Introduction
Haemogregarines (Apicomplexa: Adeleiorina) are heteroxenous, intraerythrocytic or intraleucocytic parasites, infecting a broad range of vertebrate intermediate hosts including amphibians, reptiles, fishes, birds and mammals. These parasites are possibly transmitted by an equal diversity of haematophagous invertebrate definitive hosts or vectors, such as dipteran insects, ticks, mites, leeches and even gnathiid isopods (see Smith, Reference Smith1996; Davies and Johnston, Reference Davies and Johnston2000; Curtis et al. Reference Curtis, Grutter, Smit and Davies2013). Haemogregarines are currently divided into four families (Barta et al. Reference Barta, Ogedengbe, Martin and Smith2012), namely Dactylosomatidae Jakowska and Nigrelli, 1955, Haemogregarinidae Léger, 1911, Hepatozoidae Miller, 1908, and Karyolysidae Labbé, 1894.
Within the Hepatozoidae, Hepatozoon Miller, 1908 is characterized by the presence of gamonts in erythrocytes or leucocytes, with no merogonic division occurring in the peripheral blood of the vertebrate host. Furthermore, Hepatozoon species are characterized by the pairing (syzygy) of gamonts in the definitive invertebrate host or vector following a blood meal. These paired gamonts then penetrate the gut wall and enter the haemocoel where sporogonic development and ultimately the formation of large oocysts occur. These thick-walled oocysts (also known as large multisporocystic oocysts) contain sporocysts with sporozoites, the infective stages of the parasite, which emerge upon the ingestion by the intermediate vertebrate host and give rise to merogonic stages in the liver (Desser et al. Reference Desser, Hong and Martin1995; Smith, Reference Smith1996; Barta, Reference Barta, Lee, Leedale and Bradbury2000).
Hepatozoon species are the most commonly reported haemogregarines to parasitize anurans. Currently, there are 45 recognized species from anurans globally, with 16 of these described from African hosts (see Smith, Reference Smith1996; Netherlands et al. Reference Netherlands, Cook and Smit2014a, Reference Netherlands, Cook, Smit and Du Preezb). According to Netherlands et al. (Reference Netherlands, Cook and Smit2014a), the majority of these species (12/16) were described from the Bufonidae, namely Hepatozoon aegyptia (Mohammed and Mansour, 1963), Hepatozoon assiuticus (Abdel-Rahman, El-Naffar, Sakla and Khalifa, 1978), Hepatozoon boueti (França, 1925), Hepatozoon faiyumensis (Mansour and Mohammed, 1966), Hepatozoon francai (Abdel-Rahman, El-Naffar, Sakla and Khalifa, 1978), Hepatozoon froilanoi (França, 1925), Hepatozoon ixoxo Netherlands, Cook and Smit, 2014, Hepatozoon lavieri (Tuzet and Grjebine, 1957), Hepatozoon magni (Hassan, Reference Hassan1992), Hepatozoon moloensis (Hoare, Reference Hoare1920), Hepatozoon pestanae (França, 1910), and Hepatozoon tunisiensis (Nicolle, 1904). Two species were described from the Ptychadenidae, namely Hepatozoon epuluensis (Van den Berghe, Reference Van den Berghe1942), and Hepatozoon neireti (Laveran, Reference Laveran1905), and only a single species from the Pyxicephalidae and Hyperoliidae, namely Hepatozoon theileri (Laveran, Reference Laveran1905), and Hepatozoon hyperolli (Hoare, Reference Hoare1932), respectively. Apart from Hepatozoon hyperolli, which was described from an unidentified Hyperolius species in Uganda (Hoare, Reference Hoare1932), the only other Hepatozoon species reported from the Hyperoliidae are two unnamed species reported in Hyperolius marmoratus and Hyperolius puncticulatus, from northern KwaZulu-Natal (KZN), South Africa (Netherlands et al. Reference Netherlands, Cook, du Kruger, Du Preez and Smit2015) and Amani, Tanzania (Ball, Reference Ball1967), respectively. In South Africa, only two Hepatozoon species are known from anurans, namely H. theileri and H. ixoxo, from the pyxicephalid Amietia delalandii (syn. Amietia quecketti) and three Sclerophrys species (Bufonidae) respectively, namely Sclerophrys pusilla (syn. Amietophrynus maculatus), Sclerophrys (syn. Amietophrynus) garmani and Sclerophrys (syn. Amietophrynus) gutturalis.
Over the past decade several phylogenetic studies on adeleorinid parasites, using 18S rDNA sequences, have provided useful insight into the evolutionary relationships of this group, as well as the better capability to distinguish between species. However, because the 18S rRNA nuclear gene is a relatively conserved marker, it shows certain nodes to be unresolved (Barta et al. Reference Barta, Ogedengbe, Martin and Smith2012; Maia et al. Reference Maia, Perera and Harris2012; Haklová-Kočíková et al. Reference Haklová-Kočíková, Hižňanová, Majláth, Račka, Harris, Földvári, Tryjanowski, Kokošová, Malčeková and Majláthová2014; Cook et al. Reference Cook, Netherlands and Smit2016). In an effort to resolve these polytomies, a new genus Bartazoon Karadjian, Chavatte and Landau 2015, was proposed for species previously regarded as belonging to Hepatozoon parasitizing reptiles, amphibians, marsupials, birds and rodents, and was proposed to be transmitted solely by biting insects (Karadjian et al. Reference Karadjian, Chavatte and Landau2015). However, the suggested life history of certain species within the proposed genus such as Hepatozoon fitzsimonsi (Dias, 1953) do not conform to the recommended characteristic defining Bartazoon (see Cook et al. Reference Cook, Lawton, Davies and Smit2014; Karadjian et al. Reference Karadjian, Chavatte and Landau2015). Also as pointed out by Maia et al. (Reference Maia, Harris, Carranza and Gomez-Diaz2016Reference Maia, Carranza and Harrisb), it is possible that Hepatozoon perniciosum Miller, 1908, the type species of the genus Hepatozoon, may, in fact, form part of the newly proposed genus Bartazoon, as most other rodent haemogregarine species do. Furthermore, increased work on the phylogenetic relationships of the haemogregarines continues to identify new genetic lineages, showing that Bartazoon is not a well-supported monophyletic group (Tomé et al. Reference Tomé, Rato, Harris and Perera2016; Maia et al. Reference Maia, Harris, Carranza and Gomez-Diaz2016a). Thus, to revise the deeper taxonomy (family and genus level) of haemogregarines based on their phylogenetic affinities and life histories, more studies using faster-evolving markers such as mitochondrial genes (e.g. Leveille et al. Reference Leveille, Ogedengbe, Hafeez, Tu and Barta2014), elucidating life cycles, and building larger datasets are necessary. Therefore, as suggested and used by Maia et al. (Reference Maia, Carranza and Harris2016b) we will continue to refer to species parasitizing anuran hosts as species of Hepatozoon and not Bartazoon.
