatomical differences between horses and donkeys (Table 3). No pharmacokinetic data of MOX are available in donkeys.
Table 3. Comparative studies on pharmacokinetic parameters of MLs in horses and donkeys

Adapted from Gokbulut and McKellar (Reference Gokbulut and McKellar2018).
C max, peak plasma concentration; T max, time to reach peak plasma concentration; AUClast, area under the (zero moment) curve from time 0 to the last detectable concentration; MRT, mean residence time; T 1/2, terminal half-life; P.O., oral route/per os; T., topical.
In horses, IVM and MOX are registered at 200 and 400 μg kg−1 BW, respectively. Both IVM and MOX, only when used orally in combination with PZQ, are active against tapeworms (Gokbulut and McKellar, Reference Gokbulut and McKellar2018). Although MLs are highly effective in controlling intestinal strongyles they are not effective to control roundworms (Veronesi et al., Reference Veronesi, Piergili Fioretti and Genchi2010). In donkeys, off-label IVM administered at 200 μg kg−1 BW was highly effective for controlling small strongyles (Papini et al., Reference Papini, Orsetti and Sgorbini2020). Even though IVM (200 μg kg−1 BW) and MOX (400 μg kg−1 BW) were effective for controlling intestinal strongyle infection 14 days post-treatment, a shorter egg reappearance period (ERP) was reported for both active drugs in Italy (Buono et al., Reference Buono, Roncoroni, Pacifico, Piantedosi, Neola, Barile, Fagiolo, Várady and Veneziano2018).
IVM and MOX administered at 200 and 400 μg kg−1 BW, respectively, are the drugs of choice for the treatment of D. arnfieldi in horses and donkeys (Lyons et al., Reference Lyons, Drudge and Tolliver1985; Coles et al., Reference Coles, Hillyer, Taylor and Parker1998; Matthews and Burden, Reference Matthews and Burden2013).
DRM following oral administration at 200 μg kg−1 BW showed a high persistence and bioavailability than IVM (Gokbulut et al., Reference Gokbulut, Boyacioglu and Karademir2005) in donkeys, and it also showed a larger AUC and a longer MRT than in horses (Table 3). Furthermore, in horses, DRM administered orally at 200 μg kg−1 BW showed a faster absorption than the injectable 1% formulation administered at the same dose by intramuscular route (Pérez et al., Reference Pérez, Godoy, Palma, Munoz, Arboix and Alvinerie2010).
In horses, DRM administered both orally and intramuscularly at 200 μg kg−1 BW was effective for controlling intestinal strongyles (Pérez et al., Reference Pérez, Godoy, Palma, Munoz, Arboix and Alvinerie2010). In naturally infected horses, DRM following oral administration at 200 μg kg−1 BW was effective against small strongyles with an ERP of 10 weeks (Cirak et al., Reference Cirak, Güleğen, Yildirim and Durmaz2007). In donkeys, DRM administered at 1 mL per 50 kg by subcutaneous injection was effective for controlling small strongyles until day 28 post-treatment (Elmeligy et al., Reference Elmeligy, Abdelbaset, Elsayed, Bayomi, Hafez, Abu-Sheida, El-Khabaz, Hassan, Ghandour and Khalphallah2021).
EPM is the last licensed drug belonging to avermectins (Gokbulut and McKellar, Reference Gokbulut and McKellar2018) that is 2 or 3 times more effective than IVM and yields zero milk-withdrawal time. Therefore, EPM can be used safely in lactating animals (Shoop et al., Reference Shoop, Egerton, Eary, Haines, Michael, Mrozik, Eskola, Fisher, Slayton, Ostlind, Skelly, Fulton, Barth, Costa, Gregory, Campbell, Seward and Turner1996). The pharmacokinetic parameters of EPM in horses and donkeys are shown in Table 3 and the low disposition rate of EPM in the milk of animals allows the use of the drug in milk-producing horses (Gokbulut et al., Reference Gokbulut, Ozuicli, Aksit, Aksoz, Korkut, Yalcinkaya and Cirak2016b) and donkeys (Gokbulut et al., Reference Gokbulut, Di Loria, Gunay, Masucci and Veneziano2011). In donkeys, EPM following pour-on administration (bovine dose – 500 μg kg−1 BW) was effective in eliminating D. arnfieldi larvae for 28 days (Veneziano et al., Reference Veneziano, Di Loria, Masucci, Di Palo, Brinati and Gokbulut2011). Moreover, following topical administration, EPM showed high efficacy against large and small strongyles (Gokbulut et al., Reference Gokbulut, Di Loria, Gunay, Masucci and Veneziano2011).
