Introduction
The incidence of chronic pulmonary obstructive disease (COPD), asthma and allergic rhinitis has increased throughout the world. COPD, for example, is now the fourth cause of mortality, and 358 million individuals had asthma in 2015, especially during acute phases of exacerbation (i.e. AECOPD)(Reference May and Li1). Although the Human Microbiome Project (HPM) did not include the lower respiratory tract, it was conducted in 2008 to discover the complete human microbiome content as well as its delicate relationship with the body system in health and disease. Soon after, metagenomics studies evaluating the content and diversity of the human lower respiratory tract microbiome demonstrated that the immune system balances and adjusts the respiratory microbiome as a yin-yang model (symbiosis) so that any disruption of this inter-balance could result in a disease state. Besides, more studies after that demonstrated the impact of microbiome diversity, i.e. dysbiosis during chronic respiratory diseases. In this regard, probiotics as a set of living micro-organisms are used to amend this dysbiosis and re-establish a good microbiome content (symbiosis). Accordingly, probiotics primarily used for gastrointestinal and inflammatory diseases such as antibiotic-associated diarrhoea and inflammatory bowel disease (IBD) are now used for acute and chronic respiratory diseases(Reference Mortaz, Adcock and Folkerts2,Reference Doron, Snydman and Gorbach3) . The underlying concept here is the effect of the intestinal immune system on other body sites such as the lower respiratory system (gut–lung axis). In this regard, probiotics administration (mostly orally) has been now largely evaluated, as promising for the prevention and treatment of acute respiratory infections such as pneumococcal pneumonia and influenza viruses, and, to a lesser extent, for chronic respiratory diseases including COPD, asthma, and allergic rhinitis with the various outcomes. Furthermore, the application of the nasal administration route, as another administration route, for some chronic pulmonary diseases is a new and interesting issue with controversial clinical trial results. Altogether, despite many studies having been carried out, not many studies have reviewed the impact of probiotics therapy for chronic respiratory diseases. The present review aimed to evaluate the clinical and para-clinical applications of orally and nasally administered probiotics for major chronic respiratory diseases, including chronic obstructive pulmonary disease (COPD), asthma and allergic rhinitis. In the present review, the microbiome changes in asthma, allergy and COPD are first characterised, and after evaluating the interaction of the microbiome with the mucosal immune system, therapeutic applications of oral and nasal probiotics for chronic lung diseases are described.
Microbiome changes in asthma and allergy
Asthma is an airway chronic inflammatory disease characterised by coughing, sneezing, wheezing, dyspnoea, and reversible airflow obstruction that is caused by eosinophil and mast cell-secreted inflammatory cytokines(Reference Yawn4). Respiratory allergic diseases such as atopy and allergic rhinitis also have a similar mechanism of pathogenicity in the lung with asthma. Despite currently available therapeutic strategies including avoiding allergens and improving the signs and symptoms through inhaled corticosteroids, β2 agonists, and anti-leukotrienes, asthma still has no specific treatment as well as high healthcare costs during exacerbations(Reference Vézina, Chauhan and Ducharme5). According to the hygiene hypothesis, increased levels of hygiene and antibiotic overuse can interrupt the homeostasis of the body microbiome, promote immune system responses, and increase the risk of allergies and chronic respiratory diseases, mainly due to altering the composition of the microbiome(Reference Kuo, Kuo and Huang6,Reference Omland, Hjort and Pedersen7) . A study showed that the consumption of unpasteurised and untreated cows’ milk is associated with less asthma and allergy development in infants(Reference Okada, Kuhn and Feillet8). The prevalence of asthma would decrease later in childhood for infants with poor hygiene conditions as they are subjected to various populations of micro-organisms(Reference Holt and Sly9). Besides, children with older brothers or sisters or those who have close contact with pets have lower rates of asthma and atopic diseases(Reference Mortaz, Adcock and Folkerts2). Higher exposure of non-pathogenic micro-organisms to the human body in early childhood may lead to higher stimulation and training of the immune system, and, subsequently, reduces inflammatory and allergic reactions in the body. Likewise, studies have demonstrated different lung microbiome compositions of asthmatic patients compared with healthy subjects. For example, studies showed that the colonisation rate of Chlamydia pneumoniae in sputum samples of non-atopic asthmatic patients was higher than that of the healthy population(Reference Specjalski and Jassem10,Reference Hilty, Burke and Pedro11) . In addition, the colonisation rate of Streptococcus pneumoniae and Haemophilus influenzae in upper airways has a positive correlation with persistent wheezing, eosinophilia and higher IgE secretion(Reference Kuo, Kuo and Huang6,Reference Hilty, Burke and Pedro11-Reference Wheatley and Togias13) . Besides, the type and load of colonising bacteria, the exposure period and the genetic background are important. For example, increased loads of airway pathogenic bacteria correlate positively with hypersensitivity(Reference Marsland, Trompette and Gollwitzer12). Therefore, any dysbiosis (replacing a healthy microbiome with bad ones) in airway/gut normal microflora may lead to the colonisation of pathological bacteria that, in turn, can cause inflammatory cascades and disease progression in the lungs(Reference Huang, Sethi and Murphy14).
Interestingly, not only is dysbiosis in the airway microbiome correlated in most cases with asthma and allergic rhinitis, but also dysbiosis in the gut microbiome may have a similar condition. Several studies have demonstrated a significant relationship between gut microbiome composition and the exacerbation or development of allergic diseases(Reference Specjalski and Jassem10-Reference Marsland, Trompette and Gollwitzer12,Reference Huang, Sethi and Murphy14,Reference Bisgaard, Hermansen and Bønnelykke15) . Following Caesarean section delivery, diet and drug consumption during pregnancy, dysbiosis in the gut microbiome can lead to local and systemic allergic diseases in childhood(Reference Penders, Gerhold and Stobberingh16,Reference Dominguez-Bello, Costello and Contreras17) . Cesarean section delivery increases allergy to milk and eggs by twofold(Reference van Nimwegen, Penders and Stobberingh18), and also augments the risk of asthma in the first 6 years of life in children, possibly due to the increased colonisation of Clostridium difficile in the intestine of infants. Another study in infants with food allergies showed a decreased diversity of the gut microbiome with a significant shift toward Enterobacteriaceae rather than Bacteroides (Reference Azad, Konya and Guttman19). The colonising microbiome of a vaginally delivered infant’s skin, mouth and bowel is often similar to that of the mother and correlates with a lower incidence of allergic diseases in childhood(Reference van Nimwegen, Penders and Stobberingh18). How changes in intestinal microbiome content can result in developing or exacerbating conditions in another body organ is not entirely understood. Generally, any disruption in the composition of the gut microbiome (through antibiotics, diet and lifestyle) may result in disrupting the mucosal immune tolerance mechanism, increased inflammatory responses, and disease development(Reference Noverr and Huffnagle20). The biodiversity hypothesis states that the gut microbiome interacts with intestinal immune cytokines affecting lung immune system cells, and diminishes pulmonary inflammation. Hence, more diverse gut microbiota may lead to a lower risk of asthma later in life. As an example, there is a significant relationship between the presence of Acinetobacter spp. on the skin or in the intestinal tract and the lower prevalence of asthma as well as raising IL-10 as an immune tolerance regulator(Reference Marsland, Trompette and Gollwitzer12,Reference Bisgaard, Hermansen and Bønnelykke15) . The role of the respiratory microbiome in developing respiratory diseases is summarised in Fig. 1.
