Introduction
It is becoming increasingly clear that to sustainably supply food to a growing population, improvements only on the production side (through increases in productivity, improved management and use of technology) will not be sufficient (Bajželj et al., Reference Bajželj, Richards, Allwood, Smith, Dennis, Curmi and Gilligan2014). A transition toward less resource-demanding diets in Western societies, that is, diets containing less animal products and more plant-based foods, has been identified as one of the most efficient mitigation options to reduce environmental pressures from the food system (Röös et al., Reference Röös, Bajželj, Smith, Patel, Little and Garnett2017) and to curb demand (Bijl et al., Reference Bijl, Bogaart, Dekker, Stehfest, de Vries and van Vuuren2017). For people who currently have a high intake of red meat, reducing meat consumption would also have clear health benefits (Wolk, Reference Wolk2017).
However, a drastic reduction in animal-based products in current Western diets can introduce new health challenges. White and Hall (Reference White and Hall2017) investigated the radical scenario of removing all livestock from US agriculture and replacing feed production on cropland with increases in food crops proportional to currently grown crops, resulting mainly in an increase in cereals and grain legumes. They found that food production in total would increase by 23%, but that domestic supplies of calcium, fatty acids and vitamins A and B12 would not be sufficient to meet the requirements of the US population. Millward and Garnett (Reference Millward and Garnett2010) also mention the risk of nutrient deficiency in a transition to diets with fewer animal products, highlighting especially zinc, calcium, iodine, vitamin B12 and riboflavin, and also protein supply for the elderly. Hence, when promoting more plant-based foods in diets, these nutritional risks have to be carefully monitored and handled. We suggest that legumes can play a key role in addressing these challenges.
Legumes belong to the plant family Fabaceae, which contains a vast diversity of plants. These include crops grown for seed (e.g., dry beans, dry peas and lentils), fresh vegetables (e.g., green beans and green peas) and livestock forage (e.g., clover and alfalfa). In addition to their high value in human and animal nutrition, legumes provide important agronomic benefits, including symbiotic nitrogen (N) fixation and by serving as break crops in cereal-based cropping systems. The term grain legumes refers to legumes grown for their edible seeds, harvested mature and dried before sale. The term grain legumes is often used synonymously with pulses, but according to the definition used by FAO (FAO, 2017a), pulses exclude grain legumes mainly used for extraction of oil, for example, soybean.
Grain legumes are an important source of protein in developing countries, but in developed countries, animal-source protein now dominates (Joshi and Rao, Reference Joshi and Rao2017). On a global level, grain legumes (excluding soybean) made up 5% of the protein supplied to human diets in 2013 (FAO, 2017b). This is similar to the proportion of protein supplied by fish and seafood (6%), but considerably less than the contribution from cereals (36%) and meat (19%) (Fig. 1). In Sweden, the protein contribution from grain legumes, cereals and meat is 1, 21 and 28%, respectively (FAO, 2017b) (Fig. 1). Consumption of grain legumes is thus exceptionally low in the Swedish diet, while meat (and milk) intake is far above the global average. Meat consumption has also increased continuously for a number of years. Since 1960, per capita meat consumption in Sweden has increased by 73% (SS, 2017a). Current daily per capita consumption of legumes in Sweden is 12 g, but eating patterns differ considerably between individuals and only 50% of Swedish women and 44% of Swedish men include legumes in their diet (NFA, 2012). Hence, despite the health and environmental benefits of grain legumes, the trend globally is now toward more animal protein in all countries in which income is rising (Rivers Cole and McCoskey, Reference Rivers Cole and McCoskey2013). Thus, a revival in grain legumes as an important food crop is needed, as this could provide a solution to several food system challenges.
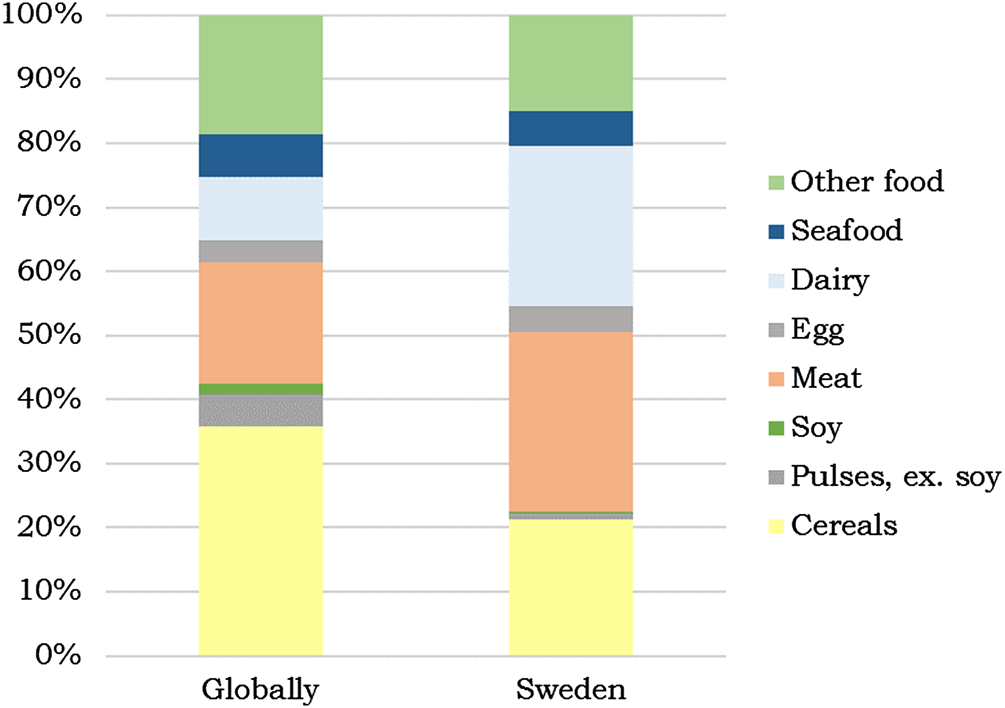
Fig. 1. Contribution of different sources to protein supply globally and in Sweden in 2013 (FAO, 2017b).
