Introduction
Gran Canaria is an Atlantic island of the Canarian archipelago (27°57′31″N, 15°35′33″W), which belongs to Spanish Macaronesia. The island has a land area of just 1560 km2 but reaches 1956 m at the highest point and contains a very diverse ecosystem for its relatively small size. Fifteen endemic species of reptile are found on the Canary Islands, but no snakes.
The introduction of foreign species poses one of the main threats to global biodiversity and ecosystem conservation and its effects are magnified on islands due to ecosystem isolation and high numbers of endemic species or subspecies (Carroll, Reference Carroll2007; Bezerra-Santos et al., Reference Bezerra-Santos, Mendoza-Roldan, Thompson, Dantas-Torres and Otranto2021). This effect has been observed on Gran Canaria where the introduced California kingsnake (Lampropeltis californiae) has resulted in a decrease in numbers of the endemic and endangered Gran Canaria giant lizard (Gallotia stehlini) in the areas where the snakes have been established the longest – a problem that seems to be getting worse (Gallo-Barneto et al., Reference Gallo-Barneto, Cabrera-Pérez, Peña-Estevez, Patiño-Martínez and Monzón-Argüello2016).
The California kingsnake is native from the southwestern USA to the northwestern part of Mexico (Fig. 1) and can be found in a wide range of habitats from forests, rocky areas, coastal, urban and suburban areas, deserts and lakes (Hubbs, Reference Hubbs2009). The snake's natural diet is equally wide and includes lizards, geckoes, small turtles and birds, other snakes, skinks and rats among others. This species of snake is generally harmless to humans and has become popular as a pet (Hubbs, Reference Hubbs2009). The invasion in Gran Canaria is presumed to be due to accidental escapes or through the release of pet snakes, since at least two of the four populations on the island were established by different groups of captive-bred individuals (Monzón-Argüello et al., Reference Monzón-Argüello, Patiño-Martínez, Christiansen, Gallo-Barneto, Cabrera-Pérez, Peña-Estévez, López-Jurado and Lee2015). The general lack of natural predators, a diet based on at least three endemic reptiles; Gran Canaria giant lizard (Gallotia stehlini), Boettger's wall gecko (Tarentola boettgeri boettgeri) and Gran Canaria skink (Chalcides sexlineatus) and rats, together with its burrowing habits, have firmly established this species as a significant ecological problem (Monzón-Argüello et al., Reference Monzón-Argüello, Patiño-Martínez, Christiansen, Gallo-Barneto, Cabrera-Pérez, Peña-Estévez, López-Jurado and Lee2015).
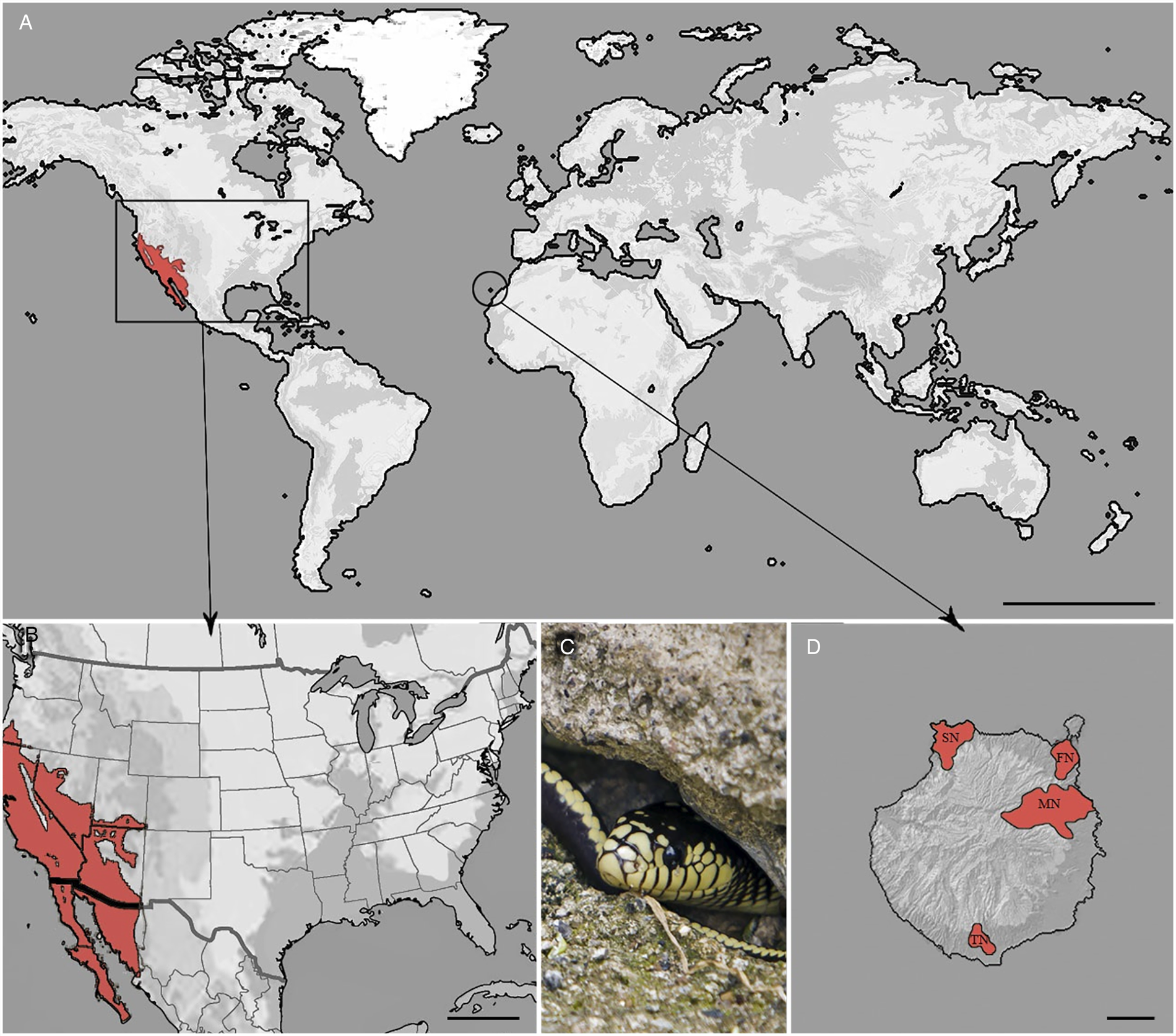
Fig. 1. (A) Distribution of California kingsnake in the world. Scale bar = 5000 km. (B) Native locality. Scale bar = 500 km. (C) An adult snake from Gran Canaria. (D) Populations of this invasive reptile in Gran Canaria, Canary Islands, Spain. Scale bar = 10 km. MN, main nucleus; SN, secondary nucleus; TN, tertiary nucleus; FN, fourth nucleus.
