The oligosaccharide fraction is the third largest constituent of human milk after lactose (Lac) and lipids. Colostrum is reported to contain 20–23 g/l oligosaccharides, and mature human milk is reported to contain 12–15 g/l oligosaccharides(Reference Montreuil and Mullet1–Reference Coppa, Gabrielli and Pierani3). It can be assumed that the oligosaccharide fraction is extremely complex, consisting of at least 1000 different components, with most of them occurring in minor quantities(Reference Stahl, Thurl and Zeng4). To date, more than eighty-five different oligosaccharide structures have been isolated and identified(Reference Kobata, Yamashita and Tachibana5–Reference Boehm, Stahl, Mattila-Sandholm and Scarela8). Most of these carbohydrates possess a Lac unit at the reducing end. Apart from Lac, several other core structures, for example, lacto-N-tetraose (LNT), lacto-N-neotetraose (LNnT), lacto-N-hexaose (LNH), three further hexaoses, several octaoses and even decaoses, have been detected so far. These core structures are built from Lac by the addition of lacto-N-biose units, that is Galβ1-3GlcNAc (type 1 structure), or lactosamine units, Galβ1-4GlcNAc (type 2 structure). Generally, these disaccharide units are either β1,3-linked to one another or to Lac, resulting in elongated linear structures, or β1,6-linked to inner galactose residues, thus building branched oligosaccharides. These core structures are substrates of various fucosyltransferases (FucT) and sialyltransferases in the mammary epithelial cells, and after being modified with fucosyl or sialyl moieties, they probably cannot be elongated or branched anymore(Reference Henry, Oriol and Samuelsson9). Carbohydrates containing sialic acids or sulphate groups are classified as acidic oligosaccharides as opposed to the neutral oligosaccharide fraction comprising core structures as well as fucosylated carbohydrates.
Due to the specific action of several human α-FucT, a variety of fucosylated carbohydrates are biosynthesised in various epithelial cells(Reference Oriol, Le Pendu and Mollicone10, Reference Ma, Simala-Grant and Taylor11). Similar oligosaccharide structures that are closely related to the Lewis blood group system can thus be detected on erythrocytes and in saliva as well as in human milk(Reference Watkins, Greenwell and Yates12). Four different milk groups had been described in accordance with specific Lewis blood group-dependent oligosaccharide patterns(Reference Kobata, Horowitz and Pigman13, Reference Thurl, Henker and Siegel14). In addition to neutral carbohydrates, a variety of acidic oligosaccharides have been found to occur in human milk in relatively low amounts of 1·5–3·3 g/l(Reference Coppa, Pierani and Zampini15). They contain mostly 5-N-acetylneuraminic acid (Neu5Ac), a predominant member of the sialic acid family. α2,3-Sialylation occurs on terminal galactoses of type 1 structures and of Lac. α2,6-Linked Neu5Ac has been detected on terminal galactoses of type 2 structures and of Lac, as well as on subterminal N-acetylglucosamine of type 1 structures(Reference Boehm, Stahl, Mattila-Sandholm and Scarela8, Reference Kobata, Horowitz and Pigman13, Reference Grönberg, Lipniunas and Lundgren16).
Several biological functions of human oligosaccharides, such as prebiotic, anti-adhesive, glycome-modifying and anti-inflammatory effects, have been postulated so far(Reference Donovan17, Reference Bode18). Some evidence exists that the oligosaccharides may protect breast-fed infants from pathogenic bacteria, viruses, toxins, protozoa and fungi by acting as soluble receptor analogues that prevent the interaction of pathogens with epithelial cells at the initial stage of infections(Reference Ruiz-Palacios, Cervantes and Ramos19, Reference Kunz, Rudloff and Baier20). Furthermore, these oligosaccharides may act as prebiotics. N-acetylglucosamine-containing oligosaccharides have been reported to promote the growth of Bifidobacterium bifidum, thereby suppressing the growth of undesirable bacteria(Reference György, Norris and Rose21–Reference Coppa, Zampini and Galeazzi23).
A prerequisite for a profound understanding of the biological role of human milk oligosaccharides is detailed knowledge of their composition and quantities. The first quantitative determinations of oligosaccharides were carried out by Montreuil & Mullet(Reference Montreuil and Mullet1). This examination and the studies done by Viverge et al. (Reference Viverge, Grimmonprez and Cassanas2, Reference Viverge, Grimmonprez and Cassanas24) were performed with a few women using paper chromatography or gel permeation chromatography in combination with colorimetric methods. In the last 15 years, some more detailed studies were performed applying various chromatographic methods for the determination of neutral oligosaccharides during the course of lactation(Reference Coppa, Gabrielli and Pierani3, Reference Coppa, Pierani and Zampini15, Reference Erney, Malone and Skelding25–Reference Newburg, Ruiz-Palacios and Altaye28). To date, only few examinations were carried out in order to quantify acidic oligosaccharides(Reference Coppa, Pierani and Zampini15, Reference Martín-Sosa, Martín and García-Prado29–Reference Bao, Zhu and Newburg31). In the present study, an efficient high-pH anion-exchange chromatographic (HPAEC) method(Reference Erney, Malone and Skelding25, Reference Thurl, Müller-Werner and Sawatzki32) was used to analyse about 175 milk samples from German women during the first 3 months of lactation. This investigation was aimed to broaden the biological knowledge of the composition of milk oligosaccharides, especially with regard to the four milk groups(Reference Thurl, Henker and Siegel14). Furthermore, more detailed facts about these important human milk components will be helpful for the development of better adapted milk formulas.
Subjects and methods
Serological tests
The Lewis blood groups of the women were determined within 3 d postpartum on the day of blood sampling by a haemagglutination tube test. Haemagglutination was examined using corresponding erythrocyte suspensions (3–5 % erythrocytes suspended in 0·9 % NaCl) and monoclonal anti-Lea and anti-Leb antibodies (Immucor, Rödermark, Germany and BAG, Lich, Germany). Incubation was done at room temperature for 15 min. Due to discrepancies between serological tests and chromatographic profiles, some haemagglutination tests were repeated 18–25 months postpartum(Reference Thurl, Henker and Siegel33). The women were not pregnant at that time.