The overarching aim of this multidisciplinary paper is to explore the role of grain legumes in the necessary transition to more healthy, resource-efficient and environment-friendly human food systems. We use the case of Sweden and explore, for a range of different aspects, a scenario in which meat consumption is reduced by 50% and replaced by domestically grown grain legumes. We quantify and discuss the implications of such a transition in terms of nutritional intake on population level, for agricultural production and environmental performance. Finally, we discuss challenges in implementing this scenario, focusing on consumer acceptance and production aspects.
The grain legumes considered are faba beans (Vicia faba), yellow peas (Pisum sativum), gray peas (P. sativum var arvense), common beans (Phaseolus vulgaris) and lentils (Lens culinaris). These grain legumes are currently grown to various extents in Sweden, and there are possibilities to enlarge their cultivation if market demand increases. Although we look at Sweden as a specific case, this analysis is also highly relevant for other countries or regions with similar production and consumption patterns.
Background
Health benefits of grain legumes
Grain legumes are a good source of protein, carbohydrates, minerals and vitamins (Table A1). The nutrient content is largely dependent on legume type, species, cultivar and growing conditions. The protein content of legumes is up to threefold higher than in cereals. Moreover, legumes contain high amounts of the amino acid lysine, which is low in cereals, and therefore legume protein favorably complement cereal protein. In contrast to meat and meat products, legumes are also rich in dietary fiber, unsaturated fatty acids and the essential nutrient folate, dietary intake of which is below the recommended level in the Nordic countries. With the exception of soybeans, grain legumes are usually low in fat (≈1%) and free from saturated fatty acids and cholesterol (Table 1). According to the Swedish food composition database (NFA, 2017), one serving of cooked legumes (140–190 g) provides <300 calories and provides a significant amount of daily recommended nutrients: up to 50% of the folate requirement, up to 85% of dietary fiber and up to 15% of protein and potassium.
Table 1. Nutrient content in cooked portions of grain legumes compared with meat products

On the other hand, legumes contain a number of bioactives that are traditionally classified as anti-nutritional compounds, which may reduce the bioavailability of nutrients. However, their adverse effects are currently being re-evaluated, as emerging research has shown that several of these compounds may also have beneficial effects on health (Table A2). In addition, most treatments such as soaking and cooking reduce the content of anti-nutritional compounds (Table A3).
There is strong scientific evidence of the positive effects of legumes on health (Table 2). Legumes have a low glycemic index, attributable to their high content of dietary fiber and resistant starch compared with other starchy foods, which is of interest in prevention of disease, for example, cardiovascular disease and type 2 diabetes (Messina, Reference Messina2014; Clemente and Olias, Reference Clemente and Olias2017).
Table 2. Beneficial health effects of legumes

Summarized from: Clemente and Olias (Reference Clemente and Olias2017), Messina (Reference Messina1999), Messina (Reference Messina2014), Polak et al. (Reference Polak, Phillips and Campbell2015), Rebello et al. (Reference Rebello, Greenway and Finley2014) and Sánchez-Chino et al. (Reference Sánchez-Chino, Jiménez-Martínez, Dávila-Ortiz, Álvarez-González and Madrigal-Bujaidar2015).
Acknowledgement of the health potential of grain legumes is reflected in numerous eating guidelines such as the revised Australian Dietary Guidelines, where tofu and sprouted legumes are listed as health-promoting foods for the first time [reviewed by Kouris-Blazos and Belski (Reference Kouris-Blazos and Belski2016)]. In the recent US Dietary Guidelines (USDA, 2017), legumes are listed as vegetables for a healthy diet. Legumes are also promoted in the Swedish dietary advice (NFA, 2015).
Benefits for cropping systems
Thanks to the ability of legumes to form root nodule symbioses with N2-fixing bacteria, legumes add substantial inputs of N to cropping systems worldwide and reduce the need to produce energy-demanding synthetic N fertilizers (Jensen et al., Reference Jensen, Peoples, Boddey, Gresshoff, Hauggaard-Nielsen, Alves and Morrison2012; Voisin et al., Reference Voisin, Guéguen, Huyghe, Jeuffroy, Magrini, Meynard, Mougel, Pellerin and Pelzer2014). Symbiotic N2 fixation not only provides the legume crop with N, but also supplies the following crop with part of its N requirement. International reviews report considerably higher yields and amounts of residual plant-available soil N for cereals grown after legumes compared with cereal crops (Preissel et al., Reference Preissel, Reckling, Schläfke and Zander2015; Stagnari et al., Reference Stagnari, Maggio, Galieni and Pisante2017). Field experiments and farm surveys have shown that N fertilization rates for cereals after grain legumes can be reduced by 20–35 kg N per hectare (ha) without affecting yield levels, compared with cereals after cereals (Preissel et al., Reference Preissel, Reckling, Schläfke and Zander2015; SBA, 2016). Based on field trials performed in Sweden, the Swedish Board of Agriculture (SBA, 2016) estimates a yield increase corresponding to 0.5–1 metric tonne (hereafter ton) per ha in cereals grown after grain legumes compared with cereals following cereals. This expected yield increase can be compared with the results from a meta-analysis of European cropping systems by Preissel et al. (Reference Preissel, Reckling, Schläfke and Zander2015), who found large variations in the yield advantage of grain legume pre-crops depending on fertilizer strategy and reference pre-crop. Average yield increase of grain legume pre-crops compared with cereal pre-crops was 2.2 tons ha−1 in unfertilized cereal crops and 0.7–1.5 tons ha−1 in moderately and highly fertilized cereal crops in that European meta-analysis (Preissel et al., Reference Preissel, Reckling, Schläfke and Zander2015).