Invasive species pose a direct threat by preying on native fauna, but they can also harbour new parasites or other pathogens that may cause additional damage to the local environment (Taraschewski, Reference Taraschewski2006). Moreover, local parasites or pathogens can infect these exotic invaders and may be more harmful to their new (possibly naïve) hosts, than to their native counterparts (Kelehear and Jones, Reference Kelehear and Jones2010).
Zoonotic parasites, such as Raillietiella sp. (Pentastomida) which can cause abdominal lesions in humans due to the migration of their larvae and nymphs, have been reported in Lampropeltis getula in the USA (Ali et al., Reference Ali, Riley and Self1985; Tappe et al., Reference Tappe, Sulyok, Riu, Rózsa, Bodó, Schoen, Birgit, Babocsay and Hardi2016; Mendoza-Roldán et al., Reference Mendoza-Roldán, Modry and Otranto2020). Their presence has already been demonstrated in Gallotia lizards from the islet of Alegranza (Abreu-Acosta et al., Reference Abreu-Acosta, Foronda Rodriguez, Valladares and Casanova2006), in the eastern Canarian archipelago. If California kingsnakes are able to introduce this pentastomid genus into the island, they could also infect the Gran Canaria giant lizard, as well as potentially humans, and maintain the infection for many generations.
Despite its popularity as a pet, few parasitological studies have been carried out on the California kingsnake, even those kept in zoological collections. Two reports, totalling 11 individuals, describe only protozoan parasites (Van Peenen and Birdwell, Reference Van Peenen and Birdwell1968; Xiao et al., Reference Xiao, Ryan, Graczyk, Limor, Li, Kombert, Junge, Sulaiman, Zhou, Arrowood, Koudela, Modrý and Lal2004).
This is the first study that describes the lesions caused by helminth parasites in California kingsnakes on Gran Canaria and the trichinoscope (compression plates) is described as a useful tool for the detection of larvae. The possible biological hazard for local fauna, and potentially humans, due to the introduction of foreign parasites is discussed.
Material and methods
On Gran Canaria, the California kingsnake is located in three distinct nuclei (Fig. 1); the main nucleus (MN), characterized by a wetland area with abundant flora and fauna in the center-east of the island; the secondary nucleus (SN), a very dry and steep location with characteristic Tabaibal-Cardonal flora, in the northwest, and the tertiary nucleus (TN), with similar climatic conditions to the secondary, in the south. A fourth nucleus has recently been identified in the northeast, surrounding the capital city of Las Palmas de Gran Canaria.