Prior to the study of Netherlands et al. (Reference Netherlands, Cook and Smit2014a) all the African anuran Hepatozoon species descriptions, ranging from the early 1900s till the late 1970s, were solely based on the morphology of the peripheral blood gamont stages. Unfortunately, many of these descriptions were scantily illustrated and incomplete, with almost 60% of the species described from the same host (Sclerophrys regularis) and in more or less the same geographical area (see Netherlands et al. Reference Netherlands, Cook and Smit2014a, Reference Netherlands, Cook, Smit and Du Preezb). Thus many of these species may later need to be synonymized once more advanced and standardized methods are used to characterize these haemogregarines. In South Africa only five studies on amphibian haemogregarines have been carried out (Laveran, Reference Laveran1905; Fantham et al. Reference Fantham, Porter and Richardson1942; Netherlands et al. Reference Netherlands, Cook and Smit2014a, Reference Netherlands, Cook, Smit and Du Preezb; Netherlands et al. Reference Netherlands, Cook, du Kruger, Du Preez and Smit2015). From these, only a single study was a multispecies haemoparasite survey across different anuran families (Netherlands et al. Reference Netherlands, Cook, du Kruger, Du Preez and Smit2015), and although in that study several different haemogregarines were observed in anurans, only one hyperoliid species, Hyp. marmoratus (as mentioned above) contained a Hepatozoon species, which was not identified to species level.
Thus the objectives of the current study were (1) to establish which hyperoliid frog species in northern KZN, South Africa, contain haemogregarines. (2) to determine the species diversity of the haemogregarine parasites observed. (3) to ascertain if any of the haemogregarines found were previously described or reported species and (4) to compare any parasites characterized in the current study with available molecular data for anuran haemogregarines in order to determine their phylogenetic relationships.
Materials and methods
Frog collection and study area
A total of 225 individuals representing nine hyperoliid species, were collected from several localities throughout northern KwaZulu-Natal, South Africa (Fig. 1), following the collection methods described in Netherlands et al. (Reference Netherlands, Cook, du Kruger, Du Preez and Smit2015). Frogs were identified using Du Preez and Carruthers (Reference Du Preez and Carruthers2009), and identifications were confirmed by one of the authors of this guide (LdP). After processing, all specimens were released at the site of capture. This study received the relevant ethical approval from the North-West University's AnimCare ethics committee (ethics number: NWU-00372-16-A5).

Fig. 1. Map of the sampling localities in northern KwaZulu-Natal, South Africa. Ndumo Game Reserve (NGR) 26°52′00″S, 32°15′00″E, the area directly surrounding the NGR (SNGR) 27°00′13″S, 32°16′50″E, Kwa Nyamazane Conservancy (KNC) 27°23′35″S, 32°08′41″E, Bonamanzi Game Reserve (BGR) 28°03′25″S 32°17′42″E, Kosi Bay (KB) 26°57′16″S 32°48′07″E, KwaMbonambi/Langepan (KB/LP) 28°39′43″S 32°10′06″E, St. Lucia (SL) 28°23′10″S 32°24′29″E and St. Lucia Monzi Farm (SLMF) 28°26′56″S 32°17′18″E.
Processing of samples and light microscopy screening
Blood (>0·1 mL) was taken from each frog via cardiac or femoral venipuncture and thin blood smears prepared on clean glass slides, air-dried, fixed and stained using Giemsa-stain (FLUKA, Sigma-Aldrich, Steinheim, Germany). The remaining blood was preserved in 70% ethanol for molecular work (ratio 1 : 15). Stained blood smears were screened at 1000× and images captured and measured using the imaging software NIS Elements Ver. 4 as described by Netherlands et al. (Reference Netherlands, Cook, du Kruger, Du Preez and Smit2015). Fifty mature gamonts were measured per Hepatozoon species. Measurements comprised the parasite's length (including recurved tail when present) and width within its parasitophorous vacuole (PV), and the parasite's nucleus length and width. Measurements of the PV length and width, and the length from mid nucleus to both anterior and posterior end of the parasite were also taken. Parasitaemia was calculated per 100 erythrocytes, with ~104 erythrocytes examined per blood smear, following previous methods (see Cook et al. Reference Cook, Sikkel, Renoux and Smit2015a).