Legumes also serve as break crops, exploiting the benefits of a diversified crop rotation in cereal-dominated crop production systems. The break crop benefits include reduced problems with crop diseases and weeds (Kirkegaard et al., Reference Kirkegaard, Christen, Krupinsky and Layzell2008; Stagnari et al., Reference Stagnari, Maggio, Galieni and Pisante2017; Watson et al., Reference Watson, Reckling, Preissel, Bachinger, Bergkvist, Kuhlman, Lindström, Nemecek, Topp, Vanhatalo, Zander, Murphy-Bokern and Stoddard2017), improved soil structure/increased content of soil organic matter (West and Post, Reference West and Post2002; Hernanz et al., Reference Hernanz, Sanchez-Giron and Navarrete2009; Preissel et al., Reference Preissel, Reckling, Schläfke and Zander2015) and increased availablity of other plant nutrients such as phosphorus (Shen et al., Reference Shen, Yuan, Zhang, Li, Bai, Chen, Zhang and Zhang2011). According to Ebert (Reference Ebert2014), the current overwhelming dominance of a few major crops (wheat, rice, maize) in global agriculture poses a high risk of crop failure. In this context, diversification of cropping systems by increased legume cultivation could increase the resilience to stress caused by variable weather conditions or weeds, insect pests and diseases (Ebert, Reference Ebert2014). Another advantage of integrating legumes into cropping systems is that they enrich the landscape with flower resources that are often scarce in cereal-dominated cropping systems, thereby enhancing the diversity and abundance of bumblebees and other insects that can provide ecosystem services, for example, pollination, for other crops (Köpke and Nemecek, Reference Köpke and Nemecek2010). Further benefits of legumes include potential for enhanced soil C sequestration, building soil fertility (Jensen et al., Reference Jensen, Peoples, Boddey, Gresshoff, Hauggaard-Nielsen, Alves and Morrison2012).
Considering their environmental benefits and potential for enhanced economic profitability through savings on inputs (reduced need for fertilizers and pesticides) and yield increases in subsequent cereal crops, grain legumes are strongly underused crops in European agriculture. Currently, <2% of the agricultural land in Europe is used for the cultivation of grain legumes (Watson et al., Reference Watson, Reckling, Preissel, Bachinger, Bergkvist, Kuhlman, Lindström, Nemecek, Topp, Vanhatalo, Zander, Murphy-Bokern and Stoddard2017). Due to the risk of increasing problems with soil-borne diseases, pathogens and pests, grain legumes cannot be grown too frequently on the same land (Watson et al., Reference Watson, Reckling, Preissel, Bachinger, Bergkvist, Kuhlman, Lindström, Nemecek, Topp, Vanhatalo, Zander, Murphy-Bokern and Stoddard2017). However, among the diversity of grain legume species and varieties, it should be possible to identify grain legume crops that can be grown in most European pedo-climatic regions. Thus, even with careful restrictions regarding the frequency of grain legumes in crop rotations, there is room for considerable increases in the area of grain legume cultivation in Europe.
Benefits for the environmental impact of food systems
Legumes, most importantly soybeans, are a feed ingredient in many livestock diets. However, when human-edible biomass is fed to animals, this entails an unavoidable loss of calories and nutrients available to humans. Mottet et al. (Reference Mottet, de Haan, Falcucci, Tempio, Opio and Gerber2017) found that, on a global scale, 2.8 kg of human-edible feed is used to produce 1 kg of ruminant meat, while for monogastric species (pigs and poultry) the corresponding value is 3.2 kg. Hence, with few exceptions, animal-based foods show larger negative environmental impacts than plant-based foods (Di Paola et al., Reference Di Paola, Rulli and Santini2017) (Fig. 2).

Fig. 2. Climate impact of legumes and pulses per kg protein relative to animal-based protein sources. Based on data from Clune et al. (Reference Clune, Crossin and Verghese2017).
A review of life cycle assessment (LCA) studies on food has shown that the cradle-to-farm gate climate impact of dried and fresh legumes, that is, the aggregated and weighted emissions of different greenhouse gases (GHG) arising on the farm and from the production of inputs, varies between 0.15 and 2.46 kg of carbon dioxide equivalents (CO2e) per kg of legume (Clune et al., Reference Clune, Crossin and Verghese2017). The major sources of on-farm GHG emissions from legume cultivation are nitrous oxide (N2O) emissions from soils and carbon dioxide (CO2) emissions from fossil energy use in field machinery and irrigation equipment. As production and use of N mineral fertilizer is associated with considerable GHG emissions, legumes can contribute to mitigation of climate change by allowing mineral fertilizer to be replaced with N2 fixation (Jensen et al., Reference Jensen, Peoples, Boddey, Gresshoff, Hauggaard-Nielsen, Alves and Morrison2012). Existing LCA studies on specific legumes do not fully consider the beneficial effects that legumes bring to other crops and the crop rotation by the pre-crop effects which reduce the need for mineral fertilizers and pesticides (‘Benefits for cropping systems’ section). Therefore, the benefits of legumes compared with other protein sources are probably underestimated in such studies.
The nitrogen footprint, the total direct N losses to the environment that occur for the production of 1 kg of food, is also higher for animal-based than plant-based foods, due to N losses from manure management and the need for greater cropland areas for feed production. Leip et al. (Reference Leip, Weiss, Lesschen and Westhoek2013) modeled the N footprint of different foods in the European Union (EU) and found that legumes had about half the N footprint of milk, 10% of that of pork and only 2% of that of beef, but still had a higher N footprint than fruit, vegetables and potatoes. However, legumes had the lowest ‘nitrogen investment factor’ (quantity of new reactive N required to produce one unit of N in the product) of all foods, only 1–2 kg of N per kg of N in legumes compared with 15–20 kg N per kg of N in beef. As for emissions of ammonia, these are considerable from livestock production due to emissions from manure management (Röös et al., Reference Röös, Sundberg, Tidåker, Strid and Hansson2013).
Another important environmental impact category related to food production is pesticide use, leading to toxic effects on ecosystems and humans. Data availability on these aspects is very limited, but Nordborg et al. (Reference Nordborg, Davis, Cederberg and Woodhouse2017) found that the potential freshwater ecotoxicity impact of bread, milk, minced beef, chicken fillet and minced pork was approximately 2, 3, 50, 140 and 170 times that of pea soup, respectively. Moreover, in comparison with Brazilian soybean, all Swedish crops scored significantly better, due to more rigorous legislation, agronomic practices with comparatively more diverse crop rotations and climate conditions preventing certain disease and pest problems. This illustrates that pesticide use, and hence the ecotoxicity impact, of growing legumes is highly variable and highly dependent on the type of cropping system and that there are good possibilities to implement practices for grain legume production with low overall ecotoxic impact.
