- EFSA
-
European Food Safety Authority
- GI
-
glycaemic index
- SACN
-
Scientific Advisory Committee on Nutrition
Carbohydrate metabolism
Carbohydrate is the major macronutrient source of energy in most human diets, contributing 40–55 % of total energy intake. This reliance on carbohydrate as a fuel source is due in part to a need for the daily provision of carbohydrate, in the form of glucose, to support brain function as well as to provide an energy source for red blood cells, the renal medulla and a variety of other cells and tissues. Many of these cells and tissues, in particular the brain, require a continuous supply of glucose as a fuel. This requirement is met by the breakdown of stored liver glycogen together with hepatic and renal gluconeogenesis, which combine to release glucose into the bloodstream during periods of fasting (e.g. during the night). This should be contrasted with the situation after meals, where the influx of glucose and other sugars from the gut is accompanied by endocrine responses (in particular, a rise in insulin), which inhibit glycogen breakdown and gluconeogenesis, stimulate glycogen storage in liver and muscle and increase the use of glucose as an oxidative fuel source in tissues, which were utilising fatty acids as a fuel during fasting (e.g. skeletal muscle). A summary of the quantitative aspects of the associated glucose fluxes in the body is provided by Frayn( Reference Frayn 1 ), and the brain glucose utilisation of 6 g/h translates into a carbohydrate requirement of 144 g/d for the average adult simply to support brain function. Thus, when glucose needs for other cells and tissues and muscle contraction are included, the physiological requirement for carbohydrate is approximately 200 g/d for the average adult.
A number of endocrine mechanisms exist to regulate glucose metabolism, ensuring glucose supply is sustained during fasting and glucose storage and utilisation are regulated after meals (Fig. 1). If these regulatory mechanisms work effectively then the perturbations in blood glucose are modest, but if the endocrine response is inadequate or excessive, or if receptor activation is abnormal, then the major impairments in regulation can occur. The most common situation for this to occur is in people with insulin resistance, where there is reduced sensitivity to insulin, so that postprandial blood glucose levels are substantially elevated. In some people, such insulin resistance can be the precursor to the eventual development of type 2 diabetes, where insulin secretion becomes inadequate to overcome the tissue insulin resistance, and postprandial as well as fasting blood glucose levels are markedly elevated. In addition to being used as a fuel source for energy metabolism, dietary carbohydrate can also be used in fat synthesis and storage in the body. Glucose as a precursor of glycerol-3-phosphate is essential for the re-esterification of dietary fatty acids prior to their storage as TAG in adipose tissue and muscle, and in some situations glucose can also be used for de novo lipogenesis (i.e. fatty acid synthesis) in adipose tissue. Under normal dietary conditions where fat is more than 20 % of dietary energy, the quantitative significance of such lipogenesis is small. However, dietary carbohydrate is used for hepatic de novo lipogenesis leading to incorporation of the resulting fatty acids in hepatic VLDL, with this then contributing to plasma TAG levels( Reference Parks and Hellerstein 2 ), although this is of minor quantitative significance accounting for <5 % of VLDL TAG with normal dietary carbohydrate content in people in energy balance.
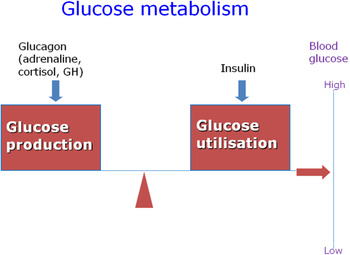
Fig. 1. (colour online) Diagrammatic representation of the balance between glucose production and utilisation, and the main hormonal regulatory factors. GH, growth hormone.
It has been known for some time that high dietary carbohydrate intakes are associated with elevations in plasma TAG and reduced HDL-cholesterol concentrations in inactive older individuals( Reference Jeppesen, Schaaf and Jones 3 ), and thus may contribute to increased risk of CVD. Such an effect of dietary carbohydrate is much smaller in physically active individuals, which may be partly due to the improved insulin sensitivity associated with increased activity, but may also be due to the effects of increased muscle glycogen turnover leading to reduced availability of carbohydrate for fat synthesis.