DNA extraction, PCR amplification and phylogenetic analyses
Ethanol-preserved blood samples from parasitized frog specimens (n = 10) were used for molecular work. Two additional blood samples of A. delalandii parasitized with H. theileri and S. pusilla parasitized with H. ixoxo from a previous study (Netherlands et al. Reference Netherlands, Cook and Smit2014a) were added to obtain longer comparative sequences as compared with the previous study by Netherlands et al. (Reference Netherlands, Cook and Smit2014a). Genomic DNA of haemogregarine species were extracted from the blood samples using the KAPA Express Extract Kit (Kapa Biosystems, Cape Town, South Africa). Once extracted, DNA was used for polymerase chain reaction (PCR) amplification. The PCR reactions targeted two fragments of approximately 940 and 1400 nt of the 18S rRNA gene. The 18S rRNA gene sequences were amplified using a combination of two primer sets based on previous studies of haemogregarines belonging to Karyolysus Labbé, 1894, Hemolivia Petit, Landau, Baccam and Lainson, 1990 and Hepatozoon (see Ujvari et al. Reference Ujvari, Madsen and Olsson2004; Criado-Fornelio et al. Reference Criado-Fornelio, Ruas, Casado, Farias, Soares, Müller, Brum, Berne, Buling-Saraña and Barba-Carretero2006; Cook et al. Reference Cook, Netherlands and Smit2015b, Reference Cook, Netherlands and Smit2016). The first fragment was amplified using HAM-F (5′-GCCAGTAGTCATATGCTTGTC-3′) and HepR900 (5′-CAAATCTAAGAATTTCACCTCTGAC-3′) (see Ujvari et al. Reference Ujvari, Madsen and Olsson2004; Criado-Fornelio et al. Reference Criado-Fornelio, Ruas, Casado, Farias, Soares, Müller, Brum, Berne, Buling-Saraña and Barba-Carretero2006), and the second fragment HepF300 (5′-GTTTCTGACCTATCAGCTTTCGACG-3′) and 2868 (5′-TGATCCTTCTGCAGGTTCACCTAC-3′) (see Medlin et al. Reference Medlin, Elwood, Stickel and Sogin1988; Ujvari et al. Reference Ujvari, Madsen and Olsson2004). Conditions for PCR were as follows: initial denaturation at 95 °C for 3 min, followed by 35 cycles, entailing a 95 °C denaturation for 30 s, annealing at 61 °C for 30 s with an end extension at 72 °C for 2 min, and following the cycles a final extension of 72 °C for 10 min. PCR reactions were performed with volumes of 25 µL, using 12·5 µL Thermo Scientific DreamTaq PCR master mix (2×) (final concentration: 2× DreamTaq buffer, 0·4 mm of each dNTP, and 4 mm MgCl2), 1·25 µL (10 µ m) of each of the primer sets mentioned above, and at least 25 ng DNA. The final reaction volume was made up with PCR-grade nuclease-free water (Thermo Scientific). Reactions were undertaken in a Bio-Rad C1000 Touch™ Thermal Cycler PCR machine (Bio-Rad, Hemel Hempstead, UK). Resulting amplicons were visualized under ultraviolet light on a 1% agarose gel stained with gel red using a Bio-Rad GelDoc™ XR+ imaging system (Bio-Rad, Hemel Hempstead, UK). PCR products from each sample were sent to a commercial sequencing company (Inqaba Biotechnical Industries (Pty) Ltd, Pretoria, South Africa) for purification and sequencing in both directions. Resultant sequences were assembled, and chromatogram-based contigs were generated and trimmed using Geneious R9·1 (http://www.geneious.com, Kearse et al. Reference Kearse, Moir, Wilson, Stones-Havas, Cheung, Sturrock, Buxton, Cooper, Markowitz, Duran, Thierer, Ashton, Mentjies and Drummond2012). Sequence and species identity was verified against previously published sequences using the Basic Local Alignment Search Tool (BLAST) (Altschul et al. Reference Altschul, Gish, Miller, Myers and Lipman1990). Sequences obtained in the current study were deposited in the NCBI GenBank database under the following accession numbers [GenBank: MG041591–MG041605].
For comparison, all 18S rDNA sequences of anuran haemogregarines, longer than 1500 nt (comprising species of Hepatozoon, Hemolivia, Babesiosoma and Dactylosoma) as well as Hepatozoon sipedon Smith, Desser and Martin, 1994, [GenBank: JN181157] from the snake Nerodia sipedon sipedon, were downloaded from GenBank and aligned to the sequences generated in the current study. Hepatozoon sipedon was selected as it was shown by Barta et al. (Reference Barta, Ogedengbe, Martin and Smith2012) to be sister to Hepatozoon catesbianae (Stebbins, 1904) and Hepatozoon clamatae (Stebbins, 1905), at that point the only two species of Hepatozoon of frogs for which 18S rDNA sequences were available. Furthermore, H. sipedon first makes use of a frog intermediate host in which tissue development occurs before transmission to its second intermediate snake host (see Smith et al. Reference Smith, Desser and Martin1994). Thus all species included in the analysis have an anuran host in their life cycle. Although there are other sequences available from a Hepatozoon species characterized from the anurans Pelophylax perezi [GenBank: KF733812] and Leptodactylus chaquensis [GenBank: JX987775], from the Azores in the North Atlantic Ocean, and Pantanal, Brazil respectively, they were not added to our analysis because these concerned shorter fragments (see Harris et al. Reference Harris, Spigonardi, Maia and Cunha2013; Leal et al. Reference Leal, Dreyer, da Silva, Ribolla, dos Santos Paduan, Bianchi and O'Dwyer2015). Babesiosoma stableri Schmittner and McGhee, 1961 [GenBank: HQ224961] and Dactylosoma ranarum Lankester, 1871 [GenBank: HQ224957; HQ224958] were chosen as the outgroup, as they were shown by Barta et al. (Reference Barta, Ogedengbe, Martin and Smith2012) to belong to a sister group to our current ingroup. Sequences were aligned using the MUSCLE alignment tool (Edgar, Reference Edgar2004) under the default settings and implemented in Geneious R9·1. The alignment consisted of 14 sequences with a 1497 nt conserved region selected using the Gblocks 0·91b server (Castresana, Reference Castresana2000). To infer phylogenetic relationships both Bayesian inference (BI) and Maximum likelihood (ML) methods were used. The BI analysis was performed using MrBayes 3·2·2 (Huelsenbeck and Ronquist, Reference Huelsenbeck and Ronquist2001) and the ML analysis was performed using RAxML Ver. 7·2·8. (Stamatakis, Reference Stamatakis2014) both implemented from within Geneious R9·1. Prior to the analyses, a model test was performed to determine the most suitable nucleotide substitution model, according to the Akaike information criterion using jModelTest 2·1·7 (Guindon and Gascuel, Reference Guindon and Gascuel2003; Darriba et al. Reference Darriba, Taboada, Doallo and Posada2012). The model with the best AICc score was the Transitional model (Posada, Reference Posada2003) with estimates of invariable sites and a discrete Gamma distribution (TVM + I + Γ). However, this model was substituted by the General Time Reversible (Tavaré, Reference Tavaré1986) model (GTR + I + Γ) in MrBayes and in RAxML, as this was the next model available with the best AICc score. For the BI analysis, the Markov Chain Monte Carlo (MCMC) algorithm was run for 10 million generations, sampling every 100 generations, and using the default parameters. The first 25% of the trees were discarded as ‘burn-in’ with no ‘burn-in’ samples being retained. Results were visualized in Trace (implemented from within Geneious R9·1), to assess convergence and the burn-in period. For the ML analysis, nodal support was assessed using 1000 rapid bootstrap inferences. Model-corrected (TVM + I + Γ) genetic distances were calculated in PAUP version 4.0a152 (Swofford, Reference Swofford2002), with the assumed proportion of invariable sites = 0·598 and the gamma shape parameter = 0·775.
