Introduction
Weeds are a significant threat to agricultural production and can reduce corn yields by up to 34% (Oerke Reference Oerke2006). Although herbicides are currently the most widely used technique for weed control, a reduction of the use and risk of chemical pesticides by 50% by 2030 is proposed as a part of the European Union (EU) Green Deal (Tataridas et al. Reference Tataridas, Kanatas, Chatzigeorgiou, Zannopoulos and Travlos2022). The reliance on chemical weed control and a lack of new herbicide modes of action (MOAs) on the market have resulted in an increased number of cases of herbicide resistance in numerous weed species in Europe (Beckie Reference Beckie2020).
However, even in the absence of herbicide-resistant weed populations, herbicide applications may sometimes provide inadequate levels of efficacy on certain weeds. Potential reasons for a reduced herbicide field efficacy are improper application equipment (Butts et al. Reference Butts, Barber, Norsworthy and Davis2021); a suboptimal weed growth stage at the time of herbicide treatment (Reinhardt Piskackova et al. Reference Reinhardt Piskackova, Reberg-Horton, Richardson, Jennings and Leon2020); and unsuitable climatic conditions, such as inadequate humidity, temperature, precipitation, and wind speed prior to, during, and after herbicide treatment (Varanasi et al. Reference Varanasi, Prasad, Jugulam and Sparks2016). Adverse weather conditions, such as drought prior to herbicide treatment, may enhance plant leaf cuticle thickness, reducing herbicides’ penetration into leaves (Shaner and Beckie Reference Shaner and Beckie2014). To obtain a maximum level of herbicide efficacy, the negative impact of the mentioned parameters should be considered and minimized.
In addition, intensive work has been conducted on improving the characteristics or formulations of herbicide products to achieve higher herbicide efficacy when applying either recommended or reduced herbicide dose rates and to avoid or delay the evolution of herbicide resistance. Likewise, significant efforts have been made in developing new techniques for herbicide application that enable adequate herbicide deposition on the treated surface by minimizing herbicide off-target movement (Vieira et al. Reference Vieira, Butts, Rodrigues, Golus, Schroeder and Kruger2018). For example, the adoption of dicamba-tolerant crop cultivars in the United States has led to herbicide applications requiring extremely or ultra-coarse spray droplets to avoid dicamba particle drift (Sousa Alves et al. Reference Sousa Alves, Kruger, da Cunha, de Santana, Pinto, Guimarães and Zaric2017). Also for other herbicide active ingredients, spray drift continues to be a major problem, causing herbicide damage to nontarget vegetation (Cederlund Reference Cederlund2017; Felsot et al. Reference Felsot, Unsworth, Linders, Roberts, Rautman, Harris and Carazo2010). Farmers have therefore adopted different drift-reducing spray application techniques, such as use of drift-reducing nozzles. These nozzles are designed to create larger droplets at the same flow rate and operating pressure as standard flat-fan nozzles (Ferguson et al. Reference Ferguson, O’Donnell, Chauhan, Adkins, Kruger, Wang, Urach Ferreira and Hewitt2015). Although large droplets are less prone to drift, they can be more easily shed from the target surface, resulting in a reduced herbicide efficacy (Knoche Reference Knoche1994). Therefore the addition of adjuvants, such as surfactants and oil concentrates, into herbicide tank mixtures is commonly proposed to increase spray droplet retention and spreading on the plant leaf surface and to improve herbicide penetration through the weed plant cuticle (Creech et al. Reference Creech, Henry, Fritz and Kruger2015; Moraes et al. Reference Moraes, Butts, Anunciato, Luck, Hoffmann, Antuniassi and Kruger2021; Sousa Alves et al. Reference Sousa Alves, Kruger, da Cunha, de Santana, Pinto, Guimarães and Zaric2017). In addition, adjuvants are used in herbicide tank mixtures to reduce spray drift by changing the physicochemical properties of the spray solution (Bellinder et al. Reference Bellinder, Arsenovic, Shah and Rauch2003).
A large number of different adjuvant types exist, but some of the most frequently used are non-ionic surfactant (NIS) adjuvants and mineral fertilizer ammonium sulfate (AMS). According to Price et al. (Reference Price, Kelton and Sarunaite2015), NIS adjuvants primarily influence the ability of herbicides to penetrate the weed leaf’s waxy cuticle. Adding NIS adjuvants to the tank mix reduces the surface tension of water on the plant, spreading in a wet, thin layer over the waxy leaf surface, thus allowing the herbicide formulation to enter into the plant. AMS is an acidic nitrogen fertilizer that is commonly used in tank mixtures with glyphosate and glufosinate as a water conditioner as it overcomes the antagonistic effect of sodium, calcium, and magnesium ions from hard water (Zollinger et al. Reference Zollinger, Nalewaja, Peterson and Young2010). Furthermore, ammonium ions from AMS may increase herbicide absorption (Bunting et al. Reference Bunting, Sprague and Riechers2004).