Between 2016 and 2018, snakes from the main and secondary nucleus, captured under the extermination plan approved by the Canary Islands government, were necropsied at the Faculty of Veterinary Science of the University of Las Palmas de Gran Canaria following standard procedures (Farris et al., Reference Farris, Squires, Ridgley, Lavergne, Serota and Mazzotti2013). Due to the few individuals captured, in both cases less than five, the tertiary and fourth nuclei were not included in this study. Biometrical parameters of weight, length, fat weight and sex were recorded. The infested tissues from the animals were fixed in 10% neutral-buffered formalin and routinely processed to paraffin blocks, serially sectioned at 5 μm and stained with hematoxylin and eosin.
Routine fecal examinations were performed following standard methods (Zajac and Conboy, Reference Zajac, Conboy, Zajac and Conboy2012) and detailed notes made on the appearance and number of any gross pathological changes. Gross lesions were further assessed microscopically using compression plates (trichinoscopy). The results are given as prevalence, mean intensity and abundance (Bush et al., Reference Bush, Lafferty, Lotz and Shostak1997).
The parasites were extracted with the help of mounting needles and identified in temporary mounts with saline solution following the keys cited in the bibliography for cestodes (Witenberg, Reference Witenberg1932; Joyeux and Baer, Reference Joyeux and Baer1936; Ryšavý, Reference Ryšavý1973; Jones, Reference Jones, Khalil, Jones and Bray1994), nematodes (Chitwood and Wehr, Reference Chitwood and Wehr1934; Moravec et al., Reference Moravec, Baruš and Ryšavý1987; Anderson, Reference Anderson2000; Willmot and Chabaud, Reference Willmot, Chabaud, Anderson, Chabaud and Wilmott2009; Kelehear and Jones, Reference Kelehear and Jones2010) and Acari (Evans, Reference Evans1955; Lindquist et al., Reference Lindquist, Krantz, Walter, Krantz and Walter2009; Moraza et al., Reference Moraza, Irwin, Godinho, Baird and Goüy De Bellocq2009).
Statistical analysis was performed with SPSS v. 24.0 (IBM SPSS Corp., Chicago, Illinois, USA). The parasite number variation was tested using the parametric Student t-test for each population, the correlations between both weight/length and parasite number were assessed by means of Pearson or Spearman correlation tests, after testing for normality. P values <0.05 were considered to be statistically significant.
Results
Biological sample data: ecological indices and correlations
In total, 108 snakes were examined, divided into two groups: Group A; the first 44 samples collected, in which coprological and postmortem examinations were performed using a stereoscopic microscope, and group B; the following 64 samples, in which the postmortem examinations were enhanced with the aid of a trichinoscope (Table 1). The biometrical parameters and sex by nuclei are represented in Table 2.
Table 1. Ecological indices for the larval parasites found in the main (MN) and secondary nucleus (SN)

a Unable to calculate.
Table 2. Sample biometrical parameters

ML, mean length; MW, mean weight; FW, mean fat weight; F%, mean fat percentage of the body weight; MN, main nucleus; SN, secondary nucleus; UN, undetermined sex and/or nuclei.
In group A snakes, assessed macroscopically, 59.1% had parasitic cysts, with 25.0% containing cysts in the stomach and 45.5% in the small intestine (Figs 2A and C). 80.0% of SN snakes harboured these cysts and 55.2% from the MN.

Fig. 2. (A) Larval nematode encysted in the gastric wall of a snake. Scale bar = 200 μm. (B) Gross appearance of a partly opened lung, showing multiple granulomas (white arrows). Scale bar = 10 mm. (C) Larval tapeworms encysted in the intestinal wall. Scale bar = 1 mm. (D) Gross image of granulomas on the gastric (top, white arrows) and intestinal (bottom, black arrow) serosa. Scale bar = 10 mm.
By contrast, group B, tested by trichinoscopy, revealed a prevalence of 98.4% with cysts – 100% of SN snakes and 96.9% of those from the MN. These cysts microscopically corresponded to cestode cysticercoids and nematode larvae. In summary, almost 30% more cysts were observed with trichinoscopy than by gross examination alone.
No significant differences in parasitic burden or prevalence were found between sex, colour and line patterns.
The prevalence of helminths was estimated using group B. No differences in cestode prevalence were observed between the MN and the SN (80.6 and 83.3% respectively). However, nematode prevalence was markedly different; 90.0% in the SN and 69.7% in the MN.