In addition, the ecotoxic impact from increasing cultivation of grain legumes is affected by the use of pesticides not only in the actual crop itself, but also in following crops. Several studies report lower ecotoxicity impacts when grain legumes are included in cereal-dominated crop rotations (Nemecek et al., Reference Nemecek, von Richthofen, Dubois, Casta, Charles and Pahl2008; MacWilliam et al., Reference MacWilliam, Wismer and Kulshreshtha2014). Increasing crop diversity by avoiding grain legume monocultures or heavily legume-dominated cropping systems, and promoting non-chemical measures to control weeds, pests and diseases, will thus be key for reducing ecotoxicity impacts and reaping the other benefits from introducing grain legumes into cereal-dominated cropping systems. In this perspective, organic agriculture provides one example of a method for cultivating grain legumes with low or no chemical inputs, especially since the yield difference between organically and conventionally grown grain legumes is relatively small (de Ponti et al., Reference de Ponti, Rijk and van Ittersum2012).
Material and methods
Description of the meat reduction scenario
To analyze the prospects and challenges of reducing meat consumption in favor of legumes, we selected the case of Sweden and an explorative scenario in which meat consumption is reduced by 50%. There were four reasons for this level of reduction. First, it would keep consumption by high-meat consumers well below the level recommended by the World Cancer Research Fund (max. 500 g of cooked red meat per week) to reduce the risk of some cancer forms, which is also the maximum red meat consumption level stated in dietary advice from the Swedish National Food Agency (NFA, 2015). Secondly, it is the level of reduction advocated by WWF Sweden for several environmental reasonsFootnote 1. Thirdly, it would reduce Swedish meat consumption to approximately the global average (from 84 to 42 kg carcass weight per capita per year; FAO, 2017b), in line with an international contraction and convergence strategy in which the current global meat consumption is distributed equally across global citizens as suggested by McMichael et al. (Reference McMichael, Powles, Butler and Uauy2007). Fourth, although even deeper cuts in meat consumption would probably be needed to reach global environmental targets (Bajželj et al., Reference Bajželj, Richards, Allwood, Smith, Dennis, Curmi and Gilligan2014), a 50% reduction as a first step would be in line with some emissions pathways to limit global warming to 2°C as stipulated by the Paris agreement (Hedenus et al., Reference Hedenus, Wirsenius and Johansson2014).
In our scenario, we assumed an equal reduction in meat across the main livestock species and across different animal parts (muscles and offal). We took data on meat consumption from the latest national food consumption survey Riksmaten (NFA, 2012), which reports an average daily per capita intake of meat products of 110 g, divided among different products as follows: red meat 63 g, chicken 22 g, sausage 21 g, offal 3 g and blood 1 g (NFA, 2012). Hence, a 50% reduction means a reduction in meat products of 55 g. In our scenario, this meat is replaced by 55 g of cooked grain legumes daily, corresponding to approximately 20 g dried legumes. After accounting for total postharvest losses of 11% (FAO 2011), the amount of grain legumes required for the transition scenario corresponds to an annual total of 75,000 tons for the Swedish population (10 million people). Replacement based on edible weight rather than energy or protein content is justified because the energy and protein content in the Swedish diet on a population level is well within the recommended range (NFA, 2012), so it is not necessary on a population level to replace all energy and protein provided by meat. Moreover, we assumed that, when shopping for foods, consumers base their purchase decisions on the amount of food, rather than the energy and protein content.
Assumptions on grain legume cultivation
Crop production in Sweden, as in many other developed countries, is characterized by a high degree of specialization. The plain areas of central Sweden are dominated by cereal production, while perennial grass, often in combination with clover, for dairy and beef production is mainly found in less favorable agricultural areas with mixed farmland and woodland (SS, 2017c). In 2016, perennial grass or grass/clover was the most frequently cultivated crop, grown on 40% of the total cropland in Sweden, followed by wheat (17%) and barley (13%). Approximately 70% of Swedish cropland is currently used for feed production (SS, 2017c).
Grain legumes are only grown on 2.2% of the total cropland area in Sweden (SS, 2017c) and production is dominated by two crops: faba bean (104,000 tons from 30,000 ha) and yellow (dry) pea (93,000 tons from 25,000 ha) (SS, 2017b). The majority of Swedish faba bean and yellow pea production is currently used as animal feed. Sweden also produces approximately 1000 tons common bean, mainly the brown bean variety which was grown on about 600 ha in 2016 (SS, 2017b). Since common bean is frost sensitive and requires a long growing season and dry weather during autumn, production has so far been restricted to suitable soils in south-east Sweden, mainly the island of Öland on the Swedish east coast (Fogelberg, Reference Fogelberg2008). Due to consumer demand, there is increasing interest within the food industry in extending Swedish common bean production, which has increased in area lately (expanding to land previously not used for common bean, e.g., on the island of GotlandFootnote 2) and diversity (adding, e.g., black, borlotti, kidney and white bean varieties) in recent yearsFootnote 3. Until recently, fresh pea was grown on 9000–10,000 ha in Sweden, but this dropped to 2500 ha in 2017 (SS, 2017b) as a consequence of changes in contract production by the main Swedish fresh pea-producing company.
Based on the above, the additional legume consumption in the transition scenario was assumed to consist of 40% each of faba beans and yellow peas, 10% gray peas, 8% common beans and 2% lentils. This mixture was chosen to provide variation in the types of legumes consumed, while taking into account the feasibility of increasing Swedish cultivation of different legume species and varieties. For example, it would have been beneficial for nutrient intake to further increase the proportion of common beans and lentils, but the Swedish cultivation area for these crops is restricted by climate and infrastructure (on-farm machinery, experience/skills in cultivation techniques, transportation to cleaning and processing facilities). Further increase in the production of common beans and lentils can be considered feasible in the long term.
We assumed that the legumes needed for replacing the meat are domestically grown. We based this assumption on political and consumer interest in more regional foods and more local food systems. In addition, the Swedish Food Strategy (GOS, 2016) ratified in 2016, aims at increasing Swedish food production, another reason why considering domestic supply is relevant. For the same reason, we assumed that the reduction in meat consumption comes primarily from a decrease in imported meat. Approximately 50% of beef consumed is currently imported to Sweden (SBA, 2017c), so our assumed 50% reduction in beef does not affect domestic beef meat production. For pork and chicken, approximately 30% is imported (SBA, 2017a, 2017b), so the scenario involves a decrease in Swedish pork and chicken production of 40,000 tons bone-free pork and 25,000 tons bone-free chicken (29%).