In Serbia, weed control in corn is a major challenge due to increasing problems with highly dominant weed species and herbicide resistance evolution. The first cases of resistance to acetolactate synthase (ALS) and/or acetyl-CoA-carboxylase herbicides in Serbia have already been confirmed for johnsongrass, redroot pigweed (Amaranthus retroflexus L.), and common ragweed (Ambrosia artemisiifolia L.) (Vrbničanin Reference Vrbničanin2020), but resistance is yet less widespread than it is in other European countries (Heap Reference Heap2022). In Serbia, corn grown in monoculture has resulted in a dominance of johnsongrass in many weed populations (Simić et al. Reference Simić, Dragičević, Brankov and Šenk2020). Additionally, recent climate change has led to an increased frequency of certain weed species across Europe (Krähmer et al. Reference Krähmer, Andreasen, Economou-Antonaka, Holec, Kalivas, Kolářová, Novák, Panozzo, Pinke, Salonen, Sattin, Stefanic, Vanaga and Fried2020). Among other factors, the impact of climate change on weeds may result in geographic range expansions of specific weed species to new geographical regions (Ramesh et al. Reference Ramesh, Matloob, Aslam, Florentine and Chauhan2017). This applies especially to weed species requiring elevated temperatures or showing a higher drought tolerance, such as johnsongrass, jimsonweed (Datura stramonium L.), and barnyardgrass [Echinochloa crus-galli (L.) P. Beauv.] (Cavero et al. Reference Cavero, Zaragoza, Suso and Pard1999; Clements and Ditommaso Reference Clements and Ditommaso2011).
Especially common lambsquarters has become dominant in corn fields in Serbia in recent years, although herbicide resistance has not yet been reported in this species. This increase in abundance is likely caused by warmer temperature conditions in spring favoring common lambsquarters germination and emergence (Tang et al. Reference Tang, Guo, Yin, Ding, Xu, Wang, Yang, Xiong, Zhong, Tao and Sun2022). Together with johnsongrass, common lambsquarters constitute up to 80% to 90% of the weed population in many spring crops in Serbia, such as soybean [Glycine max (L.) Merr.], sunflower (Helianthus annuus L.), and corn (Vranjes et al. Reference Vranjes, Vrbnicanin, Nedeljkovic, Savic and Bozic2019), and efficient control of these species is therefore vital to sustainable corn production.
Currently, ALS-inhibiting herbicides, such as the systemic selective sulfonylurea nicosulfuron, are most commonly used for chemical weed control in Serbia. To maximize herbicide efficacy for control of johnsongrass and common lambsquarters, careful selection of nozzle types and the addition of adjuvants to the herbicide tank mix are required (Butts et al. Reference Butts, Barber, Norsworthy and Davis2021). In addition, nozzle and adjuvant selections are important to avoid drift of ALS inhibitors to neighboring crops, such as soybean, that exhibit a high potential for crop injury (Bailey and Kapusta Reference Bailey and Kapusta1993). In Serbia, recommendations on herbicide application so far rarely suggest the addition of adjuvants, unlike, for example, in the United States, where labels propose the addition of certain adjuvants to improve and stabilize herbicide efficacy (Shaner Reference Shaner2014). However, some products do not work well without an adjuvant, and labels require that one be added to the tank, representing a minority of registered herbicides. Still, in Serbia, recommendations for a majority of herbicides do not include adding adjuvants into the tank (Plant Protection Society of Serbia 2022). Some of the reasons for this might be found in satisfactory weed control using herbicides, as until now, there had been no need to optimize herbicide efficacy by adding adjuvants. A better understanding of the impact of nozzle type and use of adjuvants on herbicide efficacy would therefore help to improve control of dominant weed species and minimize herbicide resistance development. Thus this study sought to determine the impact of adjuvant (NIS adjuvants vs. mineral fertilizer AMS) and nozzle type (drift-reducing nozzles vs. flat-fan nozzles) on nicosulfuron efficacy against johnsongrass and common lambsquarters in corn.