Results
A total of 225 individuals representing nine species from the family Hyperoliidae, namely Afrixalus aureus (n = 18), Afrixalus delicatus (n = 13), Afrixalus fornasinii (n = 14), Hyperolius argus (n = 39), Hyperolius marmoratus (n = 74), Hyperolius tuberlinguis (n = 38), Hyperolius pusillus (n = 14), Kassina senegalensis (n = 9), and Phylctimantis (syn. Kassina) maculatus (n = 6) were collected and screened for haemogregarines. Twenty frogs (8·9%) from three species were found positive for haemogregarines, specifically A. fornasinii (6/14), Hyp. argus (2/39), and Hyp. marmoratus (12/74) (see Fig. 2A–C). Based on peripheral blood stages, the haemogregarines of the current study conform to the genus Hepatozoon. Although possible meront stages were observed in the peripheral blood for one species, these were rare and no merogonic division was detected. Furthermore, these haemogregarines did not compare with the closely related genus Hemolivia, as no schizogony or cyst formation in the erythrocytes of the hosts was observed.

Fig. 2. Three frog species found positive for haemogregarines. (A) Afrixalus fornasinii, (B) Hyperolius argus, and (C) Hyperolius marmoratus.
Species descriptions
Phylum: Apicomplexa Levine, 1970
Class: Conoidasida Levine, 1988
Order: Eucoccidiorida Léger & Duboscq, 1910
Suborder: Adeleorina Léger, 1911
Family: Hepatozoidae Wenyon, 1926
Genus: Hepatozoon Miller, 1908
Hepatozoon involucrum Netherlands, Cook and Smit n. sp.
Type-host: Hyperolius marmoratus Rapp, 1842 (Anura: Hyperoliidae).
Vector: Unknown.
Type-locality: The specimens were collected in the Kwa Nyamazane Conservancy (KNC), KwaZulu-Natal, South Africa (27°23′35″S, 32°08′41″E).
Other localities: St. Lucia on Monzi Farm, KwaZulu-Natal, South Africa (28°26′56″S 32°17′18″E).
Type-material: Hapantotype, 1× blood smear from Hyp. marmoratus deposited in the protozoan collection of the National Museum, Bloemfontein, South Africa under accession number NMB P 467; parahapantotype, 1× blood smear from Hyp. marmoratus; deposited in the Protozoan Collection of the National Museum, Bloemfontein (NMB), South Africa, under accession number NMB P 468.
Representative DNA sequences: The 18S rRNA gene sequences have been submitted to the GenBank database under the accession numbers MG041591–MG041594.
ZooBank registration: The Life Science Identifier (LSID) of the article is urn:lsid:zoobank.org:pub:F73407D7-1E08-4C3C-B066-889058B77C4C. The LSID for the new name Hepatozoon involucrum Netherlands, Cook and Smit is urn:lsid:zoobank.org:act:A43D46E8-5C9F-4405-8907-94D7B02EAEA7.
Etymology: The species epithet is derived from the Latin word involucrum meaning envelope or sheath, and is based on the prominent PV encircling the gamont.
Description:
Trophozoites: rare, occurring singularly within erythrocytes, oval to rounded, measuring 12·2–12·5 (12·3 ± 0·2) μm long × 4·8–5·7 (4·2 ± 0·6) μm wide (n = 2) with finely vacuolated cytoplasm staining whitish-pink (Fig. 3A and B), note lysis of the host cell nucleus (Fig. 3B). Nucleus containing loosely arranged chromatin, staining pink, measuring 3·7–5·2 (4·5 ± 1·0) μm long × 3·2–4·9 (4·0 ± 1·2) μm wide (n = 2). Mid nucleus position measuring 5·8–7·4 (6·6 ± 1·2) μm to anterior, and 5·4–5·6 (5·5 ± 0·1) μm to posterior.

Fig. 3. Hepatozoon involucrum n. sp. in the reed frog Hyperolius marmoratus. (A and B) Trophozoite. (C) Possible meront stage. (D) Possible vacuolated meront stage. (E) Immature gamont stage. (F) Extracellular or free gamont. (G, arrowhead) Mature gamont displaying a recurved tail. (H) Mature gamont, note the expanding parasite nucleus and large parasitophorous vacuole. (I) Double infection of a single erythrocyte. All images captured from the deposited slides (NMB P 467 & 468). Scale bar: 10 µm.
Meronts: rare, irregular in shape, often with a foamy cytoplasm, staining whitish-blue to purple (Fig. 3C and D), and measuring 9·5 µm long × 8·8 µm wide (n = 1). Nucleus containing loosely arranged chromatin, staining pink to purple, measuring 6·8 µm long × 3·7 µm wide (n = 1).
Immature gamonts: elongated with small-recurved tail, within a vaguely visible PV, cytoplasm staining whitish-purple, causing displacement of the host cell nucleus (Fig. 3E). Parasite (including recurved tail) measuring 16·4–23·0 (19·8 ± 1·8) μm long × 4·4–5·7 (5·1 ± 0·4) μm wide (n = 10), PV measuring 14·2–18·4 (15·6 ± 1·3) μm long × 5·2–9·1 (6·5 ± 1·5) μm wide (n = 10). Nucleus rounded, usually situated in the posterior half of the parasite, loosely arranged chromatin, staining purple, and measuring 3·0–7·0 (5·4 ± 1·4) μm long × 2·6–5·6 (3·8 ± 0·9) μm wide (n = 10). Mid nucleus position measuring 10·0–13·7 (11·7 ± 1·4) μm to anterior side, and 6·6–11·1 (8·6 ± 1·6) μm to posterior side (n = 10).
Mature gamonts: elongated and oval, encased in a large PV (Fig. 3F–I); often recurved at both the anterior and posterior poles, and in some cases a clear recurved tail is visible (Fig. 3G arrowhead); infrequent extracellular or free moving gamont (Fig. 3F), as well as single erythrocytes parasitised by two gamonts (Fig. 3I); gamonts cause noticeable displacement of the host cell nucleus. Parasite (including recurved tail) measuring 18·7–25·9 (21·8 ± 1·5) μm long × 4·0–6·3 (5·1 ± 0·5) μm wide (n = 50), PV measuring 16·5–20·9 (18·3 ± 1·0) μm long × 6·3–10·8 (8·3 ± 1·1) μm wide (n = 50). Nucleus elongated or loosely arranged, usually situated in the posterior half of the parasite, loose chromatin strands often visible, staining purely-pink, and measuring 4·8–8·9 (6·4 ± 0·9) μm long × 2·2–4·2 (3·2 ± 0·4) μm wide (n = 50). Mid nucleus position measuring 8·4–19·9 (13·8 ± 1·8) μm to anterior side, and 5·4–11·6 (8·2 ± 1·4) μm to posterior side (n = 50). Parasitaemia of all infected individuals (n = 7) in percentage (%) was 1·0–30·0 (8·0 ± 2·0).