Collection of samples
The present study was conducted according to the guidelines laid down in the Declaration of Helsinki, and all procedures involving human subjects/patients were approved by the ethical committee of the University Hospital of Dresden, Germany. All mothers had given written consent for their participation in the present study. The thirty Caucasian women were living in the region of Dresden; they were between 20 and 35 years old and had given birth to healthy infants who were exclusively breast-fed during the study period. One hundred and seventy-five breast milk samples were taken predominantly on and around seven predetermined days: day 3, 2–5 d postpartum; day 8, d6–d9; day 15, d13–d18; day 22, d20–d26; day 30, d28–d33; day 60, d57–d65; and day 90, d88–d96. In cases where the mothers provided more than one milk sample corresponding to the above-mentioned time intervals, all the samples were analysed for oligosaccharide concentrations, which were expressed as the arithmetic mean values. In a preexamination with six volunteers (Lewis blood type Le(a − b+)) from the region of Frankfurt/Main, Germany, we could not find any significant oligosaccharide variation during two 24 h periods at days 7 and 60 postpartum (data not shown). In order to exclude even small diurnal effects(Reference Viverge, Grimmonprez and Cassanas34), the milk samples were generally collected during the morning hours between 06.00 and 10.00 hours by applying the mid-feed sampling technique, which was shown previously to be an adequate method for carbohydrate analysis(Reference Neville, Keller and Casey35, Reference Thurl, Henker and Taut36). About 5–10 ml aliquots were expressed manually in the middle of feeding into plastic containers. Milk samples were immediately frozen, and stored at − 20°C until analysis.
Chromatographic analysis of oligosaccharides
Sample preparation, including gel permeation chromatographic purification, as well as HPAEC analyses was performed as described previously(Reference Thurl, Müller-Werner and Sawatzki32). Briefly, human milk samples were heated for 30 min at 70°C. One millilitre of human milk was added to 0·1 ml of an aqueous solution containing the internal standards stachyose and galacturonic acid. The samples were subsequently centrifuged and ultrafiltrated (Centrifree, 30 kDa cut-off; Millipore, Schwalbach, Germany). The protein- and lipid-reduced samples were fractionated by gel permeation chromatography using Toyopearl HW 40 (S) columns (1·6 × 80 cm; TosoHaas, Stuttgart, Germany). The carbohydrate fractions were eluted with water (flow rate of 1 ml/min), and monitored by refractive index detection. The major portion of Lac could be removed from the neutral oligosaccharide fraction(Reference Thurl, Müller-Werner and Sawatzki32). Acidic, negatively charged oligosaccharides, eluting near the void volume of the gel permeation column, were clearly separated from the major neutral oligosaccharides determined in the present study. Both neutral and acidic fractions were analysed separately by HPAEC coupled with a pulsed electrochemical detector. Oligosaccharides were identified and quantified using standard substances as well as the internal standards stachyose and galacturonic acid. The calculations done for oligosaccharide concentrations were based on individual molar response factors that had been determined previously(Reference Thurl, Müller-Werner and Sawatzki32). The eluents used for the analysis of neutral oligosaccharides were 0–20 min, 30 mm-NaOH; 20–34 min, 30–100 mm-NaOH; 34–48 min, 100 mm-NaOH/0–28 mm-NaOAc; 48–55 min, 100 mm-NaOH/28–200 mm-NaOAc; and 55–60 min, 100 mm-NaOH/200 mm-NaOAc. In addition, most neutral oligosaccharide fractions were analysed a second time with a different gradient (0–12 min, 60 mm-NaOH; 12–16 min, 60–100 mm-NaOH; 16–30 min, 100 mm-NaOH/0–28 mm-NaOAc; 30–35 min, 100 mm-NaOH/28–200 mm-NaOAc; and 35–40 min, 100 mm-NaOH/200 mm-NaOAc) allowing separation and quantification of the coeluting neutral oligosaccharides 3-fucosyl-lactose (FL) and lacto-N-difucohexaose (LNDFH) I. In contrast to the above-mentioned publication(Reference Thurl, Müller-Werner and Sawatzki32), this additional step was necessary due to altered performances of the CarboPac PA-100 columns (Dionex, Idstein, Germany) used during the study.
The elution conditions for acidic oligosaccharides were 0–8 min, 100 mm-NaOH/20 mm-NaOAc; 8–30 min, 100 mm-NaOH/20–80 mm-NaOAc; 30–55 min, 100 mm-NaOH/80–200 mm-NaOAc; and 55–60 min, 100 mm-NaOH/200 mm-NaOAc. In order to monitor possible artificial hydrolytic degradation of especially susceptible sialyloligosaccharides, free Neu5Ac was quantified along with acidic oligosaccharides. Neu5Ac concentrations were relatively constant (average concentration of 0·019 g/l in milk group 1; data not shown). The amounts of free Neu5Ac were of the same magnitude as reported previously(Reference Wiederschain and Newburg37), and correspond to approximately 2 and 4 % of oligosaccharide-bound Neu5Ac at the beginning of lactation and after 3 months, respectively. Therefore, significant degradation of acidic oligosaccharides due to the action of sialidases or to heat treatment can be excluded(Reference Bertino, Coppa and Giuliani38).
Determination of lactose
Two hundred and fifty microlitres of human milk were mixed with deionised water and with 1 ml Carrez-I-solution (85 mm-K4[Fe(CN)6]) and 1 ml Carrez-II-solution (250 mm-ZnSO4) in a 25 ml volumetric flask. After filtration, Lac concentrations of the diluted (factor 100) and protein- and lipid-reduced milk samples were determined using a Lac/d-galactose test combination obtained from R-Biopharm, Darmstadt, Germany(Reference Beutler and Bergmeyer39). β-Galactosidase released galactose which was subsequently oxidised by galactose dehydrogenase. Lac concentrations could be calculated with the UV absorbances of the reduced cofactor nicotinamide adenine dinucleotide at 340 nm.