Materials and Methods
Field Experiment
A 3-yr field experiment was conducted at the experimental field of the Maize (Corn) Research Institute “Zemun Polje,” Belgrade, Serbia (44°52′N, 20°20′E), during 2020 to 2022. A novel corn variety, Stay Green hybrid ‘ZP 707’, was planted at a density of 60,000 plants ha−1, representing a common planting density. The field experiment was conducted on a slightly calcareous chernozem (USDA-NRCS 2019) that contained 32%, 15%, and 53% of clay, silt, and sand, respectively (0- to 30-cm layer). Organic matter content was 3.3%, and the 30 cm topsoil showed 0.21% total N, 1.9% organic C, 14 mg soil-available P, 31 mg of extractable K, 9.7% total CaCO3, and pH 7.8 at the beginning of the experiment in 2020.
The experimental station has 20 ha of arable land, separated into two large plots. Corn is rotationally planted on one of these plots each year, while winter wheat (Triticum aestivum L.) is grown on the other. Therefore winter wheat was the preceding crop in all experimental years. Herbicide applications on the experimental field in the last 10 yr were characterized by an alternation of different herbicide MOAs (including HRAC Group 1, cycloxidim; Group 2, amidosulfuron, metsulfuron-methyl, tribenuron-methyl, nicosulfuron, foramsulfuron; Group 3, pendimethalin; Group 4, 2,4-D; Group 15, acetochlor; and Group 27, isoxaflutole, mesotrione). No herbicide-resistant weeds were observed on the experimental fields before the start of the experiment.
To investigate the impact of adjuvant and nozzle type on nicosulfuron efficacy, two different nozzle types and two adjuvants were tested (Tables 1 and 2). Two 110° flat-fan nozzles from the TeeJet® brand, including Extended Range (XR) TeeJet® and Turbo TeeJet® induction (TTI) (TeeJet® Technologies, Glendale Heights, IL, USA), were selected for use in the study. The two nozzles were chosen to represent a traditional flat-fan nozzle (XR) and an air induction nozzle (TTI), which produce different droplet sizes, influencing coverage and possible off-target movement of the spray solution. Dependently on pressure and orifice size, an XR nozzle produces fine to medium droplets, which provide higher leaf coverage but also a higher number of driftable droplets. In contrast, a TTI nozzle produces ultra-coarse to extremely coarse droplets, resulting in a lower drift potential and lower leaf coverage. We used an orifice size of 0.2 for XR and TTI nozzles at an application pressure of 275.8 kPa.
Table 1. Adjuvants used in the field experiment in 2020 to 2022.

a 20% N + 24% S, WG.
b 349 g/l oil (fatty acid esters) and 209 g/l alkoxylated alcohols–phosphate esters, EC.
Table 2. Nozzle type treatments used in the field experiment in 2020 to 2022. a

a Abbreviations: VMD, volume median diameter according to the American Society of Agricultural Engineers (ASABE) S572.1; XR, Extended Range.
Two adjuvants most commonly used in Serbia were selected for comparison in this study. The NIS is an adjuvant that increases the spreading and wetting area of droplets on plant leaves by reducing surface tension (Castro et al. Reference Castro, Ojeda and Cirelli2014). AMS is commonly used as a water conditioner, as the ammonium ion can associate with the herbicide active ingredients, which may increase herbicide absorption into the leaf.
Nicosulfuron (Motivell Extra 6 OD, 60 g ai L−1, Belchim, Londerzeel, Belgium) was applied at 45 g ai ha−1 (recommended field rate in Serbia) testing all possible combinations of nozzle type and adjuvants. In addition, nicosulfuron was applied testing both nozzle types without an adjuvant. Therefore six different herbicide treatments were studied in total (3 adjuvant treatments × 2 nozzle types; Table 2). In addition, a weed-free (WF) treatment (manually kept free of weeds) and an untreated control plot without any herbicide treatment (C) were included.
The experiments were set up as a randomized complete block design with four replications. Individual plot size was 24.5 m2 (4.9 × 5 m), and each plot contained seven corn rows spaced 0.7 m apart.
Nicosulfuron treatments were applied in May every year when corn plants had developed 5 to 6 true leaves (15 to 16 BBCH) using a CO2 backpack sprayer with a four-nozzle boom with either XR or TTI nozzles, calibrated to deliver a spray volume of 140 L ha−1 of solution at 275.8 kPa (Table 3). At given pressure and nozzle orifice, an XR nozzle produces fine droplets, whereas a TTI nozzle produces ultra-coarse droplets. Weeds present on the experimental site before the start of the experiment were controlled by the seedbed preparation before corn seeding in April. At the time of herbicide application, common lambsquarters showed 2 to 4 leaves in 2020 and 2021 and 4 to 6 leaves in 2022. Johnsongrass had developed 2 to 3 leaves in all three years at the time of herbicide application.
Table 3. Date of corn sowing and harvest, nicosulfuron application, and environmental conditions at nicosulfuron application in the three experimental years.