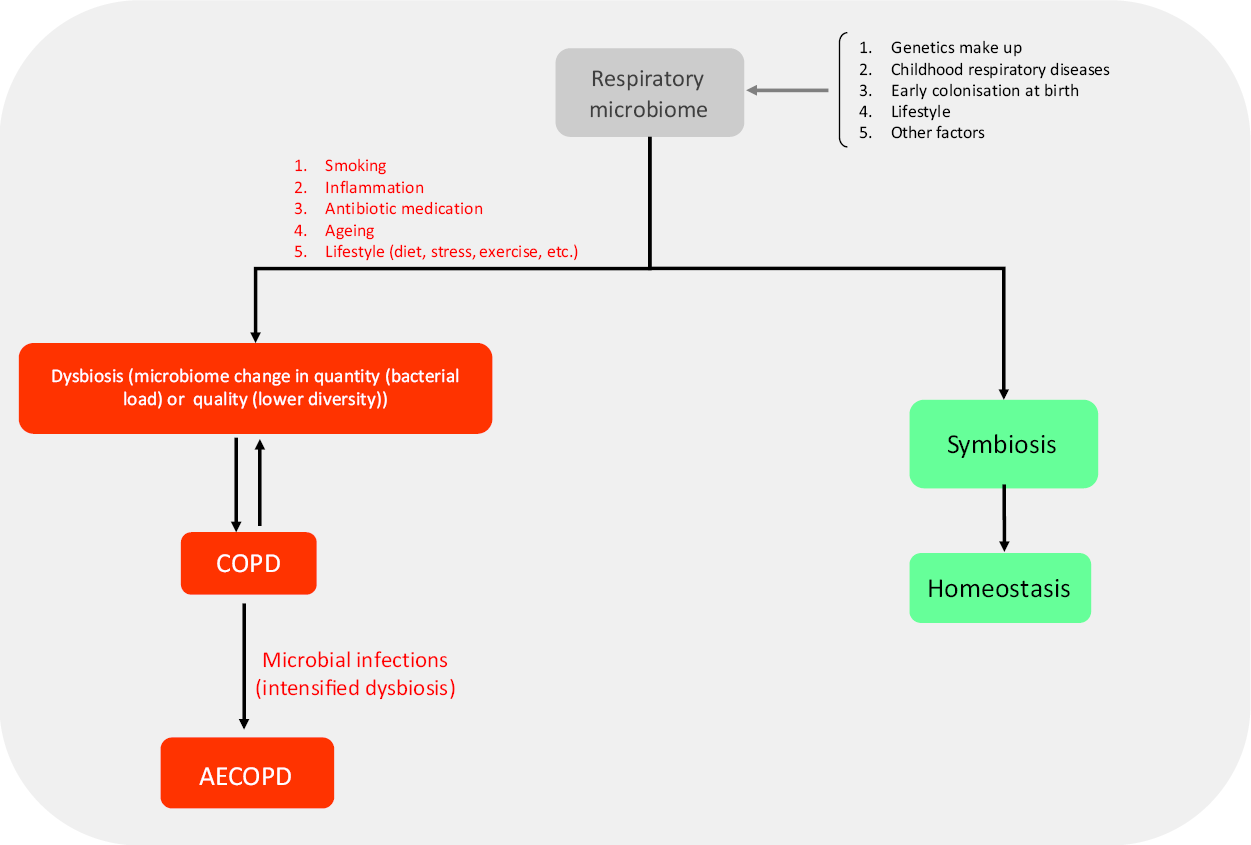
Fig. 1. Role of the respiratory microbiome in developing respiratory diseases. The structure of the respiratory microbiome is based on several factors and has a central role in promoting exacerbations. Several risk factors such as smoking are involved resulting in symbiosis or dysbiosis of the microbiome. At last, homeostasis or inflammation/infection are the outcomes of the immune system–respiratory microbiome interaction. AECOPD may be the possible outcome of this interaction. COPD, chronic pulmonary obstructive disease; AECOPD, acute exacerbation of chronic pulmonary obstructive disease.
Microbiome changes in chronic obstructive pulmonary disease
COPD is a chronic lung disease causing emphysema and airway obstruction with breath shortness, cough and sputum production. The most common cause of the disease is cigarette smoking and air pollutants, to which prolonged exposure can trigger lung inflammatory responses and lead to narrowing small airways and lung tissue destruction(Reference Nathell, Nathell and Malmberg21). It is now evident that respiratory infections are a significant cause of COPD exacerbations(Reference Sethi, Maloney and Grove22,Reference Morris, Beck and Schloss23) , as many studies have shown that the load of the microbiome in COPD patients’ lungs correlates significantly with the severity and progression of the disease. Although the overall structure of the microbiome remains primarily intact in COPD patients, its diversity begins to decrease, and, most often, dysbiosis may present(Reference Saeedi, Salimian and Ahmadi24,Reference Dy and Sethi25) . In a study by Huang et al. (Reference Huang, Sethi and Murphy14) comparing the stable COPD patients with chronic bronchitis patients, no changes were found in the microbial composition of airways. Also, there was no difference in the structure and diversity of the microbiome of sputum before and after an exacerbation, but certain limited types of potentially pathogenic bacteria became dominant, including an increase in Proteobacteria, and a decrease in Actinobacteria, Clostridia and Bacteroides (Reference Huang, Sethi and Murphy14). Also, Garcia-Nuñez et al. (Reference Garcia-Nuñez, Millares and Pomares26) showed that the microbiome diversity of lungs is decreased following COPD progression. Notably, little changes could be seen in the lung microbiome both in pre- and early stages of COPD exacerbations(Reference Huang, Sethi and Murphy14,Reference Huang, Kim and Cox27) . In the study of Galiana et al. (Reference Galiana, Aguirre and Rodriguez28), the microbiome diversity of COPD patients’ lungs was decreased more in the severe stage than in the mild/moderate stage, and H. influenzae was more prevalent in the severe stage, while Actinomycete spp. were dominant in mild/moderate stage. The relationship between lung potentially pathogenic bacteria colonisers and the severity of inflammation may be a key to understanding the pathogenesis of COPD exacerbations. The proliferation of potentially pathogenic bacteria is associated with increased airway inflammation that, in turn, provides a basis for the destruction of lung parenchyma and disease progression(Reference Huang, Sethi and Murphy14,Reference Sethi, Maloney and Grove22) . The ‘vicious cycle’ hypothesis argues that following cigarette smoke or other factors that mediate the disruption of the lung microbiome, an inflammation process starts in the lungs resulting in an imbalance of the protease–antiprotease system. This, in turn, leads to airway obstruction, parenchymal damage, and consequently increased colonisation of pathogenic bacteria in the lungs. Pathological evaluations of COPD airways have also shown an increase in lymphatic follicles, confirming the relationship between microbiome dysbiosis and COPD exacerbation(Reference Huang, Sethi and Murphy14).