Statistical analyses
Data that were analysed by statistical methods consisted either of concentrations of individual oligosaccharides or of sums of various carbohydrates. Apart from total neutral and total acidic oligosaccharides, the following total core structures and fucosylated carbohydrates were summed up, respectively:
total core Lac = Lac+3-FL+2′-FL+LDFT+3′-SL +6′-SL;
total core LNT = LNT+LNFP I+LNFP II+LNDFH I +LNDFH II+LSTa+LSTb+DSLNT;
total core LNnT = LNnT+LNFP III+LSTc;
total core LNH = LNH+2′-F-LNH+3′-F-LNH +2′,3′-DF-LNH;
Fucα1-2Gal = 2′-FL+LDFT+LNFP I+LNDFH I +2′-F-LNH+2′,3′-DF-LNH;
Fucα1-4GlcNAc = LNFP II+LNDFH I+LNDFH II;
Fucα1-3Glc = 3-FL+LDFT+LNDFH II;
Fucα1-3GlcNAc = LNFP III+3′-F-LNH+2′,3′-DF-LNH;
where LDFT, lactodifucotetraose; SL, sialyl-lactose; LNFP, lacto-N-fucopentaose; LST, sialyl-lacto-N-tetraose; DSLNT, disialyl-lacto-N-tetraose; F-LNH, fucosyl-lacto-N-hexaose; DF-LNH, difucosyl-lacto-N-hexaose; Fucα1-2Gal, α1,2-fucose linked to terminal galactose; Fucα1-4GlcNAc, α1,4-fucose linked to subterminal N-acetylglucosamine; Fucα1-3Glc, α1,3-fucose linked to reducing glucose; Fucα1-3GlcNAc, α1,3-fucose linked to subterminal GlcNAc.
The data set is two-factorially organised in the three milk groups and seven lactation times, respectively. Besides, the data set is very unbalanced due to different sample numbers. In milk group 1, 109 samples were assigned into groups according to lactation times, and each group contained between ten and twenty-one samples, whereas in group 2 (twenty-eight samples), the lactation times were represented by three to five samples, and in group 3 (seventeen samples), they were represented by two to three samples. Therefore, several methods were applied for analysing the means of oligosaccharide concentrations. In the case of milk group 1, a one-factorial ANOVA followed by the Student–Newman–Keuls test was used to compare the mean values of lactation times. By comparing the averages of the three milk groups, a two-factorial ANOVA with type III sums of squares was applied, and then least-squares means were calculated. So, the group averages are unbiased and completely comparable together. Differences between averages were tested using the Tukey–Kramer method on a significance level of P = 0·05. In both variance models, the differences between the sample concentrations of the participating women per group and time yielded the experimental error. Generally, oligosaccharide concentrations of milks from women of the same milk group at a given lactation period were highly variable. Due to these large interindividual variations, in some cases, no significant differences between means (P>0·05) were found despite seemingly clear differences.
Trends of oligosaccharide concentrations during lactation were modelled by regression analysis. Simple linear regression as well as polynomial regression of second and third degree was fitted and tested on significant coefficients of regression. The model was accepted if the coefficients of regression were significant (P < 0·05). All analyses of regression were carried out with the individual values, although only the mean values are drawn in Figs. 2 and 3 for clarification purposes. All computations were done using the SAS System (SAS Institute, Cary, NC, USA)(40).
Results
Lewis blood groups and oligosaccharide profiles
According to haemagglutination tests, twenty-two donors (73 %) revealed Lewis blood type Le(a − b+), and five donors (17 %) were determined as having Lewis blood type Le(a+b − ), whereas three women (10 %) were Le(a − b − ) donors. These proportions were in a range that was similar to the frequencies of Lewis blood groups among the European populations(Reference Oriol, Le Pendu and Mollicone10, Reference Viverge, Grimmonprez and Cassanas24, Reference Musumeci, Simpore and D'Agata41).
Applying an HPAEC method, fourteen neutral oligosaccharides listed in Table 1 could be determined (Fig. 1(a)). In accordance with their Lewis blood group Le(a − b+), milk samples of twenty-two donors exhibited all these fourteen structures including α1,2-, α1,4- and α1,3-fucosyloligosaccharides. Milk samples revealing this oligosaccharide pattern were assigned to milk group 1(Reference Kobata, Horowitz and Pigman13, Reference Thurl, Henker and Siegel14). Milk samples of the five non-secretor women (Lewis blood type Le(a+b − )) lacked 2′-FL, LDFT, LNFP I, LNDFH I, 2′-F-LNH and 2′,3′-DF-LNH, and were assigned to milk group 2 because no α1,2-fucosyloligosaccharides were detected (Fig. 1(b) and Table 1). In the milk samples of three mothers with Lewis blood type Le(a − b − ), α1,2- and α1,3-fucosyloligosaccharides were found, whereas LNFP II, LNDFH I and LNDFH II with α1,4-fucose residues were missing (Fig. 1(c). One of these women (B. A.) also lacked LDFT, a fucosylated component typical for this milk group, and 3-F′-LNH, which was normally detected in all milk samples. In spite of these minor deviations, all three mothers were assigned to milk group 3 (Table 1). None of the thirty women in the present study exhibited an oligosaccharide profile without α1,2-linked and α1,4-linked fucosyloligosaccharides, corresponding to milk group 4(Reference Thurl, Henker and Siegel14). This is in agreement with the low prevalence (1 %) of the genotype se/se and le/le among Caucasian women(Reference Oriol, Le Pendu and Mollicone10).
Table 1 Structures of the neutral milk oligosaccharides determined in the present study and their relation to milk groups 1–3

2′-FL, 2′-fucosyl-lactose; 3-FL, 3-fucosyl-lactose; LDFT, lactodifucotetraose; LNT, lacto-N-tetraose; LNnT, lacto-N-neotetraose; LNFP I–III, lacto-N-fucopentaoses I–III; LNDFH I–II, lacto-N-difucohexaoses I–II; LNH, lacto-N-hexaose; 2′-F-LNH, 2′-fucosyl-lacto-N-hexaose; 3′-F-LNH, 3′-fucosyl-lacto-N-hexaose; 2′,3′-DF-LNH, 2′,3′-difucosyl-lacto-N-hexaose; H-2ga, glucose analogue of H-2 antigen(Reference Newburg, Ruiz-Palacios and Altaye28); Lex ga, glucose analogue of Lex antigen; Ley ga, glucose analogue of Ley antigen; ‘+’, the presence of the corresponding oligosaccharide; ‘–’, the absence of the corresponding oligosaccharide.