Meteorological Conditions
The first experimental year (2020) was characterized by an optimal precipitation for corn development, whereas the two following consecutive years (2021 and 2022) showed a very low amount of precipitation after sowing (Table 4). In all three years, young corn plants received sufficient water in April and May.
Table 4. Air temperatures (C, monthly average) and precipitation (mm, totals) during April to September of the three experimental years, including the 10-yr average (2010 to 2019) for the Zemun Polje, Serbia, location.

a Precipitation until September 9.
b Precipitation until September 17.
c Precipitation until September 8.
Assessment of Herbicide Efficacy
Based on visible weed assessments after weed emergence, johnsongrass and common lambsquarters were uniformly present across all plots, dominating the weed populations by more than 90% in all plots. During the 3-yr experimental period, johnsongrass emerged only from seeds but not from rhizomes at the experimental field. Herbicide efficacy (percentage in relation to the untreated control plot) was evaluated on a weekly basis 7, 14, and 21 days after the nicosulfuron treatments (DAT) for the whole plot level. Visual estimations of herbicide efficacy were based on a percentage scale, where 0 represents no efficacy and 100 represents total weed mortality. Whole plot canopy cover (%) was analyzed at 21 DAT before the herbicide efficacy rating using the CANOPEO cell phone application (Division of Agricultural Sciences and Natural Resources, Oklahoma State University App Center, Stillwater, OK, USA). CANOPEO is an image-based application used to accurately determine the percentage of green canopy cover by classifying and counting pixels representing green canopy in the image. Fractional green canopy cover ranges from 0% (no green canopy cover) to 100% (complete green canopy cover). One picture was taken per plot using a cell phone at 1.5 m height from the plot using a tripod, at an angle of 45°. After the last herbicide efficacy assessment (21 DAT), the numbers of individual johnsongrass and common lambsquarters plants per square meter were counted, and plants were uprooted. After drying (60 C) of the plant samples to a constant mass, biomass dry weight of each species per square meter was recorded. The biomass data were converted into percentage of biomass reduction compared to the untreated control. At harvest, corn grain yield (1,000 kg ha−1) was recorded from the two center rows and calculated at 15.5% moisture content.
Statistical Analyses
The data obtained were processed using the statistical package STATISTICA 8.0 for Windows (TIBCO Software Inc., Palo Alto, CA, USA). Adjuvant and nozzle type as well as year were included as fixed effects. The differences between the treatments were determined by three-way analysis of variance (ANOVA), with mean separations made at the α = 0.05 level using Tukey’s post hoc test.
Results and Discussion
Herbicide Efficacy
Common Lambsquarters
The herbicide efficacy was evaluated on a weekly basis at 7, 14, and 21 DAT (Figures 1 and 2). For common lambsquarters control, nozzle type had a significant impact on nicosulfuron efficacy over time (Figure 1). At 14 and 21 DAT, nicosulfuron treatments without an adjuvant (N/A) provided higher efficacy when the XR (50% to 92%) compared to the TTI nozzles (29% to 91%) were used. Addition of the NIS adjuvant provided the highest nicosulfuron efficacy for both nozzle types at all assessment dates and in all experimental years, with efficacy ratings of up to 90% at 21 DAT in 2020 and 2021. No difference in nicosulfuron efficacy between the two nozzle types was observed when the NIS adjuvant was used. Addition of the AMS adjuvant reduced nicosulfuron efficacy on common lambsquarters for both tested nozzles and across all three years at 14 and 21 DAT. At 7 DAT, the efficacy of nicosulfuron with the AMS adjuvant was very low (6% to 7%) and similar to the application of nicosulfuron without an adjuvant (N/A). In contrast, addition of the NIS adjuvant resulted in a nicosulfuron efficacy of 30% (2020 and 2021) up to 50% (2022) at 7 DAT, independently of the nozzle type. The development of nicosulfuron efficacy over time was similar in 2020 and 2021, whereas a lower efficacy was observed in 2022, especially for the N/A and AMS treatments.

Figure 1. Common lambsquarters control 7, 14, and 21 d after nicosulfuron treatments. Means followed by the same letter at 21 DAT do not differ between the different adjuvants within a year and date of assessment using Tukey’s test at α = 0.05. Abbreviations: N/A, no adjuvants; AMS, ammonium sulfate adjuvant; NIS, non-ionic surfactant; XR, Extended Range TeeJet®; TTI, Turbo TeeJet® induction.