Dietary carbohydrates
Food sources of carbohydrates can be distinguished either on a structural/chemical basis, or in relation to functional aspects. The current structural/chemical characterisation recognises monosaccharides, disaccharides, oligosaccharides and polysaccharides as the four main categories of carbohydrates, which may be readily available for human metabolism( Reference Cummings and Stephen 4 ). In addition, the various types of dietary fibre, which vary in terms of chemical and structural characteristics, also contribute to the total carbohydrate content of the diet. The dietary monosaccharides consist of glucose, fructose and galactose, while the commonest disaccharides are sucrose and lactose. Oligosaccharides have between three and nine monosaccharide molecules and are exemplified by fructo-oligosaccharide and galacto-oligosaccharide, while polysaccharides have ten or more monosaccharide molecules with the main dietary source being starch. There are a number of different definitions of dietary fibre, ranging from non-starch polysaccharides (essentially plant cell wall material) plus soluble fibre, up to all non-digested dietary carbohydrates. At present the UK definition remains as described originally by the Committee on Medial Aspects of Food and Nutrition Policy in 1997, namely non-starch polysaccharides plus soluble fibre, with the caveat that in order to be regarded as fibre the material in question must be demonstrated to have a physiological effect on the person (not just the colonic bacteria)( 5 ). The current Scientific Advisory Committee on Nutrition (SACN) review of carbohydrates in the diet, which is expected to publish its report in 2014, will consider the evidence relating to whether other components of foods and drink could be included in the category of dietary fibre.
The functional basis of categorising dietary carbohydrate is related to whether or not the foods in question raise blood glucose, i.e. glycaemic v. non-glycaemic carbohydrates, or in the case of the glycaemic carbohydrates the extent to which they increase blood glucose concentration. The latter is known as the glycaemic index (GI) and was first described by Jenkins et al.( Reference Jenkins, Wolever and Taylor 6 ) as a means of characterising carbohydrates to help people with diabetes manipulate their dietary intake in order to manage their diabetes. This approach has been extended and applied to the general population and there is now great interest in the possibility that lower GI foods may provide benefit in terms of reducing disease risk, possibly by an associated reduction in insulin response and altered lipid and carbohydrate metabolism and it has been suggested that food labelling should include the GI in order to help people reduce their disease risk( Reference Chiu, Liu and Willett 7 ). Again the SACN Carbohydrates report is considering this issue and will provide an up-to-date overview in 2014.
Potential effects of carbohydrate on cardiovascular risk
The concerns over dietary carbohydrates potentially increasing CVD risk are linked to the possible effects on blood lipids, in particular TAG on ectopic fat deposition (particularly in muscle and liver cells) and on insulin resistance. There is also a concern that certain types of carbohydrate, or carbohydrate-rich foods, may contribute to such risks. Thus, there is a belief that refined grains represent a greater risk to health than whole grains, but the problem with this is that the studies performed so far have not been able to investigate whether it is the carbohydrate per se, or the other components (fibre and phytochemicals) that are retained in the whole-grain food but lost in refining, which is responsible for any impact on health risk. As far as types of carbohydrate are concerned, there is a major concern over differences between the monosaccharides, particularly between glucose and fructose. These molecules are consumed as the individual monosaccharides, combined together as sucrose, in some of the oligosaccharides, or in the case of glucose, in starch as well. There are biochemical differences in the way glucose and fructose are handled by the body, with fructose not eliciting an insulin response and being taken up and metabolised by the liver. It is theoretically possible that fructose will stimulate more de novo lipogenesis in the liver and give rise to greater increases in VLDL TAG levels, although the evidence for such an effect at normal dietary intakes is rather weak. The average UK diet has a total fructose content (from sucrose and from fresh fruit and fruit juices) of approximately 10 % of energy, based on the low-income diet and Nutrition Survey reporting non-milk extrinsic sugars intake of 14·5 % in men( Reference Nelson, Erens and Bates 8 ) with an assumption that half of this could be sucrose and half fructose as a monosaccharide. Clearly if the sucrose contribution is higher, then the total fructose intake will be less. This is of importance because a recent study( Reference Johnston, Stephenson and Crossland 9 ) showed that providing 25 % of dietary energy as fructose or glucose to overweight men for 2 weeks, fed to achieve energy balance, did not have any effect on plasma TAG levels, and also did not change liver or muscle fat content. There is concern that high fructose corn syrup may have additional harmful effects on cardiovascular risk in comparison with sucrose, but there is little evidence that it is biochemically different from sucrose( Reference Yu, Lowndes and Rippe 10 ) and the current use of high fructose corn syrup in the UK is very low.