To produce 1 kg of bone-free pork in Sweden, 5.6 kg of cereals are used, while to produce 1 kg of bone-free chicken, 2.5 kg of cereals are used (Cederberg et al., Reference Cederberg, Sonesson, Henriksson, Sund and Davis2009). As most cereals used for feed in Sweden are domestically grown, a 29% reduction in domestic pork and chicken production would ‘free up’ 288,000 tons of cereals, corresponding to approximately 48,000 ha of cropland. The reduction in domestic meat production would also mean a reduction in the use of domestically grown rapeseed, faba bean and pea as feed for pigs and poultry. According to Cederberg et al. (Reference Cederberg, Sonesson, Henriksson, Sund and Davis2009), 0.06 kg of domestically grown rapeseed and 0.08 kg faba beans and peas are used for producing 1 kg of bone-free pork, while the corresponding figures for producing 1 kg of bone-free chicken are 0.03 kg of domestically grown rapeseed and 0.13 kg faba beans and peas. Hence, in total, 3600 tons of rapeseed are ‘freed up’ when domestic pork and chicken production is reduced in the transition scenario, which corresponds to approximately 1000 ha. Finally, in total, 8400 tons of legumes are ‘freed up’ when domestic pork and chicken production is reduced. This amount was subtracted from the total requirement for faba beans and peas in the calculations for the transition scenario. Even though quality requirements are often higher when crops are used as food ingredients rather than when used as animal feed, this difference was assumed to be negligible in this context. The other feedstuffs used for pigs and poultry are either imported or are by-products from the food industry. Hence, its decreased used in the scenario does not affect Swedish agriculture.
Calculation of nutrient intake
In the current Swedish diet, meat and meat products are the main source of protein, zinc, vitamin B12 and iron, providing about 20–30% of the total daily intake of these nutrients. An important question is therefore how well the proposed scenario of reducing meat consumption by 50% and replacing it with legumes based on mass, complies with current Nordic Nutrition Recommendations (NNR) (Norden, 2014) on population level.
To investigate this, we compared the current intake of nutrients, based on data from the recent Swedish dietary survey (NFA, 2012) (not accounting for under- or overestimation of consumption of certain foods), to outcomes from the transition. For simulating the scenario after transition, the average nutrient contribution from meat and meat products was cut by 50% and replaced by the nutrient contribution of a daily portion of 55 g cooked grain legumes (see ‘Description of the meat reduction scenario’ section) using data from the Swedish food composition database (NFA, 2017). The recommended daily intake was based on the reference adult (average for men and women aged 30–64 yr, with body weight 70 kg, a sedentary lifestyle, and a low physical activity level of 1.4). We also looked at the folate and total iron relative to NNR specifically for women of reproductive age, as recommendations for women (400 µg for childbearing age and 500 µg during pregnancy and lactation) are much higher than the recommended level (300 µg) for the general population.
Calculation of environmental impacts
We estimated how the climate impact and land use of the average Swedish diet would change in our transition scenario by halving the GHG emissions and land use from meat reported by Röös et al. (Reference Röös, Karlsson, Witthöft and Sundberg2015) and adding emissions and land use from production and preparation of the additional grain legumes. Röös et al. (Reference Röös, Karlsson, Witthöft and Sundberg2015) assessed the climate impact and land use from the current Swedish diet to be 1.9 tons CO2e and 0.34 ha per capita per year, respectively. This was done by multiplying values on food consumption from the recent Swedish dietary survey (NFA, 2012) by LCA values on climate impact and land use for foods on the Swedish market. Regarding post-farm processing of the grain legumes, we accounted for emissions caused by cooking yellow peas based on Röös et al. (Reference Röös, Karlsson, Witthöft and Sundberg2015), which are of the same magnitude as emissions from preparation and freezing of processed pea burgers according to Davis et al. (Reference Davis, Sonesson, Baumgartner and Nemecek2010) (approximately 0.25 kg CO2e per kg of grain legumes, compared with emissions caused by growing the legumes of 0.7 kg CO2e per kg of legumes).
Based on current average mineral N fertilization rates of 103 kg N per ha to cereals and 143 kg N to oilseed rape (SS, 2017d), we calculated the reduced need for mineral N fertilizer in the transition scenario as a consequence of reduced use of cereals and rapeseed for animal feed. We also estimated the effect on domestic ammonia emissions, using emission factors for different animal products from Vallin et al. (Reference Vallin, Grimvall, Sundblad and Djodjic2016).
If the increased grain legume cultivation is based on mixed cropping systems, reducing the need for pesticides compared with monocropping, it is likely that water pollution in the form of pesticide residues and excess nutrients from fields will also be reduced in the scenario. However, the exact extent of this reduction is difficult to establish due to the uncertainty in terms of what will happen with the ‘surplus’ cropland, and therefore a quantitative estimate of total water pollution was not included in this study. However, we calculated the change in N load to recipient waters from Swedish wastewater treatment systems due to the lower N content in the diet in the transition scenario. We based this assessment on figures from Vallin et al. (Reference Vallin, Grimvall, Sundblad and Djodjic2016) on the average N removal efficiency of municipal sewage systems and on-site systems and the number of people connected to these systems.
Results and discussion
Impacts on nutrient intake
As shown in Figure 3, after the proposed transition, the estimated average daily intake for energy, fat, protein, vitamin B12, zinc and total iron is still within the recommended range, even before adding the grain legumes to the diet. (No data are shown for the other vitamins and minerals that are above recommendations in both scenarios.) However, calculations for iron based on the average requirements for men and post-menopausal women mask the fact that, for women of childbearing age and during pregnancy, the diet does not meet the dietary recommendations either before or after the transition (Fig. 3). Irrespective of the dietary regime chosen, women of childbearing age are recommended to consume more total iron and especially the more bioavailable heme iron. The same applies to pregnant women, who are recommended by NNR to take a supplement of 40 mg as a general dose or 60 mg as an individual prophylactic to ensure normal iron status. The situation is similar for selenium, for which average intake is lower than recommended in the current diet due to low selenium content in Swedish soils, and intake is further decreased in the scenario diet.