Praziquantel
PZQ is an isoquinoline and it is licensed both in human and animal medicine for controlling cestodes and trematodes (Gokbulut and McKellar, Reference Gokbulut and McKellar2018). PZQ acts by binding to the parasites’ glutathione S-transferase (McTigue et al., Reference McTigue, Williams and Trainer1995), altering the concentration of intracellular calcium, causing muscle contractions and tegument rupture (Harnett, Reference Harnett1988). There are no data on the pharmacokinetics of PZQ in horses and donkeys but, it is quickly and widely absorbed following administration in humans (Leopold et al., Reference Leopold, Ungethium, Groll, Diekmann, Nowak and Wenger1978).
In horses, PZQ is registered at 1.0 mg kg−1 BW and a PZQ paste of 9% was effective for controlling A. perfoliata, A. magna and A. mamillana (Slocombe et al., Reference Slocombe, Heine, Barutzki and Slacek2007). Moreover, following PZQ administration, the prevalence of cestodes was reduced by 96% until 10 weeks post-treatment (Lyons et al., Reference Lyons, Bellaw, Dorton and Tolliver2017). Similarly, PZQ paste administered orally at 1 mg kg−1 BW was effective for controlling A. perfoliata in donkeys (Getachew et al., Reference Getachew, Innocent, Proudman, Trawford, Feseha, Reid, Faith and Love2013). In equids PZQ is licensed in association with the MLs (IVM and MOX); however, anecdotal data suggest that PZQ should be poorly tolerated in donkeys (Matthews and Burden, Reference Matthews and Burden2013). PYR pamoate paste administered at a dose of 13.2 mg kg−1 BW was safe and highly efficacious for controlling Anoplocephala spp. infection in horses (Marchiondo et al., Reference Marchiondo, White, Smith, Reinemeyer, Dascanio, Johnson and Shugart2006) and, although there are no safe dosage studies in donkeys, it would be safer to administer PYR (at a double dose of 13.2 mg kg−1 BW) than PZQ for controlling Anoplocephala spp.
AR in equids: horses vs donkeys
Resistance is the ability of a worm population to survive treatments generally effective against the same species and stage of infection (Sangster, Reference Sangster1999). Drug resistance was first reported in horses in the 1960s against phenothiazine in small strongyles (Gibson, Reference Gibson1960). Resistance to anthelmintics persists for many years and is transmitted to parasite populations being a genetic-based trait. In horses, the overuse of anthelmintics has led to the development of resistance, especially against small strongyles and Parascaris spp. (Molento, Reference Molento2005; Peregrine et al., Reference Peregrine, Molento, Kaplan and Nielsen2014). The FEC reduction test is the in vivo test for determining the effectiveness of anthelmintic treatment against intestinal strongyles and Parascaris spp. in horses and donkeys and it is based on the percentage of reduction of eggs in the faces after 14 days post-treatment (Nielsen et al., Reference Nielsen, Mittel, Grice, Erskine, Graves, Vaala, Tully, French, Bowman and Kaplan2019). The first report of cyathostomin resistance to PYR in horses was published in 1996 (Chapman et al., Reference Chapman, French, Monahan and Klei1996) and nowadays, resistance to this drug class is very common (Zanet et al., Reference Zanet, Battisti, Labate, Oberto and Ferroglio2021; Nielsen, Reference Nielsen2022). In horses, resistance to THP was evaluated since 2000 in 37 studies and reported in 34 (92%) (Nielsen, Reference Nielsen2022).