Fig. 1 High-pH anion-exchange chromatography profiles of neutral oligosaccharide fractions from (a) a Le(a − b+) donor, corresponding to milk group 1, (b) a Le(a+b − ) donor, corresponding to milk group 2, and (c) a Le(a − b − ) donor, corresponding to milk group 3. The peak numbers correspond to the following milk oligosaccharides: 1, 2′-FL; 2, 3-FL; 3, LDFT; 4, LNT; 5, LNnT; 6, LNFP I; 7, LNFP II; 8, LNFP III; 9, LNDFH I; 10, LNDFH II; 11, LNH; 12, 2′-F-LNH; 13, 3′-F-LNH; and 14, 2′,3′-DF-LNH; Lac, lactose; Sta, stachyose; PED, pulsed electrochemical detector.
Carbohydrate fractions
In Table 2, average concentrations of the major carbohydrate fractions are listed. Lac concentrations determined enzymatically were similar in milk samples of all three milk groups. During the study period, concentrations remained constant in mature milk (milk group 1: 57–60 g/l; data not shown), whereas colostral milk at day 3 postpartum contained significantly lower amounts of Lac (average concentration of 50·3 g/l in milk group 1).
Table 2 Average concentrations (g/l) of oligosaccharide fractions

a,b,c Least-squares means (as a consequence of unbalanced data) within a column with unlike superscript letters were significantly different (P < 0·05; the Tukey–Kramer test).
* In some cases, the numbers of samples were slightly reduced.
The sum of the fourteen major neutral oligosaccharides determined by chromatographic analysis approximately represents the neutral oligosaccharide fraction of human milk (Table 1). This carbohydrate fraction is relatively abundant in secretor milks, especially in milk group 3, whereas non-secretors (milk group 2) only produce approximately half the amount (Table 2). In Fig. 2, the variation of neutral oligosaccharides during the course of lactation is shown. The concentration of neutral oligosaccharides in both secretor milks (groups 1 and 3) steadily decreased during the first 90 d postpartum, as indicated by two significant declining straight lines, calculated by regression analysis. In contrast, neutral oligosaccharides in non-secretor milks seemed to remain relatively constant during the study period.
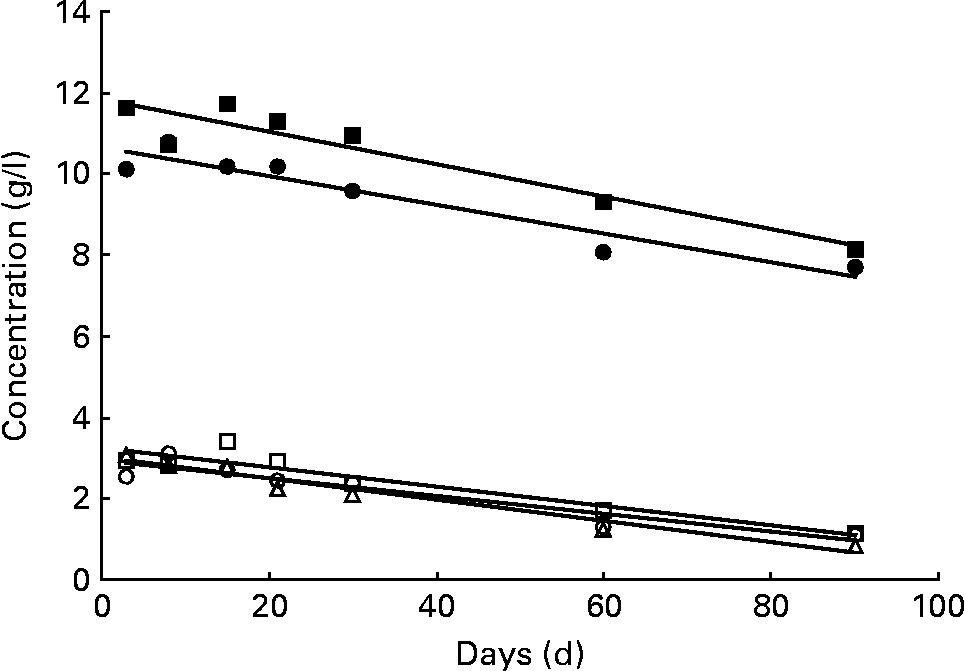
Fig. 2 Variation of neutral and acidic oligosaccharides. Neutral oligosaccharides in milk group 1 (●; c = 10·66 − 0·0355d; P < 0·01) and milk group 3 (■; c = 11·78 − 0·0391d; P < 0·05), and acidic oligosaccharides in milk group 1 (○; c = 2·95 − 0·0220d; P < 0·01), milk group 2 (△; c = 3·05 − 0·0271d; P < 0·01) and milk group 3 (□; c = 3·26 − 0·0236d; P < 0·01). All data symbols represent mean values of the corresponding oligosaccharide concentrations at seven points in time postpartum. However, statistical calculations are based on individual values. The trend line corresponding to the neutral oligosaccharide fraction of milk group 2 was not found to be significant (P>0·05).
In addition to neutral oligosaccharides, six major non-fucosylated acidic oligosaccharides, 3′-SL, 6′-SL, LSTa, LSTb, LSTc and DSLNT, as listed in Table 3, could be determined chromatographically. The sum of these carbohydrates approximately represents the acidic oligosaccharide fraction of human milk. Because of the importance of the Lewis blood group system, these oligosaccharides were also examined separately according to the three milk groups. Milk samples of all three milk types exhibited the above-mentioned six acidic oligosaccharides. The quantities of the acidic oligosaccharide fractions did not differ significantly among the three milk groups (Table 2). In addition, all milk groups exhibited an almost parallel decrease to one-third of the initial acidic sugar concentrations during the study period (Fig. 2).
Table 3 Structures of the acidic milk oligosaccharides determined in the present study

3′-SL, 3′-sialyl-lactose; 6′-SL, 6′-sialyl-lactose; LSTa–c, sialyl-lacto-N-tetraoses a–c; DSLNT, disialyl-lacto-N-tetraose.
Core structures
In Table 4, a comparison of the molar concentrations of Lac, LNT, LNnT and LNH with the amounts of the corresponding total core oligosaccharides, comprising not only the former basic structures but also their fucosylated and sialylated derivatives, is given. The knowledge of the total core structures, representing the real biosynthetic capacity of the milk-producing epithelial cells, is a prerequisite for a profound discussion of the action of various FucT and sialyltransferases. Total core Lac, which can be interpreted as a glucose analogue of type 2 chains(Reference Newburg, Ruiz-Palacios and Altaye28), was by far the most abundant core structure exceeding total core LNT, a type 1 structure, on average by a factor of 34 (group 1 in Table 4). Total core LNnT, a typical type 2 structure, and total core LNH were detected in even lower quantities. Lac was found to be the prevalent form (about 95 %) of the core Lac structure. In contrast, unmodified LNT, LNnT and LNH varied from 17 to 31 % in relation to the corresponding total core structures. Obviously, fucosylated and sialylated oligosaccharides are the predominant forms of these three basic components.