Remarks:
Based on the morphology and morphometrics of peripheral blood stages in Hyp. marmoratus, H. involucrum n. sp. does not conform morphologically to any of the 16 currently recognized Hepatozoon species in African anurans. The only other named species infecting a member of the Hyperoliidae, is H. hyperolii, and can be distinguished from H. involucrum n. sp. based on the shape of the former parasite's gamont. The gamont of H. hyperolii is cylindrical with rounded ends and a long recurved tail folded onto itself in the absence of a prominent PV (see Fig. 6A–C). In contrast the gamont of H. involucrum n. sp. has an elongated and encased gamont, which is often recurved at both the anterior and posterior poles. The mean length and width of H. involucrum n. sp., which includes the parasite's PV, is 18·3 µm long × 8·3 µm wide. Although these mean length measurements do overlap with several species namely, H. faiyumensis, H. francai, H. moloensis and H. neireti, the mean width in combination with the length of these species does not conform. Overall the gamont measurements of H. involucrum n. sp. compare closest to those of H. moloensis (18·8 µm long × 7·8 µm wide), which was described from an unidentified Sclerophrys species in Molo, Kenya (see Hoare, Reference Hoare1920). However, the oval shape, recurved tail and absence of a PV in H. moloensis are distinctive and distinguishable from H. involucrum n. sp. as described above. Similarly, these distinctive characteristics of H. involucrum n. sp, which differentiate it from H. moloensis, also differentiate it from other African anuran species of Hepatozoon.
In South Africa a Hepatozoon species corresponding morphologically to H. involucrum n. sp. was reported from the same host and area in an anuran biodiversity blood parasite survey by Netherlands et al. (Reference Netherlands, Cook, du Kruger, Du Preez and Smit2015), however, this parasite was not formally described or named (see Netherlands et al. Reference Netherlands, Cook, du Kruger, Du Preez and Smit2015, Fig. 2D).
Globally the species that conforms most closely to H. involucrum n. sp. is Hepatozoon nucleobisecans (Shortt, Reference Shortt1917) described from the Indian toad Duttaphrynus melanostictus (syn. Bufo melanostictus). Although the reported gamont length (18·3 µm long) of H. nucleobisecans, including the PV, equals the mean length of H. involucrum n. sp., the width (4·8 µm wide) is almost half. Furthermore, the gamont of H. nucleobisecans is not recurved at both the anterior and posterior poles within the PV (see Shortt, Reference Shortt1917).
Hepatozoon tenuis Netherlands, Cook and Smit n. sp.
Type-host: Afrixalus fornasinii (Bianconi, 1849) (Anura: Hyperoliidae).
Other hosts: Hyperolius argus Peters, 1854; Hyperolius marmoratus (Anura: Hyperoliidae).
Vector: Unknown.
Type-locality: The specimens were collected in St. Lucia on Monzi Farm, KwaZulu-Natal, South Africa (28°26′56″S 32°17′18″E).
Other localities: KwaMbonambi/Langepan, KwaZulu-Natal, South Africa (28°39′43″S 32°10′06″E).
Type-material: Hapantotype, 1× blood smear from A. fornasinii deposited in the protozoan collection of the National Museum, Bloemfontein, South Africa under accession number NMB P 469; parahapantotypes, 1× blood smear from A. fornasinii, and Hyperolius marmoratus; deposited in the Protozoan Collection of the National Museum, Bloemfontein (NMB), South Africa, under accession numbers NMB P 470 and NMB P 471, respectively.
Representative DNA sequences: The 18S rRNA gene sequences have been submitted to the GenBank database under the accession numbers MG041595–MG041599.
ZooBank registration: The Life Science Identifier (LSID) of the article is urn:lsid:zoobank.org:pub:F73407D7-1E08-4C3C-B066-889058B77C4C. The LSID for the new name Hepatozoon tenuis Netherlands, Cook and Smit is urn:lsid:zoobank.org:act:AD607D8B-D43D-49C6-8139-2782306FE2F5.
Etymology: The species epithet is derived from the Latin word tenuis, which means thin or slender. This refers to the long slender shape of the gamont.
Description:
Mature gamonts: slender and elongated, with a pinkish-white staining cytoplasm, within a close-fitting PV visible on the concave side of the gamont (Fig. 4A–C); in some cases a recurved tail is visible (Fig. 4A and D arrowhead); also an occasional extracellular or free moving gamont, (Fig. 3E arrow), as well as a single erythrocyte parasitised by two gamonts (Fig. 4F); gamonts cause obvious displacement of the host cell nucleus. Parasites (including recurved tail when visible) measuring 11·2–16·8 (13·9 ± 1·6) μm long × 3·7– 6·7 (4·8 ± 0·6) μm wide (n = 50), PV measuring 17·8–20·7 (19·4 ± 0·8) μm long × 5·0–7·5 (6·7 ± 0·4) μm wide (n = 50). Nucleus elongated and neatly arranged, usually situated in the posterior half of the parasite, loose chromatin staining purely-pink, and measuring 2·1–5·2 (3·9 ± 0·6) μm long × 1·6–4·9 (10·8 ± 0·9) μm wide (n = 50). Mid nucleus position measuring 4·8–9·4 (6·7 ± 1·1) μm to anterior, and 4·6–10·1 (7·2 ± 1·2) μm to posterior (n = 50). Parasitaemia of all infected individuals (n = 9) calculated in percentage (%) was 1·0–35·0 (6·0 ± 2·0), two (Hyp. argus and Hyp. marmoratus) of the nine infected individuals contained mixed infections the parasite described below.

Fig. 4. Hepatozoon tenuis n. sp. mature gamonts parasitizing erythrocytes in the folding leaf frog Afrixalus fornasinii (A–C) and the reed frogs Hyperolius marmoratus (D) and Hyperolius argus (E and F). (A–C) Close-fitting parasitophorous vacuole, visible on the concave side of the gamont. (A and D, arrowhead) Gamont with a recurved tail. (E, arrow) Extracellular or free gamont. (F) Double infection of a single erythrocyte. All images captured from the deposited slides (NMB P 469–471). Scale bar: 10 µm.