Interaction of microbiome with the mucosal immune system
Both the upper and lower parts of the airways possess their specific immune systems. The upper airway is covered with columnar epithelial and goblet cells to deal with airway pathogenic microbes, and, through the mucociliary movements, removes germs(Reference Rose and Voynow29). Also, the mucous lining of these surfaces acts as a physical barrier to prevent the penetration of microbes and contains antimicrobial agents and glycoproteins such as IgA, mucins and phagocyte cells. The upper airway is distinct from the lower airway due to the presence of a wide variety of healthy microflora (mostly symbiotic Firmicutes and Actinobacteria) involved in maintaining the homeostasis of the lower airway region(Reference Lemon, Klepac-Ceraj and Schiffer30). In the lower airways, the density of protective materials is higher so that at least twelve functionally active mucins have been identified to date(Reference Rose and Voynow29,Reference Nakanishi, Morita and Iwashita31) .
The microbiome content helps to improve the regional immune system and functions as the first line of defence against foreign pathogens(Reference Weaver and Kolter32-Reference Stecher, Chaffron and Käppeli34). The lower airway microbiome originates mostly from the upper part (especially the oropharynx) that transiently migrates to the lower regions and then is consistently phagocytosed by the regional immune system (transient but not resident ‘TBNR’)(Reference Saeedi, Salimian and Ahmadi24). The lymphatic tissues of the immune system diffused throughout the mucosal surfaces (including gut-associated lymphoid tissue, bronchial-associated lymphoid tissue and nasal-associated lymphoid tissue) start to develop from birth, mainly under the impact of microbial antigens(Reference Saeedi, Salimian and Ahmadi24,Reference Borchers, Selmi and Meyers35) . In the intestinal mucosa, after taking up and processing foreign antigens by dendritic cells, the immune response is developed as either an immune response or tolerance(Reference Steffen and Berg36,Reference Ibnou-Zekri, Blum and Schiffrin37) . Production of IL-12 and interferon-γ stimulates the T helper 1 (Th1) response (cell-mediated immunity). However, IL-4, IL-5, IL-6 and IL-13 stimulate Th2 responses (humoral immunity); and releasing IL-10 and transforming growth factor-β (TGF-β) can induce dendritic cells to trigger T-regulatory cells(Reference Chieppa, Rescigno and Huang38). The evolution of the immune system starting before birth is mostly mediated through Th2 immune responses, and, after birth, changes toward Th1 responses(Reference Bilsborough and Viney39). From infancy to maturity, the production of Th1 cytokines increases and prevents infancy asthma and allergy induced by the Th2 inflammatory responses. There is a reverse relationship between the serum level of IgE and the interferon-γ concentration(Reference Sharma, Jen and Butler40). The gastrointestinal microbiome is assumed to balance Th1/Th2 responses in the lungs. The lung microbiome promotes naive T cells to differentiate toward Th1 cells and cell-mediated immunity by recognising and attaching to pattern recognition receptors and stimulating dendritic cells to produce a large number of anti-inflammatory cytokines such as TGF-β as well as to decrease serum levels of pro-inflammatory cytokines such as TNF-α and IL-1β(Reference Azad and Kozyrskyj41). The gut microbiome keeps the lung healthy in two ways: (1) it modulates the lung inflammatory responses through shifting Th2 toward Th1 responses; and (2) it promotes T-regulatory responses(Reference Atarashi, Tanoue and Shima42). Several studies have shown that Lactobacillus species induce the expression of IL-12 and TNF-α.
On the other hand, Bifidobacterium species diminish IL-12 and interferon-α levels and increase IL-10 levels(Reference Fink, Zeuthen and Ferlazzo43). Besides, the increased rates of Lactobacillus rhamnosus and L. reuteri in the intestine of IBD patients were associated with increased peripheral T-regulatory cells(Reference Young, Simon and Baird44). Also, the increased growth of L. casei could down-regulate TNF-α and decrease IL-6, possibly due to the ability to reduce activated T cells in the lamina propria(Reference Zeuthen, Christensen and Frøkiær45). This phenomenon, ‘gut–lung crosstalk’, states that the healthy gastrointestinal microbiome plays critical roles in establishing the mucosal immune system homeostasis, resulting in suppressing respiratory inflammatory diseases. Thus, any dysbiosis in the ecological niche may lead to chronic inflammatory diseases(Reference Marsland, Trompette and Gollwitzer12). For example, a study suggested that following dysbiosis in the intestinal microbiome (decrease of Lactobacillus and Bifidobacterium species and increase of Enterococcus and Bacteroides), the inflammatory cytokines IL-1β, TNF-β and IL-8 were increased in the mucus of IBD patients. Furthermore, lamina propria T cells produced high levels of IL-6 that are associated with microbial dysbiosis(Reference Bai, Ouyang and Xiao46-Reference Atreya, Mudter and Finotto48). Although the lung microbiome–mucosal immunity interactions have not been studied as much as the intestinal microbiome, as many studies imply, these findings draw attention to the possibility of administrating probiotic supplements containing a healthy microbiome for the treatment of acute and chronic respiratory diseases(Reference Kuo, Kuo and Huang6-Reference Holt and Sly9,Reference van Nimwegen, Penders and Stobberingh18,Reference Noverr and Huffnagle20) .