Fig. 3. Intake (%) of key nutrients in the current Swedish diet (CD) and in the scenario diet (SD) in which meat is reduced by 50% and replaced with legumes, relative to the Nordic Nutrition Recommendations (NNR) (Norden, 2014). The recommended daily intake is based on the reference adult (average for men and women aged 30–64 yr, with body weight 70 kg, a sedentary lifestyle and a low physical activity level of 1.4), shown as the range (boxes) for energy, fiber and macronutrients. The black line represents the recommended average daily micronutrient intake for men and women (for folate and iron the recommendation for the reference adult is given, please note that this is below the recommendation for women of reproductive age) (Norden, 2014). The insert shows intake of folate and total iron relative to NNR for women of reproductive age.
The estimated energy intake remains almost unchanged after the transition and the intake of carbohydrates is slightly increased (Fig. 3). While there is a trend for a reduction (~10%) in total fat intake (Fig. A1), the nutritional quality is improved by the higher content of health-beneficial unsaturated fatty acids.
The most beneficial aspect increasing the legume consumption is the increased intake of fiber and folate. The current average fiber intake in the Swedish population is far below the recommended level (Fig. 3), amounting to 20 g day−1. In the transition scenario, the fiber intake increases by 25% through incorporation of grain legumes (Fig. A1), to 25 g day−1, which is at the lower limit of the recommendation. Average folate intake is also improved after the transition, due to the incorporation of grain legumes. This is of particular importance for women of childbearing age and during pregnancy and lactation.
To summarize, dietary changes according to the scenario with reduced meat and increased legume consumption – provided consumers maintain a varied diet – would not negatively affect nutrient intake on population level. However, for some individuals, especially those with a low intake of meat, those with specific nutritional requirements or pregnant women, reduced meat consumption is not recommended without introducing measures to ensure that intake of critical micronutrients is met by careful selection of specific foods in the diet or by supplementation, as only voluntary fortification is practiced in Sweden. It should also be borne in mind that the choice of meat and meat products can have an impact on the nutritional quality; for example, it is recommended to reduce intake of processed meat products rather than fresh meat.
Impact on and challenges for agricultural production
The transition scenario would require about 26,500 ha for the increased Swedish cultivation of grain legumes (Table 3), which is approximately 1% of Swedish arable land. Since the amount of land that would be made available due to the reduction in domestic chicken and pork production (reduced need for cereals and rapeseed in animal feed) exceeds this value, there is enough agricultural land in Sweden to enable the transition. Compared with the current land use, there would be a net surplus of about 21,500 ha that could be used for other purposes, such as cultivation of bioenergy crops or food crops for export, or for nature conservation.
Table 3. Assumed yield levels and required area for each of the legume varieties assumed in the transition scenario

Yields of faba bean, yellow pea and common bean correspond to national averages for these crops during the period 2000–2016 (calculated from annual reports from Statistics Sweden). Yields of gray pea and lentil are estimates based on unpublished results from field experiments in Skåne, southern Sweden, as statistics for these crops are missing.
a For faba bean and yellow pea, the amount of 8400 t that would be freed up by reduced need for animal feed was subtracted from the total amount needed (4200 t each) before calculating the land area requirement.
However, even though more than enough cropland is made available in the transition scenario by reduced cereal and rapeseed cultivation (less animal feed), expanding grain legume cultivation to the required level might be challenging, at least for some crops. The area of increased faba bean and yellow pea cultivation in the transition scenario (8900 ha each) corresponds to approximately 33% of the current cultivation of these two crops. Assuming that the current faba bean and pea production is maintained after the transition (i.e., in addition to the amounts required for the transition), the total cultivation of these crops would then amount to approximately 73,000 ha. This is still only about half the area that could potentially be used for these two grain legumes in Sweden, according to an analysis of the potential for domestic production of protein crops (Gustafsson et al., Reference Gustafsson, Bergsten, Bertilsson, Kronqvist, Lindmark Månsson, Lovang, Lovang and Swensson2013).
On the other hand, it might be more difficult to increase the production of gray pea, common bean and lentil to meet the amounts required in the transition scenario. Gray pea and lentil can be cultivated and harvested with standard machinery on farms that produce cereals, and these two crops are well suited for the climate and common soil types in southern and central Sweden. However, both crops are prone to lodging, and weed management is a major challenge in lentil cultivation (Döring, Reference Döring and De Ron2015). Intercropping with a cereal crop is known to reduce both the risk of lodging and the abundance of weeds (Hauggaard-Nielsen et al., Reference Hauggaard-Nielsen, Jørnsgaard, Kinane and Jensen2008; Döring, Reference Döring and De Ron2015), but large-scale application of intercropping will require multi-actor collaborations to generate knowledge about cultivation techniques and facilitate sorting of the mixed crops (Bedoussac et al., Reference Bedoussac, Journet, Hauggaard-Nielsen, Naudin, Corre-Hellou, Jensen, Prieur and Justes2015). Scaling up Swedish gray pea and lentil cultivation from the currently very low level is therefore associated with important challenges, at least in the short term.
Concerning common bean, its share in the transition scenario would require production to be more than four times larger than today. This increase would require expansion outside the regions where common bean is currently produced in Sweden, and since efficient harvesting of common bean requires special combine harvesters (Fogelberg, Reference Fogelberg2008), this expansion involves challenges such as investment in machinery and acquisition of knowledge for cultivation of a new crop. The suitable cultivation area for common bean is restricted to south-east Sweden, due to the crop's sensitivity to frost and the need for dry weather during harvest. The amount of common beans required in the transition scenario might therefore be close to the maximum potential production of the crop within Sweden.
The transition scenario could have included additional grain legumes, for example, narrow-leaved lupin (Lupinus angustifolius) and soybean (Glycine max). Lupin could be of particular interest, since it can potentially be grown on soils that are less suitable for common bean, faba bean or pea. Due to their limited current use in Swedish agriculture (lupin and soybean) and the lack of available data on nutritional content (lupin), these crops were not included in the calculations for the transition scenario. However, this choice does not exclude the possibility that lupin and soybean can play important roles for future Swedish cultivation and use of grain legumes.