Figure 2. Johnsongrass control 7, 14, and 21 d after nicosulfuron treatments. Means followed by the same letter at 21 DAT do not differ between the different adjuvants within a year using Tukey’s test at α = 0.05. Abbreviations: N/A, no adjuvants; AMS, adjuvant; NIS, non-ionic surfactant; XR, Extended Range TeeJet®; TTI, Turbo TeeJet® induction.
Johnsongrass
On the contrary, lower variation in nicosulfuron efficacy for the different adjuvants and nozzle types was recorded for johnsongrass control (Figure 2). No significant differences in nicosulfuron efficacy between the two selected nozzles were recorded at 7, 14, and 21 DAT. The type of adjuvant had a significant impact on nicosulfuron efficacy at 7 and 14 DAT, with NIS improving nicosulfuron efficacy. The lowest nicosulfuron efficacy was recorded with the AMS adjuvant in all years, at 7 DAT, except for using the XR nozzle in 2021. At 14 DAT, small differences in nicosulfuron efficacy between nozzle types and adjuvants were present, while at 21 DAT, a very high efficacy (>97%) was achieved for all treatments.
Weed Biomass Reduction
The type of adjuvant and nozzle as well as the experimental year showed a significant effect on the biomass reduction at 21 DAT for both common lambsquarters and johnsongrass (Table 5). The two-way interactions between adjuvant and nozzle (P < 0.001), adjuvant and year (P = 0.002), and nozzle and year (P < 0.001) were significant for common lambsquarters, while no significant two-way interactions were recorded for johnsongrass. The three-way interaction among adjuvant, nozzle, and year was not significant for both species.
Table 5. ANOVA for effects of adjuvants, nozzle type, year, and their interaction on percentage of biomass reduction after nicosulfuron application in common lambsquarters and johnsongrass (21 DAT).

Across all years, the biomass reduction in common lambsquarters measured 21 DAT varied from 53.9% to 97.7% (Table 6). The effect of nozzle type was not significant only in 2021, when application of nicosulfuron with either XR or TTI nozzles resulted in a similar biomass reduction when averaged over the three adjuvant treatments (87% and 86%, respectively). In general, adding the NIS adjuvant increased the biomass reduction of common lambsquarters independently of the nozzle type used. In comparison to nicosulfuron without an adjuvant (N/A), the addition of the NIS adjuvant increased common lambsquarters biomass reduction by up to 8% for the XR nozzle and by up to 27% for the TTI nozzle (2022). No differences in biomass reduction between the two nozzle types were observed when the NIS adjuvant was added in 2020 and 2021. In contrast, adding AMS did result in a decreased biomass reduction compared to sole nicosulfuron application across all years. The highest average antagonism between nicosulfuron and AMS was estimated in 2022, when common lambsquarters biomass was reduced by 19% compared to the treatment without adjuvants when averaged for both nozzle types (Table 6).
Table 6. Percentage of common lambsquarters and johnsongrass biomass reduction influenced by adjuvants and nozzle types at 21 DAT in the three experimental years. a, b, c

a Values for 2022 for common lambsquarters were not compared since a significant interaction of nozzle type vs adjuvants was present. For common lambsquarters, nozzle type did not produce any significant effects on the percentage of biomass reduction in 2021, while no significant effects were observed for johnsongrass biomass reduction in 2022.
b Mean values were compared within individual experimental years. Means followed by the same letter, lowercase in the row and uppercase in the column within treatments, do not differ using Tukey’s test at α = 0.05.
c Abbreviations: AMS, ammonium sulfate; N/A, no adjuvant; NIS, non-ionic surfactant; TTI, Turbo TeeJet® induction; XR, Extended Range TeeJet®.
The highest johnsongrass biomass reduction was observed in 2022. The efficacy ranged from 92% when adding AMS to 97% for no adjuvants (N/A) to 98% when adding the NIS adjuvant. In the same year, nozzle selection did not significantly affect johnsongrass biomass reduction. Contrarily, nozzle effect was significant in 2020 and 2021, when the biomass reduction average was 15% and 8% higher across all treatments, respectively, when nicosulfuron was sprayed using the XR nozzle compared to TTI. Similar to the results observed for common lambsquarters, addition of AMS as an adjuvant had an antagonism effect on johnsongrass biomass reduction, with the highest degree in 2021, up to 28% compared to sole nicosulfuron (N/A). Addition of NIS adjuvant increased biomass reduction when nicosulfruon was applied with the TTI nozzle to a higher degree compared to the XR nozzle compared to sole nicosulfruon.