Therapeutic applications of oral probiotics in chronic lung diseases
According to the WHO, probiotics are live micro-organisms which, when administered in adequate amounts confer a health benefit on the host(49). Probiotics were used for the first time in 1970 on farm animals to improve the animals’ feeding and survival(Reference Fuller, Fuller, Heidt, Rusch and van der Waaij50). For humans, probiotic administration was first used to transfer a normal microbiome from healthy individuals to irritable bowel syndrome patients (faecal bacterio-therapy)(Reference Borody, Warren and Leis51). Soon, it was demonstrated that by restoring the healthy microbiome in patients and bringing it back to the normal condition, the symptoms of inflammation might be ameliorated(Reference Borchers, Selmi and Meyers35). Afterward, probiotic administration was extensively used in various infections including gastrointestinal and respiratory infections (firstly) as well as some chronic respiratory diseases. In this regard, many studies used probiotics for the treatment of gastrointestinal infections, including acute diarrhoea, antibiotic-associated diarrhoea, traveller’s diarrhoea and IBD(Reference Borody, Warren and Leis51-Reference Ahuja and Khamar57). For example, a study showed that the successful treatment rate of antibiotic-associated diarrhoea patients treated with Lactobacillus GG was 70 % higher than of the controls(Reference Trompette, Gollwitzer and Yadava54). Also, several studies have shown that administrating Lactobacillus GG, Escherichia coli Nissle, and a probiotic mixture can be as effective as the standard therapy in IBD patients(Reference Mallon, McKay and Kirk53,Reference Zigra, Maipa and Alamanos58) . In recent years, clinical trials with probiotics for the treatment of respiratory diseases have also increased dramatically(Reference Elazab, Mendy and Gasana59-Reference Abrahamsson, Jakobsson and Böttcher77). Most of these studies have used oral supplements of Lactobacillus and Bifidobacterium spp. and have mostly reported the reduced severity of inflammatory symptoms in both chronic and acute respiratory diseases(Reference Zigra, Maipa and Alamanos58,Reference Elazab, Mendy and Gasana59,Reference Hojsak, Snovak and Abdović78-Reference Gleeson, Bishop and Oliveira81) . For example, a study by Hojsak et al. (Reference Hojsak, Snovak and Abdović78) showed that infants who received Lactobacillus probiotics were less affected by acute respiratory infections than the control group. Another study also showed that the administration of L. gasseri and Bifidobacterium probiotics could significantly reduce the duration and severity of the common cold (by as much as 2 d)(Reference de Vrese, Winkler and Rautenberg79). A meta-analysis by Barraud et al. (Reference Barraud, Bollaert and Gibot80) evaluating the impact of probiotic administration for critically ill intensive care unit (ICU) patients showed that therapy with probiotics could reduce the duration of ICU admission by up to 2·2 d and decreased the rate of ventilator-associated pneumonia by up to 10 %. In contrast, in some studies, probiotics had no significant impact on reducing mortality rates in ICU patients(Reference Manzanares, Lemieux and Langlois82). For example, in a study by Gleeson et al. (Reference Gleeson, Bishop and Oliveira81) evaluating the effects of a probiotic supplement during 4 months in men and women engaged in endurance-based physical activities on the incidence of upper respiratory tract infections and immune markers, they found that the number of infection episodes was significantly higher in the control group, and saliva IgA was higher in the group administrated probiotics. For more information, clinical trials that administrated probiotics for chronic respiratory diseases are summarised in Table 1. In a meta-analysis including twelve studies on therapy with probiotics in allergic rhinitis patients, administrating oral probiotics could improve at least one clinical sign, decrease the rate of exacerbations, and reduce the administration of allergic rhinitis-relieving medication in nine studies(Reference Elazab, Mendy and Gasana59). Probiotics, as anti-inflammatory agents, can suppress the increase of IgE levels in seasonal allergies and decrease allergic symptoms(Reference Baughman, Thorpe and Staneck83). Furthermore, Lactobacillus GG probiotic consumption for at least 3 months during pregnancy and breast-feeding could reduce the rate of allergic eczema in infants due to the increase of TGF-β in breast milk(Reference Boyle, Ismail and Kivivuori60). Miraglia et al. (Reference Miraglia Del Giudice, Indolfi and Capasso84) applied a bifidobacteria mixture (B. longum BB536, B. infantis M-63, B. breve M-16V) in children with seasonal allergic rhinitis with intermittent asthma through a double-blind randomised trial. They concluded that the bifidobacteria mixture was able to significantly improve allergic rhinitis symptoms and quality of life. Di Pierro et al. (Reference Di Pierro, Basile and Danza85) applied a mixture of Bifidobacterium animalis subsp. lactis BB12 and Enterococcus faecium L3 administered 3 months before or during the development of atopy and concluded that when administered in the 3 months before the development of atopy (as a prophylactic), the probiotics reduced atopy symptoms. However, reduced efficacy was not observed when the mixture was given as the treatment. Another study by Watts et al. (Reference Watts, Cox and Smith86) evaluated in allergic rhinitis the efficacy of a probiotic supplement (probiotik®pur; Ecologic® AllergyCare) administered orally for 8 weeks for the improvement in quality-of-life scores, and change in symptoms, and as a result, a clinically meaningful response was observed in 63 % of participants. In addition, in the study by Choi et al. (Reference Choi, Oh and Choi87), the oral administration of L. plantarum CJLP133 and CJLP243 alleviated birch pollen-induced allergic rhinitis and concluded that the oral administration of probiotics could reduce symptoms of allergic rhinitis in mice by recovering Th1/Th2 balance through increasing the Th1-type immune response. On the other hand, although many studies have demonstrated the beneficial effects of probiotics to reduce inflammatory markers in eczema and allergy patients(Reference Kuo, Kuo and Huang6-Reference Holt and Sly9,Reference Yang, Liu and Yang63,Reference Zuccotti, Meneghin and Aceti64) , some studies have reported that probiotic administration neither prevented eosinophilia nor improved disease symptoms in seasonal allergic rhinitis patients(Reference Taylor, Dunstan and Prescott61,Reference Peng, Li and Yu62) or in children with allergic diseases(Reference Abrahamsson, Jakobsson and Björksten65,Reference Loo, Llanora and Lu66) . It seems that probiotics do not have any effect on immune system mechanisms leading to allergic sensitisation; instead, their major impact is to ameliorate the disease severity by suppressing the inflammatory cells involved in allergy and eczema. Several studies have reported similar results for probiotic administration for the prevention and treatment of allergic diseases and eczema in children. In a review by Cuello-Garcia et al. (Reference Cuello-Garcia, Brożek and Fiocchi67), a decline was reported in the incidence of eczema in children who received probiotics prenatally, albeit there was no such reported effect for other allergic diseases. Ye et al. (Reference Ye, Liu and Wang88) systemically reviewed and meta-analysed the therapeutic effect of probiotics on allergic rhinitis for a total of