The two nuclei showed a significant difference in the average number of helminths per individual snake (Student t-test, P = 0.008), but there was no significant difference in numbers of cestodes between the two nuclei (P = 0.307; Mann–Whitney's U test).
In terms of intensity and abundance, the SN showed the highest mean nematode intensity and abundance, and the highest individual infestation (186 ± 306.5; 167.4 ± 296 and 492 larvae respectively). However, there were few differences between the mean intensity and abundance of tapeworms in the two nuclei. Highest tapeworm intensity was found in the MN.
The anatomic distribution of the helminths was not equal; nematodes were concentrated in the stomach, reducing in prevalence and abundance from stomach to large intestine (73.4% stomach, 67.2% small intestine and 42.2% large intestine). Similarly, cestodes were concentrated in the small intestine and reduced in numbers in the large intestine, with no records of cysticercoids in the stomach (81.0% small intestine, 51.6% large intestine and 0% stomach). This anatomic distribution was recorded in both populations studied. No correlations were found between the number of cestodes and the length of the snakes (ρ = −0.144, P = 0.448; Spearman ρ test for the SN and ρ = −0.192, P = 0.285; Spearman ρ test for the MN); however, the number of nematodes showed a positive correlation with the length of the snakes (P = 0.022 for the SN and P = 0.008 for the MN).
No significant correlations were found between the fat percentage of the body weight or fat weight with parasitic burden.
Coprological examination
Just one snake out of the 44 (group A) contained parasite eggs and larvae in its feces compatible with rhabditid nematodes which, in addition to the observation of granulomatous lesions in the lung (Figs 2B, 3A and B), and no adult parasites in the intestine, confirms the diagnosis of rhabdiasid lungworm eggs and larvae, compatible with the genus Serpentirhabdias.
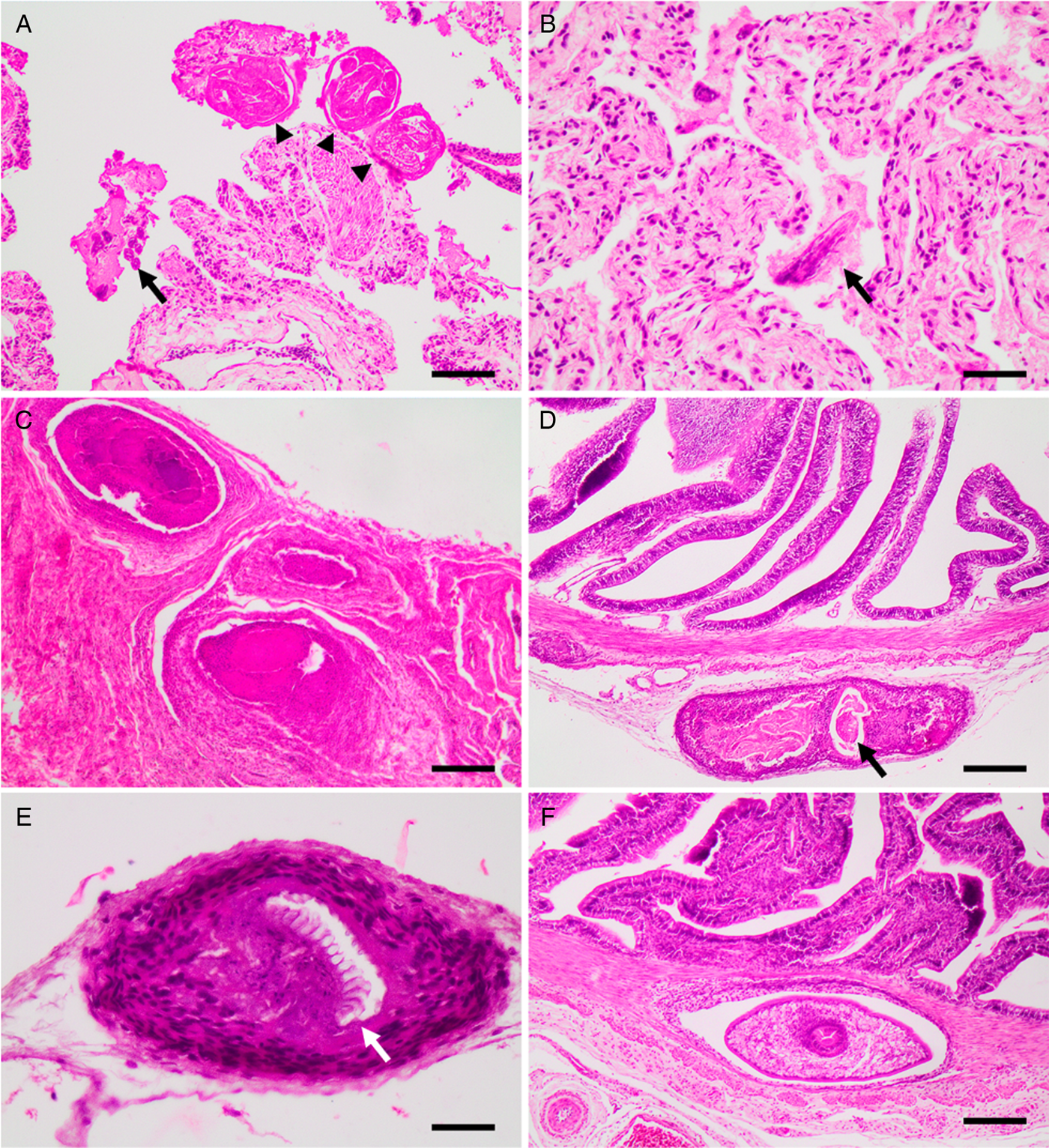
Fig. 3. Histological sections showing, (A) lung with adults (arrow heads) and larvae (arrow) of Serpentirhabdias sp. H&E. Scale bar = 100 μm. (B) Lung with atelectasis and mild suppurative inflammation. Note the longitudinal section of the head of a Serpentirhabdias larva (arrow). H&E. Scale bar = 40 μm. (C) Lung granulomas. H&E. Scale bar = 200 μm. (D): A chronic-active granuloma, with intralesional dead nematode, on the intestinal serosa (arrow). H&E. Scale bar = 200 μm. (E) A chronic granuloma, containing a dead tapeworm surrounded by a wall of fibrocytes. Note the preserved rostellum (arrow). H&E. Scale bar = 40 μm. (F) A cystic granuloma in the muscular layer of the intestine containing a cysticercoid. H&E. Scale bar = 200 μm.