Current public health advice relating to carbohydrates and CVD risk
There are a wide number of sources of public health advice relating to dietary carbohydrates and disease risk. Those of particular interest to the present review are provided by the European Food Safety Authority (EFSA)( 11 ), the US Dietary Guidelines( 12 ) and the German Nutrition Society( Reference Hauner, Bechthold and Boeing 13 ). The EFSA advice concludes that the consumption of 25 g fibre/d is sufficient to promote normal laxation. It acknowledges that all the evidence for beneficial effects of fibre is based on fibre-rich foods, but then defines dietary fibre as all non-digestible carbohydrates (plus lignin). The problem with such a definition is that the novel ‘fibres’ that are now appearing in food products did not form a part of the diets for which such beneficial associations or effects were identified, yet some food manufacturers are claiming that the benefits are linked to all ‘fibres’. There may also be beneficial effects of fibre as far as blood pressure and plasma lipids are concerned, although the magnitude of such effects in relation to normal dietary intakes of fibre is unclear. The EFSA opinion advises that carbohydrate should provide 45–60 % of energy, for both adults and children, and that there is insufficient evidence to set an upper limit for the intake of added sugars. Given the subsequent WHO review of sugars and obesity (see later), which claimed to show potentially harmful effects of a high sugar intake, such a conclusion is clearly somewhat controversial. There is also a conclusion that reducing the GI of diets may have a favourable effect on plasma lipids; the evidence of a beneficial effect on body weight is inconclusive. The advice provided in the Dietary Guidelines for Americans( 12 ) includes the view that healthy diets are high in carbohydrates, including that the acceptable range of contribution of carbohydrate to energy intake is 45–65 % of energy, with the higher values limited for those who are more physically active, and the lower values for those with lower energy requirements or on low-energy intakes. In contrast to the EFSA opinion, the US advice is that the maximum contribution that added sugars should make to energy intake should be 25 %. There is also an encouragement to choose fibre-rich foods (e.g. whole grains, vegetables, fruits and pulses) as staples in the diet.
The German Nutrition Society provides public health nutritional advice to the German government and so has a similar function to SACN in the UK. Their recent review of carbohydrates and health considered the scientific literature from the past 35 years, and included original articles, systematic literature reviews and meta-analyses. Unfortunately they did not conduct their own systematic review of the evidence available but rather took the information provided by the existing reviews, which has the potential to overemphasise certain aspects as there could be multiple counting of the same original research in a number of published systematic reviews. The overall conclusions were that a high carbohydrate intake, when substituting for total fat and saturated fat reduces the serum concentrations of total, LDL and HDL cholesterol, whereas if it substitutes for PUFA there is an increase in total and LDL cholesterol and a reduction in HDL cholesterol. There have been some suggestions that replacing saturated fat by carbohydrate has detrimental effects on cardiovascular risk( Reference Siri-Tarino, Sun and Hu 14 ), but this is based predominantly on cohort/observational studies and requires further assessment via randomised controlled trials.
The German Nutrition Society report also concluded that a high carbohydrate intake promotes an increase in serum TAG concentration and that a high consumption of sugar-sweetened beverages increases the risk of obesity and type 2 diabetes mellitus. The latter is of particular concern at present as it is echoed by a recent WHO sponsored review of sugar and obesity( Reference Te Morenga, Mallard and Mann 15 ), although the conclusions produced by the latter are not as definitive as they might first appear (see later). The German Nutrition Society review also concluded that a high fibre intake, predominantly from whole-grain foods, reduced the risk of obesity, type 2 diabetes, CVD and colorectal cancer although the evidence for some associations was stronger than for others. This is generally consistent with other opinions, although it will be worth waiting until the SACN Carbohydrate report is produced before drawing overall conclusions.