Table 4 Concentrations (mmol/l) of core structures

Lac, lactose; LNT, lacto-N-tetraose; LNnT, lacto-N-neotetraose; LNH, lacto-N-hexaose.
a,b,c Mean values within a column with unlike superscript letters were significantly different (P < 0·05; the Student–Newman–Keuls test for comparisons within group 1).
x,y,z Least-squares means within a column with unlike superscript letters were significantly different (P < 0·05; the Tukey–Kramer test for comparisons between groups).
* In some cases, the numbers of samples were slightly reduced.
LNT was expressed in higher amounts in non-secretor milks (group 2) than in secretor milks (groups 1 and 3), but when comparing total core LNT, it turned out that non-secretor mothers synthesised significantly lower amounts of this core structure (Table 4). Non-secretor women also produced significantly lower concentrations of the total core structures LNnT and LNH, roughly 75 and 55 % of the amounts detected in milk group 1, respectively. In contrast, total core Lac did not differ significantly among the three milk groups. Concentrations of total core LNT, total core LNnT and total core LNH in milk group 1 significantly decreased during the study period. However, colostrum exhibited significantly lower amounts of total core LNT and total core LNH than transitional milk at day 8 postpartum. For the purpose of clarity, time effects are listed in Table 4 only within milk group 1. Milk groups 2 and 3 revealed similar trends during the course of lactation. Due to low sample numbers, these changes were not always judged as significant (data not shown).
Fucosylated neutral oligosaccharides
Average concentrations of eleven fucosylated neutral oligosaccharides that were quantified in the present study are listed in Table 5. The major fucosylated carbohydrates in the samples of milk groups 1 and 3 were 2′-FL and LNFP I, as well as LNDFH I in the milk samples of group 1. Non-secretors (milk group 2) predominantly biosynthesised 3-FL and LNFP II. These carbohydrates, as well as LNDFH II and 3′-F-LNH, were produced at a significantly higher level in non-secretor milks than in secretor milks. During the study period, concentrations of oligosaccharides typical for secretor milks, 2′-FL, LNFP I, 2′-F-LNH and 2′,3′-DFLNH, roughly decreased by half the amount, with the exception of LDFT, which did not alter significantly, and LNDFH I. 3-FL was the only oligosaccharide that steadily increased roughly twofold in all milk groups during the first 3 months of lactation. LNFP III also seemed to increase in secretor milks (groups 1 and 3), although this variation was not statistically significant. The concentrations of LNFP II, LNDFH I, LNDFH II and 3′-F-LNH in milk group 1 samples seemed to increase to maximum levels during the first month postpartum. After 3 months, mature milks contained lower amounts of these oligosaccharides as colostral milks.
Table 5 Concentrations (g/l) of neutral fucosylated oligosaccharides

2′-FL, 2′-fucosyl-lactose; 3-FL, 3-fucosyl-lactose; LDFT, lactodifucotetraose; LNFP I–III, lacto-N-fucopentaoses I–III; LNDFH I–II, lacto-N-difucohexaoses I–II; 2′-F-LNH, 2′-fucosyl-lacto-N-hexaose; 3′-F-LNH, 3′-fucosyl-lacto-N-hexaose; 2′,3′-DF-LNH, 2′,3′-difucosyl-lacto-N-hexaose; ND, not detectable.
a,b,c,d Mean values within a column with unlike superscript letters were significantly different (P < 0·05; the Student–Newman–Keuls test for comparisons within group 1).
x,y,z Least-squares means within a column with unlike superscript letters were significantly different (P < 0·05; the Tukey–Kramer test for comparisons between groups).
* The numbers of samples were slightly reduced.
In Table 6 and Fig. 3, the variation of fucosylated oligosaccharides during the course of lactation with regard to the three milk groups is summarised. Oligosaccharides were summed up on a molar basis according to four different linkage types: α1,2-fucose linked to terminal galactose (sum of 2′-FL, LDFT, LNFP I, LNDFH I, 2′-F-LNH and 2′,3′-DF-LNH), α1,4-fucose linked to subterminal N-acetylglucosamine (sum of LNFP II, LNDFH I and LNDFH II), α1,3-fucose linked to reducing glucose (sum of 3-FL, LDFT and LNDFH II) and α1,3-fucose linked to subterminal GlcNAc (sum of LNFP III, 3′-F-LNH and 2′,3′-DF-LNH.). These molar sum parameters reflect the complete biosynthetic capacity of various FucT during milk production. In addition, the different fucosyl linkage types can possibly be assigned to the action of specific glycosyltransferases. The former α1,2-fucose moieties, typical for secretor milks, were expressed in significantly higher concentration in milk group 3 than in milk group 1. In both milk groups, these concentrations declined during the study period as indicated by significant straight lines of regression in Fig. 3. Fucose moieties with α1,4-bonds, products of the Lewis gene, were equally expressed in groups 1 and 2 (Table 6). At 2 and 3 weeks after birth, their concentrations seemed to reach maximum levels, with colostral concentrations resembling mature milk concentrations after 2 and 3 months postpartum. However, these trends were NS, and are therefore not shown in Fig. 3. Fucose moieties that are α1,3-linked to reducing glucose were detected in all three milk groups, especially in milk samples of group 2 representing the prevalent form of fucose. Increasing amounts of this fucose moiety were found during the study period, proving to be significant in the case of milk groups 1 and 2 (Fig. 3). Equally low levels of α1,3-fucose residues linked to GlcNAc were detected in all milk groups, remaining relatively constant during the first 90 d of lactation.
Table 6 Average concentrations (mmol/l) of fucose moieties

Fucα1-2Gal, α1,2-fucose linked to terminal galactose; Fucα1-4GlcNAc, α1,4-fucose linked to subterminal N-acetylglucosamine; Fucα1-3Glc, α1,3-fucose linked to reducing glucose; Fucα1-3GlcNAc, α1,3-fucose linked to subterminal GlcNAc; ND, not detectable.
a,b,c Least-squares means (as a consequence of unbalanced data) within a column with unlike superscript letters were significantly different (P < 0·05; the Tukey–Kramer test).