Assuming that current Swedish grain legume cultivation remains unchanged, the additional 26,500 ha in the transition scenario would increase the total grain legume area to 3.2% of Swedish arable land, compared with the current level of 2.2%. While this is an important increase in relative terms (45%), 3.2% is a small proportion of total cropland and there is still potential for further increasing grain legume cultivation for both feed and food, allowing more farmers to exploit the benefits of grain legumes in their cropping systems.
On a general level, challenges that need to be overcome to reach the production levels required in the scenario include: legume yield variability (lack of adapted varieties, difficulties in controlling weeds, pests and diseases), low awareness about rotational benefits (yield improvements and cost savings in subsequent crops) and tradition (generally low interest in grain legumes among researchers, advisors, plant breeders, seed producers and traders) (Zander et al., Reference Zander, Amjath-Babu, Preissel, Reckling, Bues, Schläfke, Kuhlman, Bachinger, Uthes, Stoddard, Murphy-Bokern and Watson2016; Watson et al., Reference Watson, Reckling, Preissel, Bachinger, Bergkvist, Kuhlman, Lindström, Nemecek, Topp, Vanhatalo, Zander, Murphy-Bokern and Stoddard2017). For example, increased investments in grain legume breeding to develop varieties that mature earlier or are more cold-tolerant would make it possible to expand grain legume production into areas with no or low current production, for example, in northern Sweden. Enhanced cropping systems research and advisory capacity could also be promoted to improve the generation and exchange of knowledge for optimizing the benefits of grain legumes in crop rotations (Magrini et al., Reference Magrini, Anton, Cholez, Corre-Hellou, Duc, Jeuffroy, Meynard, Pelzer, Voisin and Walrand2016; Zander et al., Reference Zander, Amjath-Babu, Preissel, Reckling, Bues, Schläfke, Kuhlman, Bachinger, Uthes, Stoddard, Murphy-Bokern and Watson2016). Regarding yield variability, weed control can partly be achieved by mechanical means and the integration of weed-suppressive cover crops, complementing and reducing the use of herbicides. Furthermore, intercropping grain legumes with cereals is known to significantly reduce weed problems, and has also been shown to reduce certain diseases and pests (Hauggaard-Nielsen et al., Reference Hauggaard-Nielsen, Jørnsgaard, Kinane and Jensen2008).
Research shows that increasing farmers’ awareness of the benefits of legume growing will not be sufficient to reach the goal of agriculture system transition to achieve wider benefits. To increase the adoption rate, aspects of profitability and other non-profit-related factors also need to be addressed (Kuehne et al., Reference Kuehne, Llewellyn, Pannell, Wilkinson, Dolling, Ouzman and Ewing2017). Environmental and risk aspects are important. Support from agricultural development agencies is needed to foster adoption of more legume-based farming practices and to compensate for losses when animal production is reduced. However, policies to support this transition will have to consider the complex interactions between agricultural systems, market demand and the climate impact from farming, processing and consumption (Wigboldus et al., Reference Wigboldus, Klerkx, Leeuwis, Schut, Muilerman and Jochemsen2016).
Impact on the environment
The per capita climate impact and land use related to food consumption would be reduced by 20 and 23%, to 1.5 tons CO2e and 0.26 ha, respectively, by a 50% reduction in meat consumption and addition of 55 g cooked grain legumes per day (Fig. 4a and b). A 20% reduction in the climate impact of food consumption is a considerable decrease. An additional 20–30% is likely to be achievable through improvements on the production side. Bryngelsson et al. (Reference Bryngelsson, Wirsenius, Hedenus and Sonesson2016) quantified this potential under ‘moderate’ and ‘optimistic’ assumptions for Swedish food consumption, including strategies such as low-emitting manure handling, the use of renewable energy and increased livestock efficiencies. They found the mitigation potential from such improvements to be between −31% (‘moderate’ assumptions) and −57% (‘optimistic’ assumptions) of the emissions caused by the current Swedish diet. The Swedish Board of Agriculture presents a more modest estimate, that the GHG emissions from Swedish agriculture could be reduced by approximately 20% through improvements in management and new technologies (SBA, 2012). Together with the decrease from halving meat consumption investigated in this study, this could reduce the climate impact of the Swedish food system by approximately half or potentially more. This can be compared with the Swedish targets for reductions of GHG, which state that emissions should be reduced by 63% by 2030 and by 75% by 2040 (compared with 1990) from sectors not covered by the EU Emissions Trading System, including agriculture (The Swedish Parliament, 2017).

Fig. 4. Climate impact (a) and land use (b) of the current Swedish diet in a scenario in which meat consumption reduced by 50% and replaced by legumes.
In the scenario, approximately 5100 tons less mineral N would be needed in Swedish agriculture due to reduced need for cereals and oilseed crops for animal feed. Furthermore, if 25 kg less N fertilizer were applied per ha to non-legume crops grown after a grain legume (compared with after a non-legume pre-crop; Preissel et al., Reference Preissel, Reckling, Schläfke and Zander2015), an additional 700 tons of mineral N fertilizer could be saved as a result of the increased grain legume cultivation in the transition scenario. This potential reduction (5800 tons) corresponds to 3.5% of the total amount of mineral N fertilizer used in Swedish agriculture in 2016 (SS, 2017d).
Storage and spreading of manure give rise to acidifying emissions through ammonia volatilization. However, since the majority of all ammonia emissions from Swedish livestock production are associated with dairy and beef production (Vallin et al., Reference Vallin, Grimvall, Sundblad and Djodjic2016), which are unaffected in the transition scenario (as domestic beef production is unaffected and only imports are reduced), ammonia volatilization within Sweden is only marginally affected (reduced by approximately 3%).
As the diet after transition contains less protein (6%) and hence less N, excretion of N will be lower, which in turn will affect wastewater composition. The N load to recipient waters from Swedish wastewater treatment systems is reduced by almost 1200 tons N per year in the transition scenario. Thus, dietary changes will affect eutrophying emissions through two pathways: land use change when less arable land is required for food production and lower N loads from sewage systems when total protein intake is reduced (Vallin et al., Reference Vallin, Grimvall, Sundblad and Djodjic2016). In addition, the reduction in chicken and pig production means that less manure is produced, which also reduces the risk of eutrophication.
Challenges related to consumption: how to get more legume-based products on the market?