Resistance to the BZDs has been commonly reported in the equine industry worldwide (Matthews, Reference Matthews2014) and in wild equids (Kuzmina et al., Reference Kuzmina, Zvegintsova, Yasynetska and Kharchenko2020), showing that resistance against this anthelmintic drug class is not always associated with the intensity of anthelmintic treatments. In horse strongyle infection, resistance to the BZDs has been associated with the polymorphisms of codons 167, 168 and 200 of isotype 1 β-tubulin (Ishii et al., Reference Ishii, Arenal, Felix, Yoshitani, Beech and Molento2017; Özben et al., Reference Özben, von Samson-Himmelstjerna, Freiin von Streit, Wilkes, Hughes and Krücken2022). In horses, since 2000, AR against BZDs has been evaluated in 58 studies and it was reported in all of them (Nielsen, Reference Nielsen2022). Moreover, in horses, multiple resistance to different anthelmintic drug classes has been reported (Flores et al., Reference Flores, Osmari, Ramos, Marques, Ramos, de Avila Botton, Silveira Flores Vogel and Sangioni2020) and it is quite common to find a population of small strongyles resistant both to BZD and THP (Canever et al., Reference Canever, Braga, Boeckh, Grycajuck, Bier and Molento2013). Another minor mutation was described by Ishii et al. (Reference Ishii, Arenal, Felix, Yoshitani, Beech and Molento2017) at codon 172 that deserves further research.
Although MLs are the most commonly administered anthelmintic drugs in horses (Tzelos et al., Reference Tzelos, Morgan, Easton, Hodgkinson and Matthews2019) as in donkeys (Buono et al., Reference Buono, Veronesi, Pacifico, Roncoroni, Napoli, Zanzani, Mariani, Neola, Sgroi, Piantedosi, Nielsen and Veneziano2021), drug resistance is not so common and it may develop in a more complex way – as more genes are involved. However, in the last few years, several studies have reported a reduced efficacy of MLs against small strongyles in horses, both as a reduced efficacy at 14 days post-treatment and as a shortened ERP (Molento et al., Reference Molento, Antunes, Bentes and Coles2008, Reference Molento, Nielsen and Kaplan2012; Tzelos et al., Reference Tzelos, Barbeito, Nielsen, Morgan, Hodgkinson and Matthews2017; Nielsen et al., Reference Nielsen, Banahan and Kaplan2020; Abbas et al., Reference Abbas, Ghafar, Hurley, Bauquier, Beasley, Wilkes, Jacobson, El-Hage, Cudmore, Carrigan, Tennent-Brown, Gauci, Nielsen, Hughes, Beveridge and Jabbar2021) that it was postulated representing the first sign of emerging resistance (Sangster, Reference Sangster1999). For this reason, in the next few years, an increased numbers of reports of AR to MLs are expected worldwide. A recent study showed that a shortened ERP cannot be explained only by the survival of 4th-stage larvae but probably could be associated also with other factors such as the selection of species or strains that accelerate their life cycle. Thus, a shortened ERP could not indicate the development of drug resistance (Nielsen et al., Reference Nielsen, Steuer, Anderson, Gavriliuc, Carpenter, Redman, Gilleard, Reinemeyer and Poissant2022).
Since 2000, resistance against MLs in horses has been evaluated in 57 studies and reported in 13 (23%) (Nielsen, Reference Nielsen2022). In several nematode species, MLs resistance is associated with a group of genes that encode adenosine triphosphate-binding cassette transporters (Tydén et al., Reference Tydén, Skarin and Hoglund2014; Raza et al., Reference Raza, Kopp, Jabbar and Kotze2015).