Fig. 3 Variation of α1,2-, α1,3- and α1,4-linked fucose moieties. Concentrations of α1,2-linked fucosyl moieties in milk group 1 (○; c = 12·26 − 0·0512d; P < 0·01) and milk group 3 (□; c = 15·89 − 0·0582d; P < 0·01); α1,3-fucosyl moieties linked to reducing glucose in milk group 1 (●; c = 1·36+0·0106d; P < 0·01) and milk group 2 (▲; c = 3·29+0·0253d; P < 0·05); α1,3-fucosyl moieties linked to subterminal GlcNAc in milk group 2 ( × ; c = 0·829 − 0·0031d; P < 0·01). All data symbols represent mean values of the corresponding oligosaccharide concentrations at seven points in time postpartum. However, statistical calculations are based on individual values. No significant trends (P>0·05) were found with α1,4-linked fucosyl moieties and with α1,3-fucosyl moieties linked to reducing glucose in milk group 3, nor with α1,3-fucosyl moieties linked to GlcNAc in milk groups 1 and 3.
Acidic sialylated oligosaccharides
Mean concentrations of the six acidic oligosaccharides determined in the present study are listed in Table 7. 6′-SL was quantitatively by far the most important acidic carbohydrate in all milks. Medium amounts of 3′-SL, LSTc and DSLNT were detected, whereas LSTa and LSTb did not occur at concentration levels above 0·1 g/l. Average concentrations of individual acidic oligosaccharides, though statistically different in some cases, did not vary to a great extent among the three milk groups, thus confirming the results of the whole carbohydrate fraction (Table 2).
Table 7 Concentrations (g/l) of acidic oligosaccharides

3′-SL, 3′-sialyl-lactose; LSTa–c, sialyl-lacto-N-tetraoses a–c; 6′-SL, 6′-sialyl-lactose; DSLNT, disialyl-lacto-N-tetraose.
a,b,c,d Mean values within a column with unlike superscript letters were significantly different (P < 0·05; the Student–Newman–Keuls test for comparisons within group 1).
x,y Least-squares means within a column with unlike superscript letters were significantly different (P < 0·05; the Tukey–Kramer test for comparisons between groups).
Analogously, time effects, as listed in Table 7 with milk group 1, were similar to tendencies observed in the other milk groups (data not shown and Fig. 2). Concentrations of 6′-SL peaked in transitional milk at day 8, but were reduced to at least one-third of the amount 90 d after birth. LSTc, also containing a Neu5Acα2-6Gal linkage, decreased in a similar manner (by a factor of 5 approximately) during the study period. 3′-SL was expressed in mature milk at a relatively constant level after a significant decrease in the first period of lactation. Concentrations of LSTa, a minor acidic sugar bearing a Neu5Acα2-3Gal linkage, decreased after 1 week postpartum, so it could not be detected in several milk samples after 1 month. In contrast, concentrations of LSTb exhibiting a Neu5Acα2-6GlcNAc linkage increased during the first month and remained relatively constant thereafter. DSLNT, the only disialylated carbohydrate analysed and a kind of hybrid structure between LSTa and LSTb, exhibited a maximum time curve.
Discussion
Lac concentrations increased significantly in the case of milk group 1 (Table 4) and milk group 2 (data not shown) during the first 3 weeks of lactation. This result is in concurrence with previous reports(Reference Viverge, Grimmonprez and Cassanas2, Reference Coppa, Gabrielli and Pierani3), although Lac concentrations seemed to be lower in the present study. Since the biosynthesis of nearly all milk oligosaccharides is based on the acceptor Lac(Reference Boehm, Stahl, Mattila-Sandholm and Scarela8, Reference Kobata, Horowitz and Pigman13, Reference Kobata42), the observed decrease of the oligosaccharide concentration during the course of lactation cannot be explained by a shortage of the acceptor molecule. Milk samples of groups 1 and 3 seemed to contain slightly lower Lac levels, although not judged as significant, compared with those of group 2 (Table 2). This possible effect could be counterbalanced by the much higher neutral oligosaccharide concentrations in the former milk groups.
Apart from Lac, six acidic sialylated oligosaccharides, three neutral core structures and eleven neutral fucosylated carbohydrates could be determined during the course of lactation by applying HPAEC. To date, only in the study done by Coppa et al. (Reference Coppa, Pierani and Zampini15), an equally large number of individual carbohydrates were examined, whereas in other studies, approximately ten neutral oligosaccharides(Reference Erney, Malone and Skelding25–Reference Newburg, Ruiz-Palacios and Altaye28) or to roughly ten acidic oligosaccharides(Reference Martín-Sosa, Martín and García-Prado29–Reference Bao, Zhu and Newburg31) were examined. In the present study, we found differences in individual oligosaccharides in relation to lactation periods and, particularly, to the three main milk groups. To date, only few comparisons of several oligosaccharides among two milk groups have been drawn(Reference Viverge, Grimmonprez and Cassanas24, Reference Erney, Malone and Skelding25, Reference Newburg, Ruiz-Palacios and Altaye28).