Adult parasites
Just two specimens out of the 108 snakes showed adult parasites, one specimen from each group (A and B). From group A, the snake passing parasite eggs in its feces was found infested with Serpentirhabdias lungworms. From group B, one snake harboured 19 Mesostigmatid skin mites of the family Macronyssidae, in various life stages, compatible with Ophionyssus natricis (Fig. 4F).

Fig. 4. (A) Rostellum in apical view, note the five rows of hooks. Scale bar = 50 μm of Diplopylidium acanthotetra. (B) Morphological type No. 1 of larval spirurids, possible Spirocercidae. Inset: posterior end. Note the two papillae on the anterior end, and the tuft of digitations on the tail. Scale bar (left) = 50 μm, scale bar (right) = 10 μm. (C) Cysticercoid of Diplopylidium acanthotetra. Scale bar = 200 μm. (D) Morphological type No. 2 of larval spirurids, possible Acuarinae. Note the two papillae and the straight reticular cordon. Scale bar = 50 μm. (E) Morphological type No. 3 of larval spirurids, possible Physalopteroidea. Scale bar = 50 μm. (F) Cleared specimen of Ophionyssus naticis. Scale bar = 200 μm.
Identification of the larval helminths
The metacestodes (Figs 2C, 4A and C) found were characterized as a solid larval form, with 4 suckers and 4–5 rows of taenioid and rose thorn hooks which identifies them as two species of the genus Diplopylidium, D. acanthotetra (70.3% prevalence) and D. nölleri (21.9% prevalence). Several specimens of both species were deposited at the Parasites and Vectors collection of the Natural History Museum of London (accession numbers: NHMUK.2020.2.12.1-2 and NHMUK.2020.2.12.3).
Nematodes were characterized based on the divided oesophagus (muscular and glandular) and the three morphological types found correspond to the order Spirurida and, based on other morphological features, possibly to three superfamilies: Spiruroidea (Type 1), Acuarioidea (Type 2) and Physalopteroidea (Type 3).
The first and most common helminth (Fig. 4B) (45.3% prevalence) was characterized by two prominent papillae at the anterior end and thin lateral wings that begin posterior to the nerve ring, up to a few micrometres before the cloaca. These also possess a tuft of finger-shaped papillae at the posterior end. This description matches several larvae from genera of the same taxa: superfamily Spiruroidea, family Spirocercidae.