The WHO sponsored systematic review of sugars and body weight/obesity performed by Te Morenga et al.( Reference Te Morenga, Mallard and Mann 15 ) is interesting and worthy of further consideration in relation to the potential health problems associated with dietary carbohydrates. A technical aspect of the systematic reviews performed is that for some of the associations studied there was marked heterogeneity between studies, which some authors would regard as indicating a lack of reliability in the studies to the extent that calculating an overall effect and meta-analysis is unsafe. The report also calculated meta-analyses for topics where there were only two studies when other authors would expect at least three studies to enable a meta-analysis to be valid. The main observations from this review are that in studies on adults consuming ad libitum diets, reducing the sucrose content of the diet is associated with a reduction in body weight (of approximately 0·8 kg). However, this is clearly linked to changes in energy intake because if the sucrose is reduced by substitution for another carbohydrate source then there is no effect on body weight. Thus, there is no evidence from this review that specific metabolic effects of sucrose or fructose per se are linked to body weight change, but rather that overconsumption of energy is the driver for weight gain, which is not at all surprising. One aspect of this review certainly raises some concerns and needs further examination, which is the potential problem of weight gain associated with sugar-sweetened beverage consumption in children. This observation is consistent with recent results provided by de Ruyter et al.( Reference de Ruyter, Olthof and Seidell 16 ) who studied the effects of sugar-free compared with sugar-sweetened drinks in children in a randomised trial over an 18-month period. The children were aged between 5 and 12 years and so were clearly growing. Overall there was a smaller increase in weight (approximately 1 kg less) and a smaller rise in BMI in the children consuming the sugar-free drinks. This was a very impressive study and illustrates a potential problem, which requires further consideration, although at this stage it is not clear whether the effects observed are illustrative of the sugar-free drinks being beneficial as far as weight gain and growth are concerned, or the sugar-sweetened drinks being detrimental in causing undesirable weight gain( Reference Siri-Tarino, Sun and Hu 14 ). Further work is needed to investigate the potential for sugar-sweetened beverages, and probably other energy containing drinks, to lead to an increase in total energy intake and potential weight gain.
Fructose
The German Nutrition Society review( Reference Hauner, Bechthold and Boeing 13 ) concluded that there was no association between a fructose intake of <100 g/d and the fasting plasma TAG concentration, or between a fructose intake of <50 g/d and the postprandial plasma TAG concentration. Higher fructose intakes, between 100 and 350 g/d, did appear to be associated with increased fasting plasma TAG levels. However, such a conclusion, which they judged as ‘convincing’, may need to be modified to include consideration of the state-of-energy balance of the individual. Providing 25 % of energy as fructose or glucose in overweight men with fasting insulin resistance did not affect fasting TAG concentrations when they were fed at energy balance. This approximated to 150 g/d of either fructose or glucose, in pure form, and was consumed as drinks. It was only when the fructose or glucose was consumed in addition to an ad libitum diet, i.e. in an overfed state, that plasma TAG levels increased, and even then the effect was exactly the same for glucose and fructose( Reference Johnston, Stephenson and Crossland 9 ). It was also observed that when the subjects were fed at energy balance with 25 % of energy from fructose or glucose for 2 weeks there were no increases in liver fat or intramuscular fat content. By contrast, overfeeding with fructose or glucose for 2 weeks increased fat content of liver and muscle, with no difference between fructose and glucose.
Future aspects
It is clear that carbohydrates are an essential part of the diet, needed to provide energy for the nervous system. This is part of the basis of the bulk of current public health advice, which includes approximately 50 % of the total energy intake being derived from carbohydrate. It must also be recognised that some nutritional epidemiologists do not support this view and argue for lower carbohydrate intakes, although this is based on cohort type studies rather than randomised controlled trials. There is a clear difference at present between the advice offered by EFSA, and that from the German Nutrition Society, regarding GI, glycaemic load, sugar-sweetened beverages and disease risk. Some of these differences of opinion may be resolved by future expert reports, but it is also likely that further empirical research will be needed to clarify some of the issues. Any future studies will need to give full consideration to the challenges of designing well-controlled experiments that are capable of answering the questions being posed. The problem with nutritional studies using food is that in order to alter the content of one macronutrient it is necessary to change at least one of the other two if energy balance is to be achieved. Moreover, if for example the carbohydrate content of a diet is reduced by removing a carbohydrate-rich food, then there will also be changes in micronutrient and possibly phytochemical intake due to such foods having a mixture of components contained in them. Such issues clearly also apply to cohort studies and are extremely difficult to control for in a biological sense, especially if the dietary tools used to assess nutrient intake are not sufficiently detailed to allow such minor nutrients to be evaluated.
Acknowledgements
None.
Financial Support
None.
Conflicts of Interest
The author is a member of Public Health England's Scientific Advisory Committee on Nutrition, The European Scientific Advisory Council for Coca Cola Europe, The Nutrition Advisory Board for Mars Europe and the Mars Scientific Advisory Council for Mars, Inc.
Authorship
The author was solely responsible for all aspects of preparation of this paper.