In donkeys, drug resistance has not been reported as commonly as in horses and few clinical trials have been performed for evaluating the efficacy of the most common anthelmintic drugs (FBZ, PYR and MLs) (Matthews and Burden, Reference Matthews and Burden2013). Resistance to PYR was reported in 2 donkey farms in the UK (Lawson et al., Reference Lawson, Burden and Elsheikha2015); furthermore, in 1 of these farms, a suspected resistance to MOX was reported 10 years earlier after continuous use of the cattle formulation (Trawford et al., Reference Trawford, Burden and Hodgkinson2005). However, considering that donkeys were treated orally using an injectable formulation licensed for cattle, the data reported for MOX probably did not confirm the presence of AR. Recently, a population of small strongyles resistant to FBZ and PYR was reported in an Italian donkey farm, also associated with a reduction of ERP for IVM and MOX (Buono et al., Reference Buono, Roncoroni, Pacifico, Piantedosi, Neola, Barile, Fagiolo, Várady and Veneziano2018).
In horses, the first report of MLs resistance in Parascaris spp. was described by Boersema et al. (Reference Boersema, Eysker and Nas2002) in the Netherlands. Nowadays, AR to MLs have been reported in 29 out of 29 studies worldwide, while resistance against THP and BZDs was reported in 4 out of 16 and 3 out of 13 studies, respectively (Nielsen, Reference Nielsen2022). The sporadic resistance of Parascaris spp. against BZDs and THP should be due to the limited studies on the effectiveness of these anthelmintic drug classes. Thus, drug resistance should be more common than those reported in the literature, as also suggested in some studies (Martin et al., Reference Martin, Hoglund, Bergstrom, Lindsjo and Tydén2018, Reference Martin, Halvarsson, Delhomme, Höglund and Tydén2021b; Hautala et al., Reference Hautala, Näreaho, Kauppinen, Nielsen, Sukura and Rajala-Schultz2019).
AR of P. univalens against BZDs is not associated with SNP suggesting that the mechanism of AR in this parasite is different from those reported for intestinal strongyles (Martin et al., Reference Martin, Halvarsson, Delhomme, Höglund and Tydén2021b). The lack of efficacy of IVM, MOX and PYR has been reported also in donkeys (Matthews and Burden, Reference Matthews and Burden2013). Studies on anthelmintic efficacy in donkeys are reported in Table 4.