In the second type (Fig. 4D) (35.9% prevalence), there were two pseudo-labia at the anterior end, accompanied by four non-recurrent or anastomosed reticular cordons and two monocuspic cervical papillae. At the posterior end, no papillae or other ornaments were found. These characteristics match those described for larvae from the superfamily Acuarioidea, subfamily Acuarinae, which are the only ones in which larvae have cephalic cordons. Finally, the third (Fig. 4E) and smallest morphological type (4.7% prevalence) was characterized by two trilobed lips, a posterior end without papillae and thin lateral alae. These larvae likely belong to the superfamily Physalopteroidea.
Gross and microscopic findings
One snake presented with diffuse red discolouration of the lung with numerous, multifocal to coalescing white, round nodules, 0.5–2 mm diameter, affecting around 70% of the cranial lung parenchyma (Fig. 2B).
Microscopically, these lesions corresponded to multifocal mild verminous pneumonia composed of a mild infiltration of heterophils, macrophages and lymphocytes, as well as a small amount of cellular debris, fibrin and oedema surrounding adult and larval nematodes inside faveolar spaces. Mild pulmonary congestion as well as faveolar atelectasis were also observed (Figs 3B and A). In addition, occasional pleural granulomas with intralesional bacteria were observed (Fig. 3C).
The parasites were characterized by the presence of a body cavity and an external eosinophilic cuticle, surrounding an inner layer of degenerated platymyarian muscle fibres, a digestive tract and a reproductive structure compatible with ovary (Fig. 3A). Also present, predominantly within faveolar walls and the interstitium, were often highly cellular and basophilic larval forms. On sagittal sections, a single-cell digestive tract was observed – compatible with early larval stages (L1–L2).
In total, 98.4% of snakes presented with moderate to severe multifocal white nodular lesions from 0.7 to 5 mm in diameter on the serosal surface of the intestine and stomach, and coelomic wall (Fig. 2D). Microscopically, these lesions were present in various stages of maturity (Figs 3D and E) and were located mostly in the serosal and muscular layer of the intestine (Fig. 3F) and coelomic wall (Fig. 3E). The lesions were microscopically characterized as cystic granulomas, composed of an outer layer of fibroblasts, fibrocytes and collagen surrounded by several layers of compacted macrophages and few lymphocytes and heterophils, surrounding a parasitic structure that floated in an unstained fluid. These parasites (Cestoda) were characterized by the presence of an outer thick eosinophilic tegumentary layer, lack of body cavity, and in its place a vacuolated space without digestive system, presence of armed scolex and suckers, lack of reproductive organs and presence of calcareous corpuscles.
Non-cystic granulomas were also found containing cysticercoids, with a well-preserved rostellum, surrounded by numerous macrophages, lymphocytes, fibroblasts and fibrocytes, or with more active inflammation including the presence of heterophils inside the granulomas (Figs 3D and E). Associated with these latter areas of inflammation were degenerated nematode larvae (Fig. 3D).
Discussion
Here the first record of two Diplopylidium species and three larval nematode morphological types in the California kingsnake are described. These findings are consistent with local parasites infecting an invasive species, which would act as a ‘dead-end’ paratenic host, since no predators are described for this snake in Gran Canaria.
Larval helminth cysts from reptiles, and snakes specifically, have been reported previously in the literature; however, just Mesocestoides tetrathyridia and Macracanthorhynchus cystacanths, have been described in a Lampropeltis sp. snake (Elkins and Nickol, Reference Elkins and Nickol1983; Jacobson, Reference Jacobson and Jacobson2007).
Only larvae from members of the superfamilies Spiruroidea, Acuarioidea and Physalopteroidea have been commonly described parasitizing reptiles (Anderson, Reference Anderson2000; Criscione and Font, Reference Criscione and Font2001; Goldberg and Bursey, Reference Goldberg and Bursey2001; Santos et al., Reference Santos, Martínez-Freiría, Pleguezuelos and Roca2006). Specifically; Physocephalus, Ascarops and Spirocerca lupi, from Spirocercidae (Moravec et al., Reference Moravec, Baruš and Ryšavý1987; Goldberg and Bursey, Reference Goldberg and Bursey1988; Goldberg et al., Reference Goldberg, Bursey and Holshuh1994), Acuarinae gen. from Acuarioidea (Roca, Reference Roca1985; Moravec et al., Reference Moravec, Baruš and Ryšavý1987) and Physaloptera, from Physalopteroidea (Widmer, Reference Widmer1970).