Remarks:
Hepatozoon tenuis n. sp. parasitising A. fornasinii, Hyp. argus and, Hyp. marmoratus, can be distinguished from H. involucrum n. sp., based on the difference in gamont morphometrics. Morphologically, gamonts have an overall similar appearance to H. involucrum n. sp., however, gamonts of H. involucrum n. sp. measure a mean of 21·8 µm long × 5·1 µm wide (n = 50) (PV not included) and a mean of 18·3 µm long × 8·3 µm wide (n = 50) (PV included), as compared with gamonts of H. tenuis n. sp. measuring a mean of 13·9 µm long × 4·8 µm wide (n = 50) (PV not included) and a mean of 19·4 µm long × 6·7 µm wide (n = 50) (PV included). This slender looking parasite can be distinguished from other anuran Hepatozoon species based on the marginally visible PV, as well as often being recurved at both the anterior and posterior poles within the PV.
Hepatozoon thori Netherlands, Cook and Smit n. sp.
Type-host: Hyperolius marmoratus Rapp, 1842 (Anura: Hyperoliidae).
Other hosts: Hyperolius argus; Hyperolius puncticulatus (Pfeffer, 1893) (Anura: Hyperoliidae).
Vector: Unknown.
Type-locality: The specimens were collected in the Kwa Nyamazane Conservancy (KNC), KwaZulu-Natal, South Africa (27°23′35″S, 32°08′41″E).
Other localities: KwaMbonambi/Langepan, KwaZulu-Natal, South Africa (28°39′43″S 32°10′06″E); Amani, Tanzania.
Type-material: Hapantotype, 1 × blood smear from Hyp. marmoratus deposited in the protozoan collection of the National Museum, Bloemfontein, South Africa under accession number NMB P 472; parahapantotype, 1 × blood smear from Hyp. marmoratus; deposited in the Protozoan Collection of the National Museum, Bloemfontein, South Africa, under accession number NMB P 473.
Representative DNA sequences: The 18S rRNA gene sequences have been submitted to the GenBank database under the accession numbers MG041600–MG041603.
ZooBank registration: The Life Science Identifier (LSID) of the article is urn:lsid:zoobank.org:pub:F73407D7-1E08-4C3C-B066-889058B77C4C. The LSID for the new name Hepatozoon thori Netherlands, Cook and Smit is urn:lsid:zoobank.org:act:00CD84D9-D6A8-4B41-A048-DFD0DBF4B045.
Etymology: The species epithet is derived from Norse mythology after the hammer-wielding god Thor. This is based on the hammer-like shape of the gamont.
Description:
Immature gamonts: rare, elongated without a visible PV, cytoplasm staining whitish-purple, measured 18·7 µm long by 5·5 µm wide (n = 1), causing displacement of the host cell nucleus and found parasitizing a single erythrocyte together with a mature gamont (Fig. 5A arrow). Nucleus rounded, situated in the posterior half of the parasite, loosely arranged chromatin, staining purple, and measuring 8·1 µm long × 2·7 µm wide (n = 1). Mid nucleus position measured 8·9 µm to anterior side, and 9·8 µm to posterior side (n = 1).

Fig. 5. Hepatozoon thori n. sp. gamonts parasitizing erythrocytes in the reed frogs Hyperolius marmoratus (A–C) and Hyperolius argus (D–F). (A) Double infection of a single erythrocyte, with an immature (arrow) and mature (arrowhead) gamont. (B–F) Prominent hammer-like or boot-shaped parasitophorous vacuole, allowing only a certain portion of the gamont to be visible. (C and D, arrow) Gamont displaying a short recurved tail. (E) Gamont causing the host cell nucleus to lyse. (F) Extracellular or free gamont. All images captured from the deposited slides (NMB P 472 & 473). Scale bar: 10 µm.
Mature gamonts: elongated, causing displacement of the host cell nucleus. Encased in a prominent hammer-like or boot-shaped PV, with a pseudopodial-like projection (Fig. 5A–F); occasionally a short recurved tail is visible (Fig. 5C and D arrow); mature gamonts cause the host cell nucleus to lyse (Fig. 5E); extracellular or free moving gamont, possibly probing to enter new host cell (Fig. 5F). Parasite measuring 11·2–16·8 (13·9 ± 1·6) μm long × 3·7– 6·7 (4·8 ± 0·6) μm wide (n = 50), with the PV measuring 17·8–20·7 (19·4 ± 0·8) μm long × 5·0–7·5 (6·7 ± 0·4) μm wide (n = 50). Parasites, including the recurved tail (see Fig. 5C and D arrow), measuring 19·1–21·7 (20·4 ± 1·1) μm long (n = 5). Nucleus elongated or loosely arranged, usually situated in the posterior half of the parasite, loose chromatin strands often visible, staining purely-pink, and measuring 2·1–5·2 (3·9 ± 0·6) μm long × 1·6–4·9 (10·8 ± 0·9) μm wide (n = 50). Mid nucleus position measured 4·8–9·4 (6·7 ± 1·1) μm to anterior, and 4·6–10·1 (7·2 ± 1·2) μm to posterior (n = 50). Parasitaemia of all infected individuals (n = 6) in percentage (%) was 1·0–21·0 (3·0 ± 2·0), two (Hyp. argus and Hyp. marmoratus) of the six infected individuals contained mixed infections with H. tenuis n. sp.
Remarks:
Hepatozoon thori n. sp. parasitizing Hyp. argus and Hyp. marmoratus can be distinguished from H. involucrum n. sp., H. tenuis n. sp., and other anuran Hepatozoon species based on the distinctive shape of the hammer-like or boot-shaped PV that has a pseudopodial-like projection. The mean length and width of the parasite measure 13·9 µm long × 4·8 µm wide (PV not included) and 19·4 µm long × 6·7 µm wide (n = 50) (PV included). Based on the size and shape, the only other haemogregarine H. thori n. sp. conforms closest to is an unnamed Hepatozoon species (see Fig. 6D and E), measuring a mean of 14·1 µm long × 4·8 µm wide (PV not included) and 20·8 µm long × 6·7 µm wide (PV included). This unnamed species was reported in Hyperolius puncticulatus, from Amani, Tanzania (see Ball, Reference Ball1967) (see below).