sixteen clinical trials (randomised controlled trials) involving 1374 patients and concluded that compared with the placebo group, probiotics could effectively reduce the symptoms of allergic rhinitis patients, and different probiotics indicated no differences in improving disease symptoms. Also, a study in 2016 showed similar results confirming the positive effects of probiotic administration on eczema and seasonal allergy(Reference West68). Despite all, some reports have yet found no significant effects of probiotics for improving wheezing, rhino-conjunctivitis, and asthma prevention(Reference Zuccotti, Meneghin and Aceti64,Reference Loo, Llanora and Lu66) . For asthma, since it has a chronic disease nature and is influenced by multiple genetic and environmental factors, these studies have reported different results comparing with allergic diseases. Several clinical trials examining the impact of probiotics on asthma indicated that, in contrast to allergic rhinitis, administration of probiotics by the oral route did not affect asthma(Reference Das, Singh and Shafiq69,Reference Azad, Coneys and Kozyrskyj70) . The subjects of these studies were children and adults with mild to moderate asthma. Bacterial species used as probiotics included L. casei, E. faecalis, L. rhamnosus and L. acidophilus. Also, in studies that examined the risk of food allergy, the administration of probiotic supplements had no significant effect on reducing food allergies or asthma(Reference Azad, Coneys and Kozyrskyj70). Surprisingly, the study of Kalliomäki et al. (Reference Kalliomäki, Salminen and Arvilommi71) monitoring clinical outcomes for 5 years reported that the administration of Lactobacillus GG-containing probiotics even increased the risk of allergy and asthma compared with the placebo group. There are also other studies confirming no clinical efficacy of probiotic administration for asthma patients(Reference Taylor, Dunstan and Prescott61,Reference Wheeler, Shema and Bogle72) . In the meta-analysis by Das et al. (Reference Das, Singh and Shafiq69) probiotics had neither an impact on the prevention of asthma in at-risk infants nor for the treatment of moderate/severe asthmatic patients. However, only one study could find a positive relationship between the administration of probiotics and longer periods of no symptoms(Reference Das, Singh and Shafiq69). On the other hand, some studies comparing cytokine levels and inflammatory responses following the use of probiotics have indicated positive effects. Other studies reported that although probiotic administration had no significant impacts on clinical manifestations of asthma, it could decrease serum levels of IgE(Reference Kopp, Hennemuth and Heinzmann73-Reference Nonaka, Izumo and Izumi75). For example, the study by Chen et al. (Reference Chen, Lin and Jan76) showed that the daily consumption of L. gasseri for 2 months in 6- to 12-year-old asthmatic children had positive effects on both clinical symptoms and cytokine levels. The severity of symptoms as well as the patients’ peak expiratory flow rate were improved. Another study confirmed the increased blood levels of TNF-α, interferon-δ and IL-12 in asthmatic patients(Reference Chen, Lin and Jan76). It is noteworthy that there is contradictory evidence about the time of probiotic consumption and clinical findings. Probiotic consumption was more effective in reducing the risk of asthma and atopic disease while administered either prenatally or postnatally. In addition, the effect of probiotic consumption for reducing serum IgE was absolutely more evident in a long period of follow-up(Reference Kalliomäki, Salminen and Arvilommi71,Reference Abrahamsson, Jakobsson and Böttcher77,Reference Kukkonen, Savilahti and Haahtela89,Reference Pellaton, Nutten and Thierry90) . These results are in agreement with the hygiene hypothesis stating that the lack of exposure to micro-organisms in early childhood can imbalance the Th1/Th2 response and lead to IgE-mediated immune responses. Elevated levels of plasma C-reactive protein (CRP) are related to the risk of eczema and allergy to cows’ milk in children. Probiotic administration can also lead to a decrease in the plasma level of CRP and can lower cows’ milk related eczema and allergy before 2 years old. Also, the plasma concentration of IL-10 (as an anti-inflammatory cytokine that is inversely associated with CRP level) increases after probiotic administration. Probiotics can also induce the secretion of local inflammatory markers that correlate with a lower incidence of IgE-mediated allergic diseases(Reference Nonaka, Izumo and Izumi75,Reference Marschan, Kuitunen and Kukkonen91) . In addition, some studies have reported that extending the period of probiotic consumption can improve therapeutic outcomes. Furthermore, the prenatal administration of probiotics is another factor that increases its effectiveness. Several investigations indicated that prenatal probiotic administration lasting until the neonatal period could reduce the severity of asthma compared with children who received probiotics only after birth(Reference Boyle, Ismail and Kivivuori60,Reference Abrahamsson, Jakobsson and Böttcher77,Reference Dotterud, Storrø and Johnsen92,Reference Allen, Jordan and Storey93) , mainly due to shifting towards Th1 responses(Reference Borchers, Selmi and Meyers35). Although probiotics have more therapeutic effects in allergic diseases than asthma, serum inflammatory factors including IgE, IL-1 and TGF-β are increased in both diseases. Due to the disease’s pathological nature, asthma is more chronic than other lung inflammatory diseases and has multiple pathological causes such as airway fibrosis and polymorphonuclear infiltration(Reference Yawn4). Accordingly, despite improving inflammatory factors and cytokines, probiotics could not significantly improve asthma signs and symptoms. In this regard, a meta-analysis confirmed that serum IgE levels as well as atopic sensitisation are significantly decreased in patients administrated probiotics through a time-dependent manner, but had no effect on asthma(Reference Elazab, Mendy and Gasana59). Although many studies investigated therapeutic applications of probiotics in allergy and asthma patients, due to the chronic nature of COPD and the role of environmental factors in developing COPD, few studies have been done to date evaluating the clinical application of probiotics for COPD patients. In a study administrating orally L. rhamnosus and B. breve (as probiotics), and short-chain galacto-oligosaccharides and long-chain fructo-oligosaccharides (as prebiotics) on mice with emphysema (destruction of the lung parenchyma mostly seen in COPD patients), the reduced infiltration of alveolar macrophages into lungs and a subsequent reduced damage were observed(Reference Verheijden, van Bergenhenegouwen and Garssen94). Given the close relationship between emphysema and COPD in terms of clinical and epidemiological properties, it seems that probiotics could reduce the inflammation and severity of COPD. In addition, there is a potential application of probiotics for COPD management via natural killer (NK) cells. It is known that the function of NK cells is disrupted during COPD progression. Since the daily administration of Lactobacillus as probiotics can increase the activity of NK cells(Reference Borchers, Selmi and Meyers35), probiotics administration in COPD patients can reduce inflammatory responses and symptom severity. Clinical trials that administrated probiotics for chronic respiratory diseases are summarised in Table 2.