Our results indicate that biomass reduction for both weed species at 21 DAT was influenced by nozzle type in 2020, whereas in 2021 and 2022, significant effects were observed only for either johnsongrass or common lambsquarters (Table 6). These findings support previous studies suggesting that there are often no consistent effects of different nozzle types on weed control level for herbicides other than nicosulfuron (Carter et al. Reference Carter, Prostko and Davis2017; Ferguson et al. Reference Ferguson, Chechetto, Adkins, Hewitt, Chauhan, Kruger and O’Donnell2018; Moraes et al. Reference Moraes, Butts, Anunciato, Luck, Hoffmann, Antuniassi and Kruger2021). However, the use of adjuvants showed a significant effect on nicosulfuron efficacy for common lambsquarters control, and adding the NIS adjuvant to nicosulfuron increased the control level independently of the nozzle type used. Improved efficacy of herbicides used with NIS adjuvants was also reported for clethodim used for control of volunteer corn (Obradović et al. Reference Obradović, Kruger, Vrbničanin, Henry and Vieira2017). NIS adjuvants are surfactants that aim to reduce the surface tension of the water molecule, which further increases spreading and wetting areas on weed leaves (Castro et al. Reference Castro, Ojeda and Cirelli2014). Although the highest biomass reduction for both species at 21 DAT was obtained when adding NIS to nicosulfuron, johnsongrass biomass reduction after nicosulfuron and NIS was lower only in the first year (2020) of the experiment compared to sole nicosulfuron (84%) (Table 6). A lower efficacy of nicosulfuron without an adjuvant on common lambsquarters was observed when using the TTI compared to the XR nozzle (biomass reduction from 74% and 75% in 2020 and 2021, respectively). To reduce spray drift, applicators can spray herbicides with TTI nozzles, which produce coarse spray droplets. Because common lambsquarters has a waxy surface that increases the risk of large droplets slipping from the leaf surface (Burghardt et al. Reference Burghardt, Friedmann, Schreiber and Riederer2006), the lower nicosulfuron efficacy observed with TTI nozzles can be attributed to a lower herbicide uptake due to runoff of the spray solution from the plant surface. Meyer et al. (Reference Meyer, Norsworthy, Kruger and Barber2016) reported that coarser droplet sizes together with a low spray volume (94 L ha−1) could reduce efficacy of glyphosate and glufosinate on barnyardgrass, potentially explaining lower nicosulfuron efficacy when using the TTI nozzle. NIS adjuvants can increase herbicide penetration and absorption through the cuticle and improve the homogeneity of spray coverage on both waxy and hairy leaf surfaces (Xu et al. Reference Xu, Zhu, Ozkan, Bagley and Krause2011). By increasing herbicide leaf coverage and penetration, the addition of NIS adjuvants increased nicosulfuron efficacy for common lambsquarters control in our study, especially for the TTI nozzles. The addition of NIS adjuvants may therefore enable the use of drift-reducing nozzles for nicosulfuron application without jeopardizing herbicide efficacy.
In our study, adding AMS decreased nicosulfuron efficacy for both weed species. Depending on the weed species, the biomass reduction was up to 28% lower when AMS adjuvant was used compared so the sole nicosulfuron treatment. AMS comprises sulfuric acid complexed with urea and will reduce spray water pH to approximately 2.0 (Zollinger et al. Reference Zollinger, Howatt, Bernards and Young2016), influencing the efficacy of certain herbicides, for example, glyphosate (Nurse et al. Reference Nurse, Hamill, Kells and Sikkema2008). In giant foxtail (Setaria faberi Herm.) and barnyardgrass, the addition of AMS increased glufosinate efficacy by increasing foliar absorption of the herbicide, but no impact was found for common lambsquarters (Maschhoff et al. Reference Maschhoff, Hart and Baldwin2000). In addition, Young et al. (Reference Young, Knepp, Wax and Hart2003) found no significant effect on common lambsquarters control using glyphosate with an AMS adjuvant in comparison to glyphosate without an adjuvant. Some of the latest research has demonstrated an antagonistic effect for sulfonylurea herbicides when AMS adjuvants are added (Idziak and Woznica 2013). Nicosulfuron is a stable compound in neutral and alkaline environments, whereas it decomposes quickly in acidic environments (Bunting et al. Reference Bunting, Sprague and Riechers2004). The assumingly lower pH and associated decomposition of nicosulfuron caused by the addition of AMS might be a potential reason for the lower efficacy of nicosulfuron + AMS. In our study, the first symptoms of an antagonistic effect of AMS on nicosulfuron efficacy were observed at 7 DAT for johnsongrass and at 14 DAT for common lambsquarters (Figures 1 and 2). The highest negative impact of AMS on nicosulfuron efficacy was detected in the third year (2022) of the experiment, when nicosulfuron + AMS achieved only 10% control efficacy on common lambsquarters, which could be explained by the size of common lambsquarters at the time of nicosulfuron application. In contrast, the antagonistic effect of AMS was lower for johnsongrass.