Fig. 6. Illustrations of haemogregarine blood parasites in African hyperoliids. (A–C) Hepatozoon hyperolii (Hoare, Reference Hoare1932), described from an unidentified Hyperolius species in Uganda. Redrawn and adapted from Hoare (Reference Hoare1932). (D and E) Unnamed Hepatozoon species reported in Hyperolius puncticulatus, from Amani, Tanzania. Redrawn and adapted from Ball (Reference Ball1967). Scale bar: 10 µm.
Phylogenetic analysis
Amplicons of between 1640 and 1701 nt were derived from H. involucrum n. sp., H. tenuis n. sp., and H. thori n. sp. from the blood of A. fornasinii, Hyp. argus and Hyp. marmoratus. Additionally, sequences of H. ixoxo and H. theileri, were amplified from the blood collected in a previous study (Netherlands et al. Reference Netherlands, Cook and Smit2014a) from S. pusilla and A. delalandii, respectively. The details of sequences used in the analyses are presented in Table 1.
Table 1. List of the sequence (18S rDNA) information used in the current study
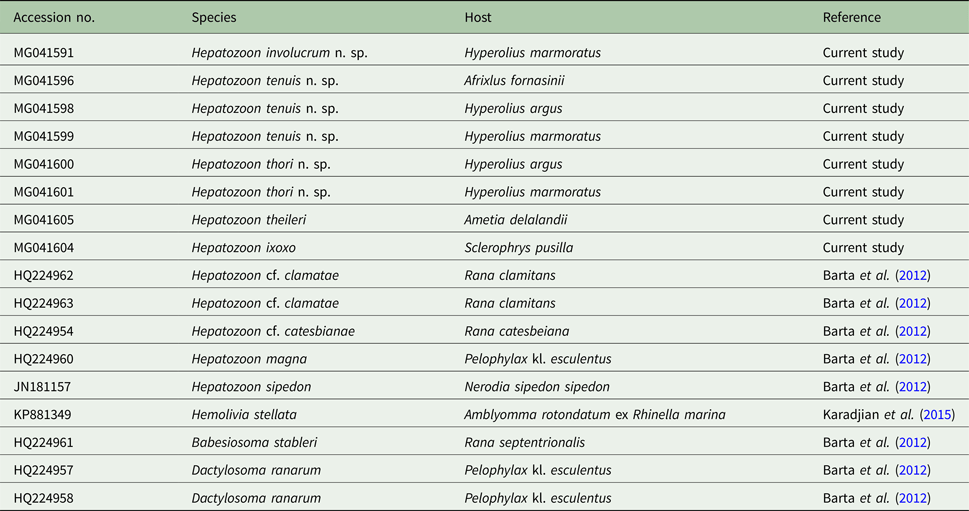
The table includes the GenBank accession number, species, host species and the reference study.
Based on 1497 nt sequence comparisons of the 18S rRNA gene (see Table 2), the interspecific divergence (model-corrected genetic distance) between H. involucrum n. sp. and its closest relative H. tenuis n. sp. was 1·0%. Hepatozoon involucrum n. sp. and H. thori n. sp. had an interspecific divergence of 2·0%, and H. tenuis n. sp. and H. thori n. sp. differed by 1·8%. The interspecific divergence between the Hepatozoon species parasitizing anuran hosts and Hepatozoon sipedon [GenBank: JN181157] was between 7·7 and 10·6%. The intergeneric divergence between the Hepatozoon species parasitising anuran hosts, and Hemolivia stellata Petit, Landau, Baccam and Lainson, 1989 [GenBank: KP881349], B. stableri [GenBank: HQ224961] and D. ranarum [GenBank: HQ224957; HQ224958] were between 4·9–5·8%, 8·8–9·6% and 8·5–9·7%, respectively (Table 2).
Table 2. Estimates of divergence between partial 18S rDNA sequences from the haemogregarine species used in the current study

Distance matrix showing ranges for the model-corrected genetic distances between the sequences. Alignment length 1497 nt. Genetic distances shown as percentage (%).
For the phylogenetic analyses the topologies of both the BI and ML trees were similar. The analyses showed Hemolivia stellata [GenBank: KP881349] as a well-supported sister taxon to the Hepatozoon species cluster, with H. sipedon [GenBank: JN181157] shown to be a sister species to a well-supported monophyletic clade comprising Hepatozoon species isolated from anuran hosts. The Hepatozoon species isolated from African and North American anurans formed two well-supported monophyletic clades, respectively, and were separate from the European species H. magna [GenBank: HQ224960]. The African Hepatozoon clade represents a polytomy with H. involucrum n. sp. and H. tenuis n. sp., forming a well-supported monophyletic clade and H. ixoxo and H. theileri, forming a poorly-supported clade, nested within this polytomy and separate to H. thori n. sp. (Fig. 7).
Discussion
In the present study, we screened the peripheral blood of 225 individual frogs from nine species within the Hyperoliidae. Six species (A. aureus, A. delicates, Hyp. tuberlinguis, Hyp. pusillus, K. senegalensis and P. maculatus), totalling 205 specimens were found negative for haemogregarine parasites. Only 20 frogs from three species were found positive, namely A. fornasinii (6/14), Hyp. argus (2/39), and Hyp. marmoratus (12/74).
Morphological and molecular data indicate that the haemogregarines parasitising these hosts represent three distinct species of Hepatozoon, herein described as H. involucrum n. sp. parasitising Hyp. marmoratus; H. tenuis n. sp., parasitising A. fornasinii, Hyp. argus and Hyp. marmoratus; and H. thori n. sp. parasitising Hyp. argus and Hyp. marmoratus. Mature gamonts of H. involucrum n. sp. are characterized by the prominent PV encircling the large gamont, as well as the recurved ends of both poles of the gamont. When compared with H. tenuis n. sp., the overall appearance and characteristics are similar, except for a difference in size of the gamont and PV. The interspecific divergence between these two species is 1·0%. This has been shown in several studies to correspond to species-level differences in haemogregarines and for the slow evolving 18S rRNA marker (see Barta et al. Reference Barta, Ogedengbe, Martin and Smith2012; Cook et al. Reference Cook, Netherlands and Smit2015b; Borges-Nojosa et al. Reference Borges-Nojosa, Borges-Leite, Maia, Zanchi-Silva, da Rocha Braga and Harris2017). Hepatozoon thori n. sp. can be distinguished from both H. involucrum n. sp. and H. tenuis n. sp. based on the distinctive hammer-like shape of the gamont's PV. The interspecific divergence between H. thori n. sp., H. involucrum n. sp. and H. tenuis n. sp. was 2·0% and 1·8%, respectively.