Fucosyloligosaccharides
The predominant individual oligosaccharides in milk samples of secretor mothers (milk groups 1 and 3) were 2′-FL and LNFP I, consistent with qualitative and quantitative results of earlier reports(Reference Coppa, Pierani and Zampini15, Reference Erney, Malone and Skelding25, Reference Chaturvedi, Warren and Altaye26). The highest concentrations of α1,2-linked fucosyloligosaccharides, especially of 2′-FL, LNFP I and 2,3-DF-LNH (Table 5 and Fig. 3), were found in the samples of milk group 3 (Le(a − b − )), confirming the results reported by Newburg et al. (Reference Newburg, Ruiz-Palacios and Altaye28). It is generally acknowledged that these carbohydrates are synthesised by FucT II, which is encoded by the so-called secretor gene and preferentially accepts type 1 structures(Reference Ma, Simala-Grant and Taylor11, Reference Kumazaki and Yoshida43). LNT, a type 1 structure, represents the precursor of LNFP I. Similarly, Lac, which can be defined as the glucose analogue to type 2 chains (Galβ1-4GlcNAc)(Reference Newburg, Ruiz-Palacios and Altaye28), is the precursor of 2′-FL. Although molar concentrations of total core Lac exceeded those of the acceptor total core LNT by a factor of approximately 34 in milk group 1 (Table 4), the amount of 2′-FL and LDFT, the secretor enzyme products from Lac, surpassed by only 2·3 times the amount of LNFP I and LNDFH I, the corresponding products from LNT. Hence, at least a tenfold preference for type 1 chains can be deduced. In addition, we found that LNH was fucosylated to the same extent as LNT, which means that it was equally accepted by FucT II (data not shown). Ordinary type 2 chains obviously are not at all α1,2-fucosylated at the terminal galactose in milk oligosaccharides, because free carbohydrates containing H-2 antigens, for example, 2′-fucosyl-lacto-N-neotetraose, had not been detected so far(Reference Boehm, Stahl, Mattila-Sandholm and Scarela8). In agreement with previous reports(Reference Coppa, Pierani and Zampini15, Reference Erney, Malone and Skelding25–Reference Sumiyoshi, Urashima and Nakamura27), the concentration of the sum of α1,2-fucosyloligosaccharides decreased significantly within the first 3 months of lactation (Fig. 3). Therefore, one can conclude that the activity of the secretor enzyme (FucT II) is generally reduced during the course of lactation. However, since the acceptor core structures LNT and LNH with the exception of Lac declined in a similar manner during the study period (Table 4), it remains doubtful whether FucT II really became less active on type 1 structures.
The sum of α1,4-fucosyloligosaccharides detected (LNFP II, LNDFH I and LNDFH II) seemed to be slightly higher, although not significantly, in the samples of milk group 2 than in those of milk group 1 (Table 6). These carbohydrates are the main products of the Lewis enzyme (FucT III), which preferentially accepts type 1 chains, resulting in Lea or Leb antigens. We argue that non-secretor women (milk group 2) reveal a higher activity of the Lewis enzyme than the secretor women, because the precursor total core LNT is present in significantly lower amounts in the former (about 80 %, Table 4), whereas the Lewis products tended towards even higher concentrations. The Lewis enzyme was found to be about 100 times more efficient on H-type 1 substrates than on H-type 2 substrates(Reference Costache, Apoil and Cailleau44). Therefore, we concluded that Lex antigens, α1,3-fucose moieties linked to subterminal GlcNAc from type 2 chains, as found in LNFP III, 3′-F-LNH and 2′,3′-DF-LNH, are not or only to a minor extent products of the Lewis enzyme. The finding of similar concentrations of these α1,3-fucosyloligosaccharides in the samples of Le(a − b − ) women (milk group 3 in Table 6), which do not express FucT III, strongly supports this argument. It can be assumed that these oligosaccharides are biosynthesised by a third FucT, for example, the so-called plasma enzyme (FucT VI) that had been detected in human milk(Reference Mollicone, Gibaud and Francois45). Even many other FucT could be involved, since human FucT IV, V, VII, VIII and IX are also known to act as α1,3-FucT(Reference Ma, Simala-Grant and Taylor11).
3-FL, LDFT and LNDFH II, exhibiting fucose moieties α1,3-linked to reducing glucose, were found in the samples of milk group 1 in amounts that were approximately threefold higher than those found in the samples of milk group 3 (Tables 5 and 6). Obviously, women with no active Lewis enzyme produce only minor amounts of these carbohydrates. The Lewis enzyme isolated from human milk as well as a soluble form of the recombinant FucT III have been reported to link fucose also to the 3-O position of the reducing glucose of human milk oligosaccharides in reasonable amounts(Reference Johnson, Donald and Feeney46, Reference Costa, Grabenhorst and Nimtz47). Therefore, we assumed that the major portions of these α1,3-fucosyloligosaccharides are also the products of the Lewis enzyme. A smaller portion should be due to many other FucT. The particularly high amounts of the Fucα1-3Glc motif in milk group 2, which are caused by high quantities of 3-FL and LNDFH II, can only be partially explained with an increased activity of the Lewis enzyme. Knowing that a common enzyme is involved in the biosynthesis of Fucα1-4GlcNAc- and Fucα1-3Glc motifs, similar variations during the course of lactation can be expected. However, α1,4-fucosyloligosaccharides seemed to exhibit maximal values during the first month after birth, whereas oligosaccharides containing the Fucα1-3Glc linkage significantly increased during the study period (Fig. 3).
Sialyloligosaccharides
In contrast to neutral carbohydrates, the six acidic oligosaccharides determined in the present study did not exhibit important differences among the three milk groups. This is not unexpected since these carbohydrates lacked fucose moieties. Several α1,2- and α1,4-fucosylated acidic oligosaccharides that had been detected in human milk(Reference Boehm, Stahl, Mattila-Sandholm and Scarela8, Reference Grönberg, Lipniunas and Lundgren16) should considerably vary among the various milk groups, but such components were not quantified in the present study. Concentrations of 6′-SL, the predominant acidic sugar in the present study, exceeded the amounts reported by other groups by at least twofold, whereas the concentrations of DSLNT were relatively low(Reference Coppa, Pierani and Zampini15, Reference Martín-Sosa, Martín and García-Prado29–Reference Bao, Zhu and Newburg31). The amounts of 3′-SL, LSTa, LSTb and LSTc were in a range that was similar to that of the amounts detected by Asakuma et al. (Reference Asakuma, Akahori and Kimura30), lower than the concentrations reported by Coppa et al. (Reference Coppa, Pierani and Zampini15) and higher than the values found by Bao et al. (Reference Bao, Zhu and Newburg31). Although quantitative results obtained from different studies (including our report) revealed large differences, a significant decrease of the acidic sugar fraction as a whole and of most individual oligosaccharides was generally found during the first few months of lactation.