Table 1. Clinical trials that administrated probiotics for respiratory infections
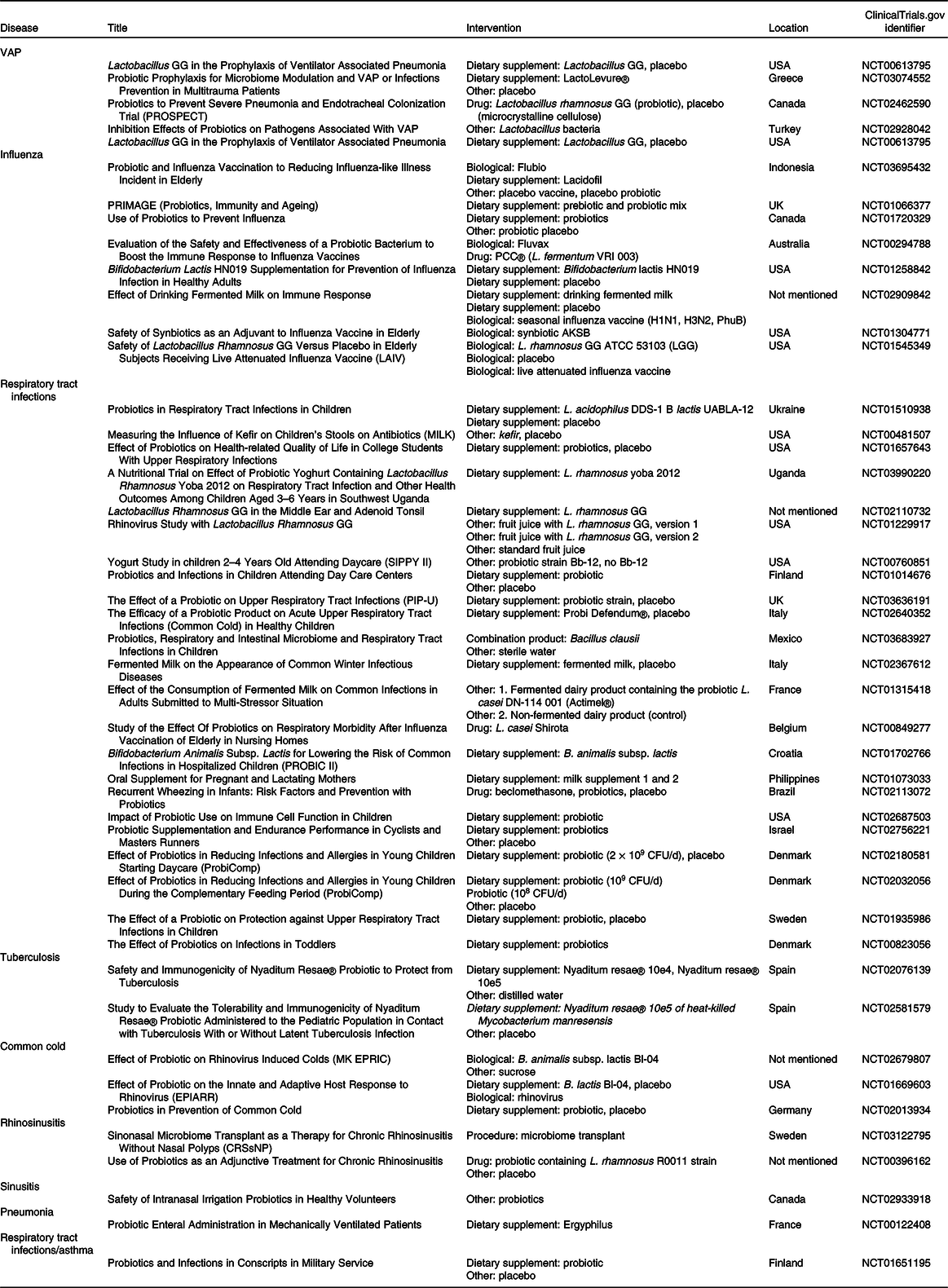
VAP, ventilator-associated pneumonia; CFU, colony-forming units.
Table 2. Clinical trials that administrated probiotics for chronic respiratory diseases
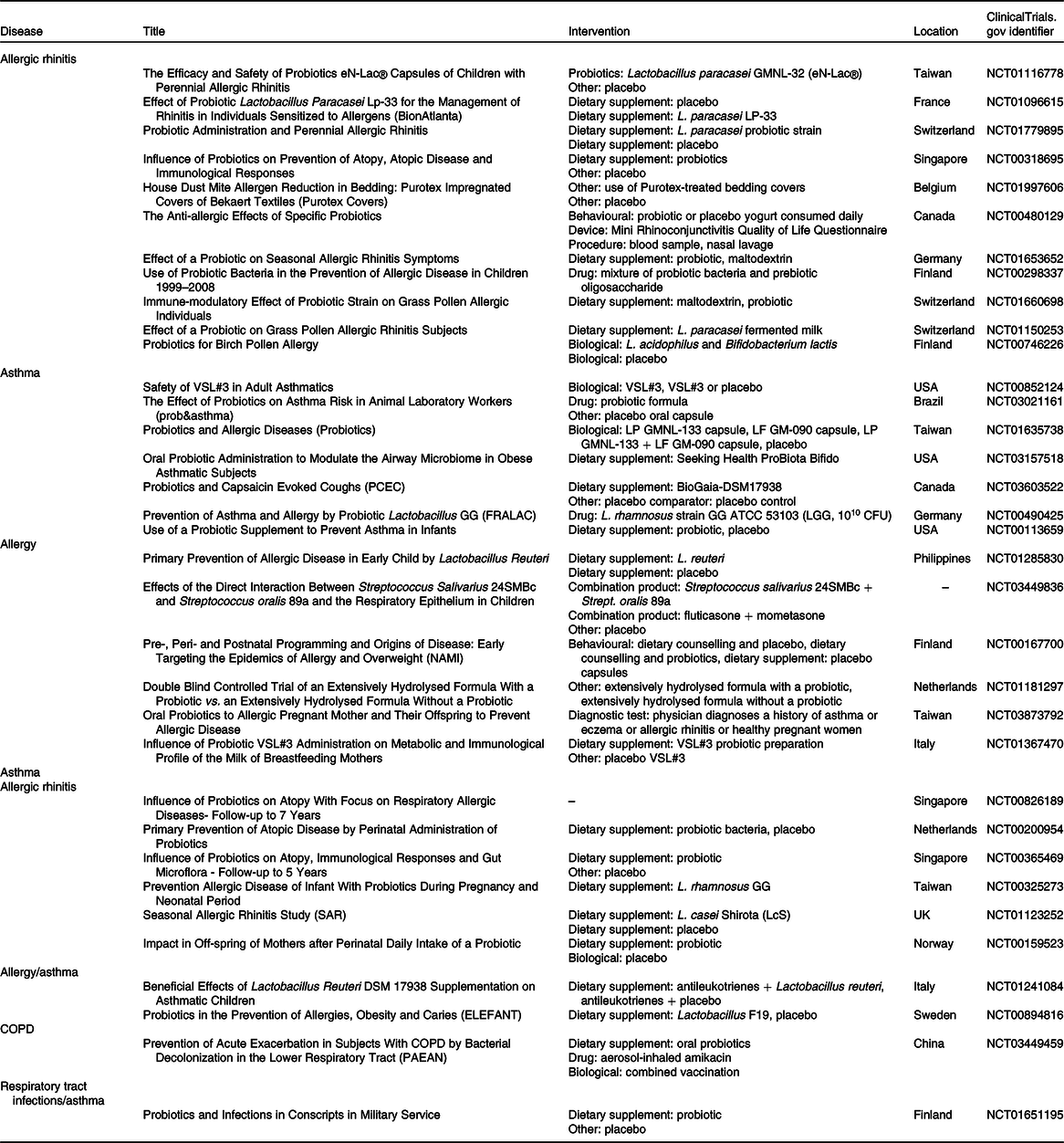
CFU, colony-forming units; COPD, chronic obstructive pulmonary disease.