There are many other challenges than those related to primary production (‘Impact on and challenges for agricultural production’ section) which need to be overcome in implementing the scenario explored here. Increasing demand from consumers and the food industry is probably the first and most important driver to overcome current limitations in the cultivation and use of grain legumes in the Swedish food system. New market opportunities for legume-based foods and increased consumer awareness about the environmental and health benefits of legumes are reported to be important factors for increasing the price paid to farmers (Zander et al., Reference Zander, Amjath-Babu, Preissel, Reckling, Bues, Schläfke, Kuhlman, Bachinger, Uthes, Stoddard, Murphy-Bokern and Watson2016). This mechanism would help to expand the cultivation of grain legumes, provided that an increase in consumer demand is met by domestic production.
In terms of consumer demand, there are positive developments. The Nielsen global consumer report for 2015 shows that food attributes such as ‘fresh, natural and minimally processed’ are increasingly important.Footnote 4 There is also a growing trend for vegetarian and vegan diets in many countries in the Global North. At the same time, there is a growing trend for ready-to-eat, fast-cooking, convenience food. In a scenario where meat consumption is reduced by 50% and replaced with legumes, the legumes will therefore also have to be incorporated into convenience foods, as it is unlikely that most consumers will alter their food habits to consume substantially more unprocessed plain legumes. In the scenario explored here, 80% of legume consumption is assumed to be based on the traditional animal feed crops faba bean and pea. A successful transition will thus require product development where these crops need to be processed into attractive food products that Swedish consumers accept as meat substitutes.
However, one potential obstacle for consumers as regards these novel convenience foods is higher price compared with conventional products (De Marchi et al., Reference De Marchi, Caputo, Nayga and Banterle2016). While grain legumes in raw form are typically a low-cost alternative, in processed form and as an ingredient in convenience foods legume prices are currently higher.
One key component of changing consumption and purchase patterns is knowledge regarding the environmental and health benefits of legume-based products (Lemken et al., Reference Lemken, Knigge, Meyerding and Spiller2017). A recent study on consumer preferences in Finland found that, although beans and soy-based plant proteins are infrequently consumed in Finland, there is potential for using beans as a meat substitute in the ‘meat-eating culture’ of Finland (Jallinoja et al., Reference Jallinoja, Niva and Latvala2016). However, the proportion of consumers who plan to increase their bean consumption in the future is relatively low (20%). Consumers aged 25–34 are generally more inclined to eat beans, as are consumers living in cities and those with a higher education level. Knowledge about the benefits of legume food and about how to prepare tasty bean-based meals is important for increasing consumption of legumes.
A central challenge for the true potential of the scenario to materialize is that increased intake of legumes is not enough; meat consumption must also decrease substantially. Stoll-Kleemann and Schmidt (Reference Stoll-Kleemann and Schmidt2017) list 11 influential factors behind the high level of meat consumption in developed countries. These include values and attitudes, but also social norms, roles and relationships. In addition, the ‘food environment’ or other personal, social and external factors may be important in explaining the existing meat eating culture. Besides subjective and social norms, price and availability are important in changing consumption patterns. If broader consumer groups, rather than small groups of health-conscious or vegetarian consumers, were to recognize that meatless foods can be linked to personal health, animal welfare and sustainability issues, then consumer habits and perceptions might gradually change and a new awareness and new social norms could be created. However, as this is a slow process, public policy options have also been suggested, including a tax on meat and dairy (Säll and Gren, Reference Säll and Gren2015). Apostolidis and McLeay (Reference Apostolidis and McLeay2016) highlight the need to target interventions and policies for reduced meat consumption at specific consumer segments, rather than at the average consumer, as preferences for meat substitutes vary greatly between consumer groups.
A limiting factor at the food industry level is access to local facilities for intermediate processing or pre-treatment of legume grains to be included as functional ingredients, such as a flour or protein isolate. In this interface between raw material supply and processing into novel products, co-evolutionary mechanisms in the agri-food sector can be an additional reason why it is difficult to increase the use of grain legumes. Interdependencies among actors in the dominant cereal-based systems for production and processing are suggested to cause lock-in effects that hinder the development of alternative (legume-based) systems (Magrini et al., Reference Magrini, Anton, Cholez, Corre-Hellou, Duc, Jeuffroy, Meynard, Pelzer, Voisin and Walrand2016). Unlocking the system will therefore require, among other actions, investment in processing/pre-treatment facilities for other crops than the currently dominating commodities.
Conclusions
Reducing Swedish meat consumption by 50% and replacing it with a daily per capita consumption of 55 g domestically grown cooked grain legumes would bring many benefits. It would reduce the climate impact (−20%) and land use (−23%) associated with the Swedish diet, reduce the need for N fertilizer and the N load from wastewater plants, greatly increase fiber intake and improve folate intake from diets, and bring many agronomic benefits to Swedish cropping systems. However, achieving a large increase in the production of domestic grain legumes to supply the necessary legumes involves several challenges, such as lack of suitable varieties and difficulties controlling weeds, pests and diseases. The challenges are likely to be particularly pronounced for grain legume crops that are not yet well known to Swedish farmers, including lentils, gray peas and common beans. Nevertheless, the transition is technically feasible from an agronomic perspective based on available land and considering how grain legumes can be included in current crop rotations. Other challenges are lack of processing facilities to supply functional legume-based ingredients to food industries and low consumer awareness about the benefits of grain legumes. A successful transition according to our scenario requires the development of new attractive products acceptable as meat substitutes, made out of legumes that have traditionally been used as feed crops (pea and faba beans). Hence, a successful transition to more sustainable plant-based food systems would require concerted actions by many food system actors, including continued research on legume cultivation and processing and a diverse set of policy actions to reduce meat consumption and increase domestic grain legume production, processing and consumption.
Acknowledgements
Our thanks to the Swedish research council FORMAS for funding the project.
Annex

Fig. A1. Relative contribution (%) of nutrients from meat products (gray bars) plus substitute portion of grain legumes (green bars) after transition compared with current habitual meat intake before transition.
Table A1. Nutrient composition of raw dried grain legumes (per 100 g)

Table A2. Potentially positive and negative effects of anti-nutritional compounds on health

Table A3. Effects of food processing of legumes on content of nutrients and anti-nutritional compounds