Table 4. Studies in donkeys reporting anthelmintic efficacy – FECRT (14 days post-treatment)
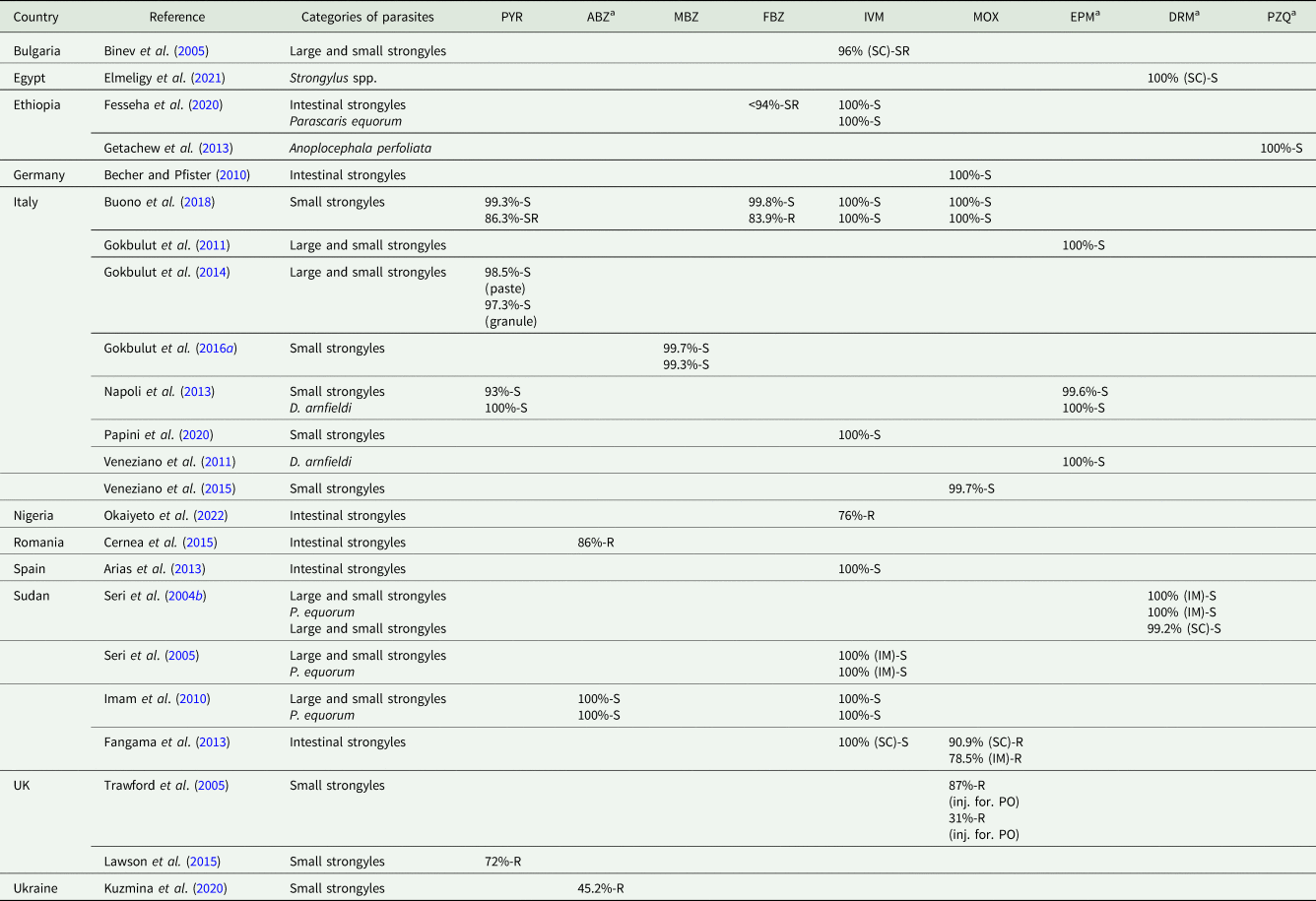
PYR, pyrantel pamoate; ABZ, albendazole; MBZ, mebendazole; FBZ, fenbendazole; IVM, ivermectin; MOX, moxidectin; EPM, eprinomectin; DRM, doramectin; PZQ, praziquantel; SC, subcutaneous administration; IM, intramuscular administration; inj. for., injectable formulation; PO, per os.
According to American Association of Equine Practitioners Parasite Control Guidelines cut-off (Nielsen et al., Reference Nielsen, Mittel, Grice, Erskine, Graves, Vaala, Tully, French, Bowman and Kaplan2019), the cut-off values used to interpret the results of FECRT were the following: PYR susceptible (S) >90%, suspected resistance (SR) 85–90%, resistant (R) <85%; FBZ susceptible (S) >95%, suspected resistance (SR) 90–95%, resistant (R) <90%; IVM/MOX susceptible (S) >98%, suspected resistance (SR) 95–98%, resistant (R) <95%.
a For these anthelmintic drugs, cut-off values are not suggested in AAEP Guidelines.
Conclusions
The host–parasite relationship is a complex process in equids that still needs much attention. Although donkeys and horses are closely related species and share practically the same parasite fauna, they are divergent in their physiological and pharmacological (absorption and distribution of drugs) characteristics. Some features such as breed, geographic location/climate, parasite challenge and intensity, immune response and control methods including chemical use shall be regarded as a priority to help solve important parasitic infections in both species. Target selective treatment can also be adopted, looking mainly for clinical signs, performance (body growth and withers height) and to FEC. Once adopted, the selective strategy may help preserve drug effectiveness and the welfare of both horses and donkeys. Horses and donkeys are ‘different cousins’; for this reason, precise farm management and parasite control programme must take these differences into account.
Author's contribution
F. B. conceived and designed the study and wrote the first draft of the manuscript. V. V., F. V. and M. B. M. conceived and designed the study and wrote and reviewed the manuscript.
Financial support
This research received no specific grant from any funding agency, commercial or not-for-profit sectors.
Conflict of interest
The authors declare there is no conflict of interest.