Overall, studies to evaluate the clinical efficacy of probiotics for the treatment of COPD are limited. Although most of these studies demonstrate a considerable change in the blood level of inflammatory cytokines, indicating some para-clinical efficacies, there are limited benefits of the therapeutic efficacy of probiotics to improve COPD. Since several environmental and personalised factors are involved in the pathophysiology of COPD, the clinical success of probiotic therapy in these patients needs more analytical case–control and cohort assessments. Taken together, the clinical efficacy of probiotic administration for the prevention and amelioration of chronic respiratory diseases can be classified as follows: (1) in allergic rhinitis and eczema: probiotics are effective for the improvement of clinical symptoms, exacerbation periods and inflammatory markers; (2) in asthma: probiotics could only reduce blood inflammatory cytokines, with only a few reports for improving the clinical symptoms; and (3) in COPD: the studies are so limited with no definite conclusion, warranting more investigation.
Nasal administration of probiotics for chronic respiratory diseases
The route of probiotic administration is one of the most important factors affecting its efficacy. Until now, most studies evaluating the effect of probiotics have paid attention to the oral administration route, while the nasal administration route has less been considered. More recently, however, other routes, including the nasal administration route, have been examined in several studies for the administration of probiotics in respiratory diseases with significant results(Reference Hori, Kiyoshima and Shida95). The nasal route makes it possible for the formulated probiotic to arrive directly and affect the lungs. The intranasal administration of probiotics was used first in 2001 for the treatment of influenza disease, due to the possibility to directly access the target organ(Reference Hori, Kiyoshima and Shida95). Some studies have shown that the use of this method in patients with influenza H1N1 reflects significant clinical and para-clinical advantages over the oral route(Reference Pellaton, Nutten and Thierry90,Reference Hori, Kiyoshima and Shida95-Reference Harata, He and Hiruta98) . Since then, several studies have been conducted for the intranasal administration of probiotics mostly against the influenza virus, pneumoviruses (PVM) and syncytial virus infections through animal models and the results have shown an increased level of pro-inflammatory cytokines and a significant reduction of disease severity(Reference Gabryszewski, Bachar and Dyer99-Reference Percopo, Rice and Brenner106). Some studies on nasal probiotic administration for respiratory infections are summarised in Table 3. For example, in a study by Youn et al. (Reference Youn, Lee and Lee104), it was shown that probiotic nasal administration not only decreases viral titration in chicken pulmonary tissues but also diminishes the disease transmission between chickens, suggesting that nasal probiotic therapy is a good approach to increase the efficacy of chicken vaccination against animal transmissible viral diseases. Besides, the studies of Yang et al. (Reference Yang, Yang and Shi102) and Yeo et al. (Reference Yeo, Lee and Kim105) separately introduced the nasal administration route as an effective method to increase immunity against the influenza virus to diminish mortality rates and sequels. These studies claimed that the intranasal route is more efficient than the oral route due to increasing T cell-mediated immunity in the nasal group(Reference Yang, Yang and Shi102,Reference Yeo, Lee and Kim105) . Overall, intranasal studies on H1N1 infections confirmed the effective role of probiotics for the treatment of influenza through stimulating the Th1 response and changing IL-10 and IL-12 cytokines to result in the involvement of T-regulatory cells and balance the immune system. Besides viral infections, nasal probiotics have also been used for bacterial respiratory infections including pneumococcal pneumonia(Reference Zelaya, Laiño and Villena107,Reference Villena, Barbieri and Salva108) , otitis media(Reference Skovbjerg, Roos and Holm109) and chronic sinusitis(Reference Nagalingam, Cope and Lynch110), and promoting immune systems for defence during infections(Reference Youn, Lee and Lee96,Reference Mastrangeli, Corinti and Butteroni111) . Probiotic administration was also effective in allergic reaction responses, as evaluated in some studies. Co-administration of the nasal probiotic L. plantarum with some allergens such as Derp1 and BetV1 was studied and the results showed that immune cells were induced to produce interferon-γ, IL-12 and IL-10 for higher activating Th1 cells, and to decrease significantly eosinophilia in bronchoalveolar lavage (BAL) samples(Reference Hisbergues, Magi and Rigaux112). Also, serum factors that play an important role in allergy formation are more reduced through the nasal administration route. In addition, due to the increase in IgA levels in mucosal surfaces, probiotics can play a preventive role for allergies. These results show that nasal probiotics can be used to reduce the severity of allergic reactions(Reference Hisbergues, Magi and Rigaux112-Reference Hazebrouck, Przybylski-Nicaise and Ah-Leung114). Finally, some studies that compared the efficacy of oral and nasal probiotics have mostly claimed that the nasal route can be clinically more effective than the oral route for respiratory diseases. For example, the study of Pellaton et al. (Reference Pellaton, Nutten and Thierry90) compared the impact of oral and nasal probiotics administration on airway inflammation, showing that although the infiltration of inflammatory cells was decreased in bronchoalveolar lavage (BAL) fluid for both routes, it was statistically significant only in the nasal group with the following changes: a reduction in the number of infiltrating neutrophils, lymphocytes, and eosinophils, a reduction in the level of IL-5 and IgE; a reduction in resealing exotoxins from inflammatory cells; and an increase in the number of nasal cavity T-regulatory cells and FOXP3 (forkhead box P3) cells expressing CD25 and CD5. All these changes can lead to a reduction in the severity of inflammation in the lungs. The increased efficacy of intranasal probiotics in comparison with the oral route can be due to the stronger stimulation of airway immune cells in the nasal cavity and upper respiratory tract. In addition, the direct entrance of these bacteria into the airways could lead to replacement of the pathogenic microbiome (dysbiosis) with the healthy one (symbiosis), which in turn decreases the rate of acute exacerbations. In addition, previous studies have demonstrated that only 20 % of inhaled drugs for asthma are absorbed through the lung tissue and the remaining is absorbed and metabolised systemically resulting in systemic complications(Reference Brutsche, Brutsche and Munawar115). Accordingly, it seems that nasal administration can cause systematic effects by stimulating mucosal lymphoid tissues in the gastrointestinal tract. Also, symbiotic bacteria living in the gastrointestinal tract can prevent the entrance of unknown pathogens to deeper parts by maintaining the integrity of the epithelial wall(Reference Nagalingam, Cope and Lynch110). Such a mechanism could probably apply to inhaled probiotics. Some important studies on nasal probiotic administration for chronic respiratory diseases are summarised in Table 4. In general, the results of intranasal probiotic studies for respiratory infections and also some allergic diseases may suggest that nasal probiotic administration can efficiently help to modulate immune responses and decrease the severity of immune reactions for chronic respiratory diseases including asthma, COPD and allergy, even sometimes more applicable than the oral route. However, how probiotics affect the airway immune system of the lungs and respiratory system, and what is the exact mechanisms of the interaction is still poorly understood and warrants further studies.