Regardless of the parasitic species, the burden and prevalence found in the Gran Canaria snake population (98.4%) is considerably higher than that reported for larval parasites from other reptiles in Spain, and indeed North America (usually less than 2%) (Widmer, Reference Widmer1967; Roca, Reference Roca1985; Martín et al., Reference Martín, Roca, Galdón, Sánchez-Mut and Muniesa2003; Santos et al., Reference Santos, Martínez-Freiría, Pleguezuelos and Roca2006; Goldberg et al., Reference Goldberg, Bursey and Glaudas2013; Davis et al., Reference Davis, Beane and Flowers2016). Furthermore, there are no records of Diplopylidium species in North American fauna (Criscione and Font, Reference Criscione and Font2001; Goldberg and Bursey, Reference Goldberg and Bursey2001; Yildirimhan et al., Reference Yildirimhan, Bursey and Goldberg2005; Goldberg et al., Reference Goldberg, Bursey and Glaudas2013; Davis et al., Reference Davis, Beane and Flowers2016; McAllister and Bursey, Reference McAllister and Bursey2016). This difference could be due to the use of macroscopic examination during necropsy, instead of using compression plates (trichinoscopy) to see through the organ. In fact, the data obtained using the two techniques in this study differ by almost 30%, with the more sensitive utilization of trichinoscopy being developed through research on larval parasitic forms of Trichinella.
Due to the lack of studies in the California kingsnake, it is not possible to compare the epidemiological results here with the native American populations of these snakes. In the Canary Islands, a maximum prevalence of 21.7% for D. nölleri and 2.2% for D. acanthotetra (Roca et al., Reference Roca, Martin and Carbonell1999) is reported in the endemic gecko species described as natural hosts for the tapeworm species. Meanwhile in its invasive host the general prevalence was 25.8 and 76.7%, respectively. Moreover, the mean intensity registered for Diplopylidium species in endemic geckoes was also lower, reaching a maximum of 37.5 cysticercoids for D. nölleri from the Boettger's wall gecko (T. boettgeri) in Gran Canaria, vs 43.8 larvae in the snakes. In addition, the anatomic distribution of the cestodes was similar in the two hosts; in both species on the intestinal serosa, but in the case of geckoes, they were also found on the liver surface (Roca et al., Reference Roca, García-Adell, López and Zapatero-Ramos1987). These findings could mean that Diplopylidium cysticercoids are being transferred from geckoes to the snakes. The only suitable definitive carnivore hosts on the island are cats and dogs, with only Diplopylidium acanthotetra described in cats from the island of Tenerife (Sánchez, Reference Sánchez2013).
There is only one report of larval nematodes, which match the morphology described in this study as ‘Type 1’, parasitizing the endemic Boettger's lizard (Gallotia caesaris) on the island of El Hierro (Martín et al., Reference Martín, Roca, Galdón, Sánchez-Mut and Muniesa2003). In that study, they reported a mean intensity of 2 larvae and a prevalence of 2.5%, which is significantly lower in comparison with the mean intensity of 186 larvae and 90% prevalence found in these kingsnakes. Apart from the report from G. caesaris from El Hierro, no other larval nematodes have been recorded in vertebrate hosts which can be eaten by the snakes in Gran Canaria, thus the previous paratenic host remains unknown.
Furthermore, it has been demonstrated that geckoes can harbour larval spirurids of the three morphological types described in this study (Criscione and Font, Reference Criscione and Font2001; McAllister and Bursey, Reference McAllister and Bursey2016), therefore despite the negative results found in Gran Canaria populations, Boettger's wall gecko would be a suitable previous paratenic host for these nematodes.
Ophionyssus natricis is a widely distributed mite that represents a potential zoonotic risk, predominantly in suburban areas, where the wildlife–human interface shrinks. There are no reported cases of human infestation from Gran Canaria, however, with concentrated sampling effort of habitations within the larger nuclei, its presence may be demonstrated. In addition to dermatitis in humans (Schultz, Reference Schultz1975), other reptiles can be infested with this mite (Norval et al., Reference Norval, Halliday, Sih, Sharrad and Gardner2020) which can also act as a vector for Aeromonas sp. (Wozniak and DeNardo, Reference Wozniak and DeNardo2000), leading to fatal disease in certain cases. Hence, the possible spillover of these mites, as well as Serpetirhabdias sp., to local fauna, requires further research.
In addition to the ecosystem damage caused by predation of native fauna and competition with other native predators for food, these snakes can be considered ‘dead-end’ paratenic hosts. Most of the parasites found here will not be able to reach their respective definitive hosts, such as birds of prey, and thus are disrupting natural life cycles in the island. For example, once the population of geckoes decreases, the transmission of parasites, such as acuariid nematodes, will likely be affected, since these are transmitted to birds of prey by eating geckoes (as paratenic hosts) rather than consuming terrestrial isopods or other arthropods (intermediate hosts). This is a rather less obvious, but no less important, consequence of the presence of invasive snakes which will be contributing not only to the extinction of geckoes and other native reptiles, but also to their respective parasites of which several are endemic to the Canary Islands, e.g. Thelandros filiformis, Alaeuris stehlini, Sarcocystis stehlini, Ophionyssus setosus.