The only other named species of Hepatozoon infecting a member of the Hyperoliidae is H. hyperolii described in an unidentified Hyperolius species by Hoare (Reference Hoare1932), this parasite being vermicular in shape and folding over on itself within its host erythrocyte (see Fig. 6A–C) and therefore does not conform to any of the Hepatozoon species of the present study. However, Ball (Reference Ball1967) reported a second, but unnamed species in Hyperolius puncticulatus from Amani, Tanzania, and this species conforms both in size and shape to H. thori n. sp. (see Fig. 6D and E). In the current study, we propose that these two species are the same, despite parasitizing different hosts and possibly being geographically isolated. However, to confirm this, molecular data for this species from Amani, Tanzania is required.
In our phylogenetic analysis, Hepatozoon species isolated from anuran hosts formed a well-supported monophyly, separate to other closely related species of Hepatozoon. Furthermore, the African clade formed a monophyly, with H. thori n. sp. separate from the other species within this clade. Hepatozoon involucrum n. sp. and H. tenuis n. sp. form a well-supported monophyletic clade nested within the larger African clade. With an interspecific divergence of 1·0% (model-corrected distance), these two species are closely related, which concurs with their close morphological resemblance. Hepatozoon ixoxo and H. theileri form a less well supported (0·80/75) monophyletic group. The BI statistical information for the bipartitions of this group showed that apart from the 80% probability support, only 13% included H. thori n. sp. as part of this clade and 9% showed H. theileri formed a clade with H. involucrum n. sp. and H. tenuis n. sp., thus explaining the low support of this group. Furthermore, H. ixoxo and H. theileri differ considerably in morphological structure (see Conradie et al. Reference Conradie, Cook, Preez, Jordaan and Netherlands2017), and if compared with the phylogenetic and morphological relationship of H. involucrum n. sp. and H. tenuis n. sp. (as mentioned above), the former two species are not expected to be sister species. This underlines the importance of increased taxon sampling for these parasites, as the addition of more species to this dataset could result in better-supported clades and the polotomy of the African clade could be resolved. Additionally, faster-evolving markers (e.g. mtDNA) may further explain the biogeography and evolutionary history of these species globally. However, to date, only one haemogregarine, H. catesbianae isolated from the frog Rana catesbeiana has mtDNA sequence data available (see Leveille et al. Reference Leveille, Ogedengbe, Hafeez, Tu and Barta2014). Although these markers (mtDNA) may be complementary in providing an evolutionary perspective among these parasite groups, a lot more data are required if we want to use similar sized datasets such as those available for 18S rDNA sequences for haemogregarines, especially in terms of vertebrate host diversity (amphibians, reptiles, fishes, birds and mammals) and geographical distribution.
This study highlights the importance of screening anurans from different families and genera in an effort to increase the known biodiversity of these parasites and types of hosts they infect. This study also shows the significance of providing detailed descriptions or reports of species, localities and host records, as we were able to link a species reported by Ball (Reference Ball1967) with H. thori n. sp. in the current study based on the morphological details he provided. However, although morphological details are important, the use of them in combination with molecular tools provides a richer dataset with which to work, allowing us to infer historical relationships. Furthermore, if molecular data were available for all the currently recognized species of Hepatozoon, those with close morphological characteristics could be correctly distinguished. This stresses the importance of using both of these techniques in combination when describing species, and where possible to provide molecular data for already described species. Future research should, when possible, include faster-evolving genes, identification of possible definitive hosts or vectors and life cycle studies.

Fig. 7. Consensus phylogram of anuran haemogregarines based on 18S rDNA sequences. Tree topologies for both Bayesian inference (BI) and Maximum Likelihood (ML) analyses were similar (represented on the BI tree), showing the phylogenetic relationships for H. involucrum n. sp., H. tenuis n. sp., and H. thori n. sp. (represented in bold), compared to other species of anuran Hepatozoon (with the exception of Hepatozoon sipedon), Hemolivia, and three species from the Dactylosomatidae as outgroup. Clades that neither produced 0·80 posterior probability (BI) or 70 bootstrap (ML) nodal support values were collapsed. Black circles represent 100% support for both BI/ML. The scale bar represents 0·02 nucleotide substitutions per site.
Acknowledgements
We are grateful to Ndumo Game Reserve, the Kwa Nyamazane Conservancy and Pongola River Company, Bonamanzi Game Reserve, KwaMbonambi/Langepan, and St. Lucia Monzi Farm (Charles and Alisa Wally) for permission to collect samples and do fieldwork. Mr. Nico Wolmarans, Dr Wynand Vlok, Dr Leon Meyer and Dr Donnavan Kruger are thanked for their assistance during field work. Ezemvelo KZN Wildlife is thanked for research permits OP 526/2014, OP 839/2014 and OP 4374/2015. Dr Wynand Malherbe, NWU, is thanked for designing the study area map. We would also like to thank Cambridge University Press for permission to reproduce figures 23, 24, 25 from Cecil A. Hoare (1932), On protozoal blood parasites collected in Uganda, Parasitology 24(2), 210-224 (License Number: 4212380359865); and John Wiley and Sons, for permission to reproduce figures 1 & 2 from Gordon H. Ball (1967), Blood Sporozoans from East African Amphibia, Journal of Eukaryotic Microbiology, 14(3), 521-527 (License Number: 4212380887756). This is contribution number #218 from the NWU-Water Research Group.
Financial Support
This paper forms part of a VLIR-UOS TEAM funded project (ZEIN21013PR396), co-funded by a Water Research Commission of South Africa funded study (Project K5-2185, NJ Smit). The financial assistance of the National Research Foundation (NRF) towards CAC is also hereby acknowledged (project SFP13090332476), and ECN who is supported by the DAAD-NRF doctoral scholarship (Grant UID: 108803), and the VLIR-UOS university scholarship (ID 0620854/Contract 000000076310). MPMV is partly supported by the Czech Science Foundation (GBP505/12/G112 – ECIP). Opinions expressed and conclusions arrived at, are those of the authors and are not necessarily to be attributed to the funding bodies.