To optimize herbicide efficacy, application at the optimal growth stage of the weeds is critical (Kudsk Reference Kudsk2008). According to Barros et al. (Reference Barros, Calado, Basch and Carvalho2016), an early timing of iodosulfuron application in spring barley (Hordeum distichon L.) resulted in higher herbicide efficacy, and Vranjes et al. (Reference Vranjes, Vrbnicanin, Nedeljkovic, Savic and Bozic2019) found a higher efficacy of mesotrione for common lambsquarters and velvetleaf (Abutilon theophrasti Medik.) control at early (2 leaves) compared to late growth stage (4 leaves). Also, nicosulfuron as a postemergence herbicide should be applied at early growth stages of the target weed species (2- to 4-leaf stage). In our field experiment, weeds started to emerge earlier in the year 2022 due to favorable weather conditions, and especially common lambsquarters had already reached the 4- to 6-leaf stage at the time of nicosulfuron application. This advanced growth stage of common lambsquarters led to a reduced nicosulfuron efficacy when used either alone or with AMS. In contrast, the addition of NIS adjuvant significantly increased control of common lambsquarters in 2022 despite the higher growth stage of the target plants. These findings highlight the importance of a tailored adjuvant selection and addition, especially under unfavorable conditions for herbicide applications, such as caused by larger target weed plants.
Canopy Cover
At 21 DAT, significant differences were observed in canopy cover (Figure 3). As expected, the significantly highest canopy cover (90.1%) was estimated in the control treatment without any nicosulfuron treatment (C), while the WF treatment showed a canopy cover of 62.0%. Canopy cover under the nicosulfuron treatments including the NIS adjuvant (60.1 and 63.0% using XR or TTI, respectively) did not significantly differ from cover in the WF treatment. Among the nicosulfuron treatments, the highest canopy cover was measured under the AMS treatments (78.6 and 81.0% using XR or TTI, respectively). For nicosulfuron applied without an adjuvant (N/A), canopy cover values were significantly higher than for the WF plots.

Figure 3. Canopy cover as influenced by nicosulfuron applied with different adjuvants and nozzle types (3-yr average). Means followed by the same letter do not differ using Tukey’s test at α = 0.05. The red line signifies values higher than in the WF plots, which actually represent corn cover. Abbreviations: N/A, no adjuvant; AMS, ammonium sulfate; NIS, non-ionic surfactant; XR, Extended Range TeeJet®; TTI, Turbo TeeJet® induction; WF, weed-free; C, control.
Although ALS resistance has been reported in certain weed species in Serbia (Vrbničanin Reference Vrbničanin2020), satisfactory control of common lambsquarters and johnsongrass was achieved with nicosulfuron in our study. This indicates that no resistance to nicosulfuron was present in the experimental populations, most likely due to an intensive rotation of herbicide MOA conducted on the experimental site. However, in cases of lower nicosulfuron efficacy, such as under addition of AMS, individual plants surviving nicosulfuron treatment continued growing and produced seeds. There is evidence that reduced herbicide efficacy caused by either lower dose rates or poor herbicide performance may lead to metabolic resistance development if weeds survive sublethal doses (Gressel Reference Gressel2011; Vieira et al. Reference Vieira, Luck, Amundsen, Gaines, Werle and Kruger2019). This elucidates the importance of increasing herbicide efficacy, for example, by a correct use of adjuvants or nozzle selection to minimize the risk of herbicide resistance evolution.
Given that the EU Commission proposed a substantial reduction of herbicide use in the EU by 2030, one of the solutions for a reduction in herbicide inputs could be regulation of lower herbicide rates together with customized adjuvants. Because adjuvants can improve herbicide efficacy, it may be of interest to label all herbicides to add adjuvants before applications, as has been done in the United States. In fact, the literature reports that reduced herbicide rates by adding adjuvants are as effective as full rates without adjuvants. Javaid et al. (Reference Javaid, Tanveer, Ahmad, Yaseen and Khaliq2012) reported that two adjuvants may increase the efficacy of fluroxypyr + 4-chloro-2-methylphenoxyacetic acid (MCPA), carfentrazone-ethyl, bromoxynil + MCPA, thifensulfuron-methyl, and tribenuronmethyl at reduced rates (0.75X) to control devil’s thorn [Emex spinosa (L.) Campd.]. A half-rate of sulcotrione (225 g ai ha−1) with adjuvant (methylated rapeseed oil) can increase the control of barnyardgrass to the same level as at the full dose. On the other hand, using reduced rates has to be a part of integrated weed management (IWM), because literature also reports negative effects of using lower-than-recommended rates. Gressel (Reference Gressel2011) reported that lower pesticide rates may directly hasten the evolution of resistance. Furthermore, based on IWM principles, using reduced herbicide rates can have positive effects on weed control, particularly when used as an addition to other measures (Nazarko et al. Reference Nazarko, Van Acker and Entz2005).