There was a positive correlation between nematode burden and the age of the snake, a correlation that did not exist for Diplopylidium sp. When an animal is exposed to a new parasite, the immune response is usually more severe than would occur in response to a parasite with which it had had co-evolved (Kelehear and Jones, Reference Kelehear and Jones2010). No records of Diplopylidium have been found for North American fauna, unlike larval spirurids, therefore in its natural habitat, the California kingsnake would not be exposed to Diplopylidium. An increased immune response to new parasites could mean that the California kingsnake more efficiently controls tapeworms, rather than accumulating them during life as with nematodes, which would explain the positive correlation between longevity and parasite burden. Moreover, this could also explain how, if the two parasitic groups have the same donor host, such as the Boettger's wall gecko, that a correlation could exist for just one.
Rhabdiasid lungworms are considered common parasites of amphibians and reptiles (Anderson, Reference Anderson2000); however, their prevalence in North American snakes is very low. Only single cases of rhabdiasid-induced pneumonias, with limited gross and histological descriptions, are reported in snakes from various countries (Jacobson, Reference Jacobson and Jacobson2007; Langford, Reference Langford2010; Mihalca et al., Reference Mihalca, Micluş and Lefkaditis2010; Goldberg et al., Reference Goldberg, Bursey and Glaudas2013; Davis et al., Reference Davis, Beane and Flowers2016).
Of the eight genera of Rhabdiasid nematodes which can infest reptiles, only one has been described in Lampropeltis spp. snakes, with two species: Serpentirhabdias fuscovenosa and S. eustreptos (Langford, Reference Langford2010).
The low prevalence of Serpentirhabdias in kingsnakes in Gran Canaria could be explained by the relatively infrequent exposure of the snakes to their own feces in the environment, and their captive-bred origin. These first feral, presumed dewormed, animals had been released into an environment lacking existing snake parasites or natural paratenic hosts.
Parasitic pneumonias in snakes are reported with variable severity, from suppurative pneumonias with clear clinical signs and death (Jacobson, Reference Jacobson and Jacobson2007), to those with only mild microscopic changes (Santos et al., Reference Santos, Takahira, Rall, Calderón, Sequeira and Silva2008).
Infective larvae of Serpentirhabdias sp. can inoculate soil bacteria, carried inside their intestines, within the snake lung, resulting in a significant secondary bacterial pneumonia with prominent gross lesions (Santos et al., Reference Santos, Takahira, Rall, Calderón, Sequeira and Silva2008). Various Gram-negative bacteria such as Proteus sp. and Pseudomonas sp. (Hilf et al., Reference Hilf, Wagner and Yu1990; Santos et al., Reference Santos, Takahira, Rall, Calderón, Sequeira and Silva2008) have been isolated from parasitized animals, likely acting as opportunistic agents, rather than the primary pathogen. Thus, the lung granulomas found in this study are possibly caused by inoculated soil bacteria, through indirect parasite damage.
Physaloptera sp. nematodes are described producing gastric lesions via larval penetration in a prairie rattlesnake (Crotalus viridis) in the USA (Widmer, Reference Widmer1970). In contrast, in this study, encysted larvae are described, with the small and large intestine representing a new anatomic location for these parasites. In addition, similar lesions to this report have been described for larval spirurids in several reptiles and amphibians (Goldberg and Bursey, Reference Goldberg and Bursey1988; McAllister et al., Reference McAllister, Goldberg and Holshuh1993; Goldberg et al., Reference Goldberg, Bursey and Holshuh1994).
Acknowledgements
The authors would like to thank the collaboration of Ramón Gallo Barneto, Head of Gestión y planeamiento territorial y ambiental (GesPlan S.A.) as well as Miguel Ángel Cabrera Pérez, from Servicio de Biodiversidad, Dirección general de protección de la naturaleza, Gobierno de Canarias, for the donation of the specimens. We would also like to thank the personnel of GesPlan, who collected snakes in the field. Finally, we would like to thank de Blas and Raul Bruno for their help with photography and graphic content.
Financial support
This study was supported by the project ‘POSTLIFE + Lampropeltis para el control de la culebra real de California en Gran Canaria (LIFE10/NAT/ES/656)’ financed by the Government of Canary Islands and Cabildo of Gran Canaria.
Conflict of interest
None.
Ethical standards
Not applicable.