6′-SL as well as LSTc declined in a similar manner, a fact that supports the hypothesis that a single sialyltransferase, possibly ST6GalI(Reference Legaigneur, Breton and Battari48, Reference Harduin-Lepers, Vallejo-Ruiz and Krzewinski-Recchi49), exclusively accepting type 2 structures, is involved in the biosynthesis of these carbohydrates. The more pronounced decrease of LSTc can be explained by a decrease of total core LNnT, the precursor of LSTc (Table 4), whereas the amounts of total core Lac, precursor of 6′-SL, remained constant after 2 weeks postpartum. 3′-SL decreased to a small extent during the study period, and could be synthesised by ST3Gal IV or also by ST3Gal VI, two α2,3-sialyltransferases preferentially accepting type 2 structures(Reference Harduin-Lepers, Vallejo-Ruiz and Krzewinski-Recchi49). Both we and Coppa et al. (Reference Coppa, Pierani and Zampini15) found that LSTa, a minor acidic sugar, declined very much and could not be or could only partially be detected after 2 and 3 months. We hypothesise that ST3Gal III, an α2,3-sialytransferase acting preferentially on type 1 structures(Reference Harduin-Lepers, Vallejo-Ruiz and Krzewinski-Recchi49), is involved in the biosynthesis of LSTa as well as of DSLNT. LSTb was the only acidic carbohydrate that increased within the first month postpartum, confirming previous results(Reference Coppa, Pierani and Zampini15). The so-called ST6GlcNAc could transfer sialic acid moieties to subterminal GlcNAc(Reference Harduin-Lepers, Vallejo-Ruiz and Krzewinski-Recchi49) yielding LSTb as well as DSLNT, an oligosaccharide exhibiting both α2,6-linked and α2,3-linked Neu5Ac.
Biological implications
Human milk oligosaccharides resist digestion within the stomach and intestine(Reference Donovan17, Reference Engfer, Stahl and Finke50, Reference Gnoth, Kuntz and Kinne-Saffran51), and a significant proportion passes into the lower gastrointestinal tract. Here, the oligosaccharides, especially those compounds containing lacto-N-biose (type 1 structure) or GlcNAc, are thought to promote growth of a favourable gut flora in newborns(Reference György, Norris and Rose21–Reference Coppa, Zampini and Galeazzi23, Reference Wada, Ando and Kiyohara52). The total core structures LNT and LNH, exhibiting lacto-N-biose units and representing the major GlcNAc-containing oligosaccharides in human milk, attain maximum levels after approximately 1 week postpartum but show an almost 50 % decrease by 3 months postpartum. One could therefore conclude that newborns obtain the best protection from human milk oligosaccharides during the first few weeks of lactation, taking into account not only increasing milk volumes(Reference Neville, Keller and Seacat53) but also increasing baby weights during lactation. In addition, we found out that secretor women corresponding to milk groups 1 and 3 significantly produced higher amounts of these core structures than non-secretor women corresponding to milk group 2. It remains to be investigated whether the gut flora of non-secretor mothers differs analogously with a lower occurrence of protective species, such as Bifidobacteria and Lactobacilli.
Several in vitro studies(Reference Ruiz-Palacios, Cervantes and Ramos19, Reference Cravioto, Tello and Villafán54), but also a clinical study(Reference Newburg, Ruiz-Palacios and Altaye28), have suggested the role of fucosyloligosaccharides, particularly α1,2-fucosyloligosaccharides, in reducing infectious diseases, including diarrhoea, which is carried out through inhibition of adherence of pathogens to epidermal surfaces by functioning as soluble competitive inhibitors of the host cell surface ligand and pathogen receptor interaction. Newburg et al. (Reference Newburg, Ruiz-Palacios and Altaye28) reported that babies fed with milk of Le(a − b − ) mothers (milk group 3), exhibiting a high proportion of α1,2-fucosyloligosaccharides, were significantly less infected by stable toxin-Escherichia coli than babies fed with milk of Le(a − b+) mothers (milk group 1). It can be hypothesised that newborns fed with milk of non-secretor mothers (group 2), lacking α1,2-fucosylated oligosaccharides and H-antigens, are even more susceptible to stable toxin-E. coli. In this context, the relatively high frequencies of non-secretors (se/se) in Europe (20 %) compared with the low occurrence of non-secretors, for example, in the mestizo population of Mexico (1 %), have to be discussed(Reference Oriol, Le Pendu and Mollicone10, Reference Erney, Malone and Skelding25, Reference Newburg, Ruiz-Palacios and Altaye28, Reference Musumeci, Simpore and D'Agata41). The high incidence of secretors possibly evolved among the Mexican population also as a result of this pathogenic E. coli species. In other regions with a different evolutionary pressure of pathogens, non-secretors may be better apt to feed babies successfully, resulting in a higher incidence of this Lewis blood group. Apart from the above-mentioned H-antigens, human milk oligosaccharides exhibit a variety of other antigenic determinants, such as Lea, Leb and Lex, depending upon the Lewis blood groups of the mothers as listed in Table 1. Interesting biological functions of these components are not unlikely(Reference Jiang, Huang and Zhong55, Reference Sinclair, Smejkal and Glister56), and remain to be elucidated in more detail.
Human milk oligosaccharides may also influence the infants on a systemic level. Obviously, they are partially absorbed in the intestine of babies, and can be detected in the urine of breast-fed infants(Reference Obermeier, Rudloff and Pohlentz57). Some evidence exists that milk oligosaccharides may function as anti-inflammatory factors, contributing to the lower incidence and severity of inflammatory diseases in breast-fed infants(Reference Donovan17). Particularly, sialic acid-containing oligosaccharides were found to inhibit the formation of platelet–neutrophil complexes and neutrophil activation(Reference Bode, Rudloff and Kunz58). In addition, the acidic oligosaccharide fraction significantly inhibited leucocyte rolling and adhesion on epithelial cells(Reference Bode, Kunz and Muhly-Reinholz59). In this context, the significant decrease of sialylated oligosaccharides during the course of lactation, as reported in the present study, could be biologically relevant.
Acknowledgements
The present research received no specific grant from any funding agency in the public, commercial or not-for-profit sectors. The authors declare no conflicts of interest. We are grateful to Manfred Fels, Danone Research, Friedrichsdorf, Germany, for data processing, and to Christina Hofmann, Fulda University of Applied Sciences, Germany, for performing Lac determinations. We thank Geraldine Groll, Petersberg, Germany, for the language revision. S. T. and J. H. designed the study, and J. H. supervised the clinical part of the study. B. S. supervised the analytical part of the study. B. M.-W. developed the chromatographic methods and analysed the milk samples. M. M. performed the data analyses. S. T. wrote the paper. B. S. and J. J. provided a lot of suggestions with regard to the content and concept of the manuscript. G. B. contributed to the discussion of the results and to the revision of the manuscript.