Table 3. Studies of nasal probiotic administration for respiratory infections
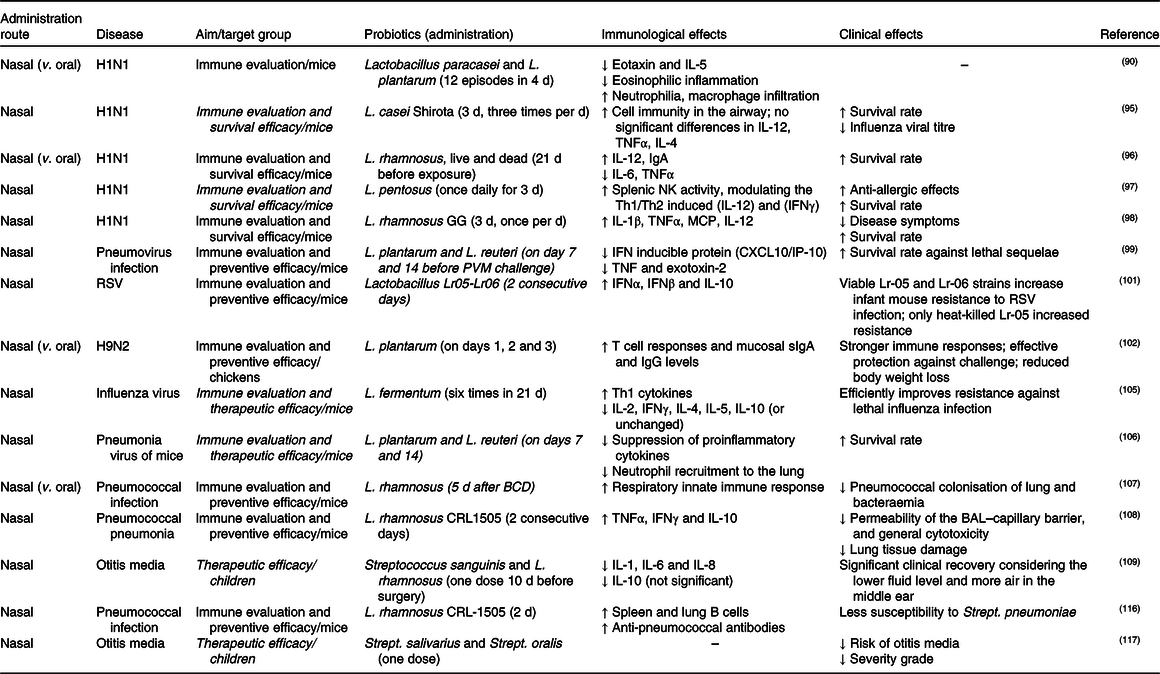
NK, natural killer; Th1, T helper 1; Th2, T helper 2; IFN, interferon; MCP, macrophage chemotactic protein; PVM, pneumoviruses; RSV, respiratory syncytial virus; sIgA, secretory IgA; BCD, balanced conventional diet; BAL, bronchoalveolar lavage.
Table 4. Studies of nasal probiotic administration for chronic respiratory diseases
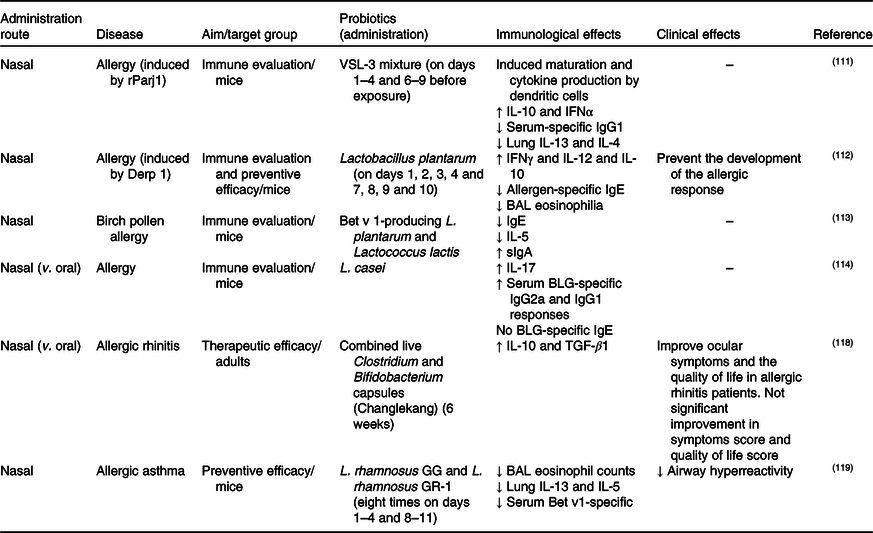
IFN, interferon; BAL, bronchoalveolar lavage; sIgA, secretory IgA; BLG, bovine β-lactoglobulin; TGF-β1, transforming growth factor-β1.
Conclusion
Different studies have various conclusions about the impact of the administration of probiotics in chronic respiratory diseases. Although they have shown the effectiveness of probiotics mostly for the prevention (and in some cases for the treatment) of digestive diseases, there are controversial results for probiotic administration in some chronic respiratory diseases. For allergic diseases, it is found to be effective; for asthmatic patients, it can modulate the serum cytokines and IgE and decrease eosinophilia, but with no significant reduction in clinical symptoms; and for COPD, only limited studies were found with uncertain clinical efficacy. It seems that this efficacy depends on various factors including the type of disease, duration of administration, environmental and personal factors and, most importantly, the route of administration. For intranasal administration, some studies propose more efficacy than the oral route. The direct entrance and contact of a probiotic micro-organism to respiratory epithelial cells might be a reason why the nasal administration is more effective. It is noteworthy that nasal probiotic administration most often was used for acute respiratory diseases/infections (although allergic rhinitis is a chronic inflammatory condition), but it could provide a promising approach for other chronic inflammatory conditions. In general, due to limited studies performed now, it is difficult to determine the impact of nasal administration of probiotics definitively, and it warrants more investigation.
Acknowledgements
The present review was supported by the Research Council of the Baqiyatallah University of Medical Sciences. There was no funding received for the present study. The supporter had no role in the study design, data collection and analysis, decision to publish, or preparation of the manuscript.
The present research received no specific grant from any funding agency, commercial, or not-for-profit sectors.
All authors contributed to the writing and editing of the manuscript.
There are no conflicts of interest.