Corn Grain Yield
The meteorological conditions during the experiment influenced grain yield to the highest degree. The lowest corn grain yields were recorded in the second year (2021), when a reduction in grain yield of more than 80% compared to the first and the third years was observed. Although more than 100 mm of precipitation were recorded in July 2021, all rainfall occurred at the end of the month. Together with high temperatures, this resulted in very low corn pollination. In contrast, 65 mm of rain in 2022 resulted in sufficient available water for normal corn yielding, although more than 40 d without any precipitation were recorded afterward. Therefore yield results are presented for individual years (Table 7). Nozzle selection did not impact grain yield (P > 0.05), whereas significant effects of adjuvants were recorded (P < 0.05). As the AMS had an antagonism effect on nicosulfuron efficacy, yields were lowest in this treatment in all three years due to higher weed densities (37% lower yield compared to the WF treatment in 2022). Yields in the sole nicosulfuron treatment were higher compared to the treatments with AMS and were significantly lower in comparison with NIS. Grain yield under NIS treatments did not differ significantly from yields in the WF treatment. The results indicate that, although nozzle selection influenced weed control level in our study, grain yield as the most important agronomic parameter was not influenced by the choice of nozzle type. Contrarily, the selected adjuvants significantly influenced weed densities and therefore had a stronger impact on corn grain yield. Idziak and Woznica (Reference Idziak and Woznica2014) reported benefits from adding methylated seed oil and ammonium nitrate adjuvants to nicosulfuron to increase both herbicide efficacy and corn grain yields. Kapusta et al. (Reference Kapusta, Krausz, Khan and Matthews1994) indicated that corn grain yield was related to the level and timing of weed control and found that petroleum oil concentrate or a NIS in combination with urea ammonium nitrate increased nicosulfuron efficacy and corn grain yields in comparison to nicosulfuron application without an adjuvant. Additionally, Fluttert et al. (Reference Fluttert, Soltani, Galla, Hooker, Robinson and Sikkema2022) reported methylated seed oil as the most significant factor in enhancing corn weed control when applying tolpyralate 4-hydroxyphenylpyruvate dioxygenase–inhibiting herbicide.
Table 7. Corn grain yield as influenced by nicosulfuron applied with different adjuvants and nozzle types in the three experimental years. a, b
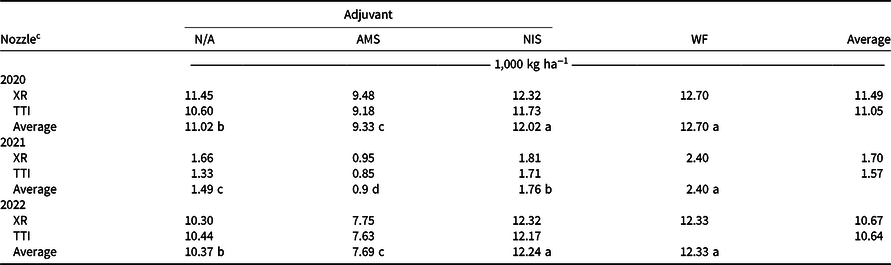
a Means followed by the same letter do not differ using Tukey’s test at α = 0.05.
b Abbreviations: AMS, ammonium sulfate; N/A, no adjuvant; NIS, non-ionic surfactant; TTI, Turbo TeeJet® induction; WF, weed-free; XR, Extended Range TeeJet®.
c Nozzles did not produce any significant effect on corn grain yield.
Practical Implications
Our research shows that nicosulfuron performance can be optimized by the accurate choice of specific adjuvants. The addition of NIS improved nicosulfuron efficacy in our study, while adding AMS adjuvants resulted in lower weed control efficacy. Our study shows that low-drift nozzles mitigating off-target movement of herbicides under less favorable spraying conditions can be used without a decrease in herbicide efficacy given that NIS adjuvants are added to the tank mix.
Acknowledgments
The authors thank the Serbian Ministry of Science and Technological Development for financial support, Grant no. 451-03-68/2020-14/200040, and the Maize Research Institute Zemun Polje, Belgrade, Serbia. The authors also thank all Agro-ecology and Cropping Practices Group staff for their assistance in this project. The authors declare no conflicts of interest.