The ecology of broad spectrum diets has long been an issue in the prehistory of arid western North America. Broad spectrum diets have been described as energetically expensive, incurring high caloric costs in harvesting, processing, and preparing food materials for human consumption (Flannery Reference Flannery, Ucko and Dimbleby1969). Such diets included seed, insect, and small mammal components that required different technologies and social structures to provide sufficient caloric yields. This “broad spectrum revolution” of subsistence modification occurs around 9000 cal BP in the Great Basin and Colorado Plateau and is associated with the intensive incorporation of small seed processing, basketry, and grinding stone technologies (Geib and Jolie Reference Geib, Jolie and Vierra2018; Rhode et al. Reference Rhode, Madsen and Jones2006; Simms Reference Simms2008).
The hypothesis that has been most often proposed to explain this dietary pattern is drawn from optimal foraging theory, which suggests that people in general only resorted to low-return small seeds after calorically higher-return resources and high-quality resource patches diminished in abundance (O'Connell et al. Reference O'Connell, Jones, Simms, Madsen and O'Connell1982). Based on the principles of the diet breadth (or prey choice) model (Stephens and Krebs Reference Stephens and Krebs1986), food resources are ranked based on their expected post-encounter energetic return rates (kcal/hr) relative to other resources in the diet. Small seeds and other expensive plant resources are typically ranked low compared to large-bodied animals because of their high handling costs relative to energetic yields (Table 1). The diet breadth model predicts that foragers will always pursue higher-ranked resources on encounter and take lower-ranked resources only when encounter rates with higher-ranked resources decline (Schoener Reference Schoener1971). An increase in the abundance of lower-ranked resources in the diet therefore indicates a decline in the availability of high-ranked resources and an overall reduction in foraging efficiency (Bayham Reference Bayham1979; Broughton and Grayson Reference Broughton and Grayson1993; Kelly Reference Kelly1995a; Winterhalder and Smith Reference Winterhalder, Smith, Smith and Winterhalder1992).
Table 1. Post-encounter Return Rates and Relative Rank of Dietary Resources at North Creek Shelter.

Note: Return-rate values come from the published literature (e.g., Broughton et al. Reference Broughton, Cannon, Bayham and Byers2011; Couture et al. Reference Couture, Ricks and Housley1986; Diehl and Waters Reference Diehl, Waters, Kennett and Winterhalder2006; Gremillion Reference Gremillion2004; Kelly Reference Kelly1995a; Rhode and Rhode Reference Rhode and Rhode2015; Simms Reference Simms1987; Smith and Martin Reference Smith and Martin2001; Smith and McNees Reference Smith and McNees2005; Ugan and Rosenthal Reference Ugan and Rosenthal2016).
This hypothesis, however, assumes an “average” or “undifferentiated” forager. It does not take into account differentiated and specialized roles in foraging strategies, such as age or gender, nor does it account for other hunting technologies (Hawkes and O'Connell Reference Hawkes and O'Connell1992:64; Jochim Reference Jochim1988:130; Kelly Reference Kelly, Cummings, Jordan and Zvelebil2014; Sassaman Reference Sassaman1992; Wood et al. Reference Wood, Brian, Harris, Raichlen, Pontzer, Sayre, Sancilio and Berbesque2021). These assumptions are important because the costs and benefits associated with reproductive fitness can differ between men and women (O'Connell et al. Reference O'Connell, Hawkes and Blurton-Jones1999). A more nuanced hypothesis (Elston and Zeanah Reference Elston and Zeanah2002) suggests gender-specific foraging patterns across the Great Basin and Colorado Plateau during the early and middle Holocene. Whereas women foraged in local and reliable resource patches, accumulating low-return resources and investing heavily in plant-processing tools, men pursued fluctuating but highly mobile, large-bodied animals at some distance. It has also been suggested that variability in acquiring large game would lead to women foraging for lower-ranked resources (Elston et al. Reference Elston, Zeanah and Codding2014).
Increasing aridity and vegetation responses have been offered as the ecosystem drivers of global changes in dietary patterns (e.g., Edwards and O'Connell Reference Edwards and O'Connell1995; O'Connell and Hawkes Reference O'Connell, Hawkes, Winterhalder and Smith1981, Reference O'Connell and Hawkes1984; O'Connell et al. Reference O'Connell, Jones, Simms, Madsen and O'Connell1982; Rhode et al. Reference Rhode, Madsen and Jones2006). In the Great Basin and Colorado Plateau specifically, dietary changes have been attributed to intense aridification that began at about 9500 cal BP (e.g., Grayson Reference Grayson2000, Reference Grayson2011; Louderback et al. Reference Louderback, Kiahtipes and Janetski2020; O'Connell et al. Reference O'Connell, Jones, Simms, Madsen and O'Connell1982; Schmitt and Lupo Reference Schmitt and Lupo2012; Simms Reference Simms2008). However, this paradigm of increasing aridity and dietary response has never been tested quantitatively using multiple lines of evidence from a single site. The current study applies the diet breadth model to understand human dietary patterns through time using the North Creek Shelter (NCS) faunal, botanical, stone tool, and climate datasets.
Although there are several types of measurable parameters used to examine diet breadth and foraging efficiency (e.g., evenness/richness, skeletal representation, age/sex ratio of faunal remains, climate, technology/tools, etc.), this study relies on abundance indexes constructed over time for faunal and botanical resources at NCS. These indexes, along with one for stone tools, are used to test the assumption that increasing aridity during the early to middle Holocene caused the decline of high-return resources and, therefore, led to the incorporation of low-return resources into the diets of the NCS occupants. To determine if diet breadth changes and/or a shift to low-return resources are coincident with climate change, an increase in leporids, small seeds, and ground stone tools should be positively correlated with warmer/drier climates. If the patterns do not conform, then alternative hypotheses must be examined.
Site Setting and Stratigraphy
NCS lies at the base (1,900 m asl) of a south-facing sandstone cliff (Straight Cliffs Formation) overlooking Escalante Valley in southern Utah (Figure 1). The stratigraphic sequence is more than 4 m deep and contains many archaeological features—such as hearths and pits—as well as abundant stone tools, faunal assemblages, and botanical remains (Figure 2). The finds were recovered from cultural strata deposited between 11,300 cal BP and 300 cal BP, and they are defined as Paleoarchaic (Strata II, III, and IV; 11,300–10,200 cal BP), Early Archaic (Stratum V; 10,200–8000 cal BP), Mixed Archaic (Stratum VI; 8000–6700 cal BP), Fremont (Stratum VII; 1200–300 cal BP), and Late Prehistoric (Stratum VIII; Figure 2). The strata were further subdivided into 68 substrata (Va, Vb, Vc, etc.) based on observable changes in the sediments, with some substrata interpreted as short-term living/use surfaces (see Janetski et al. Reference Janetski, Bodily, Newbold and Yoder2012).
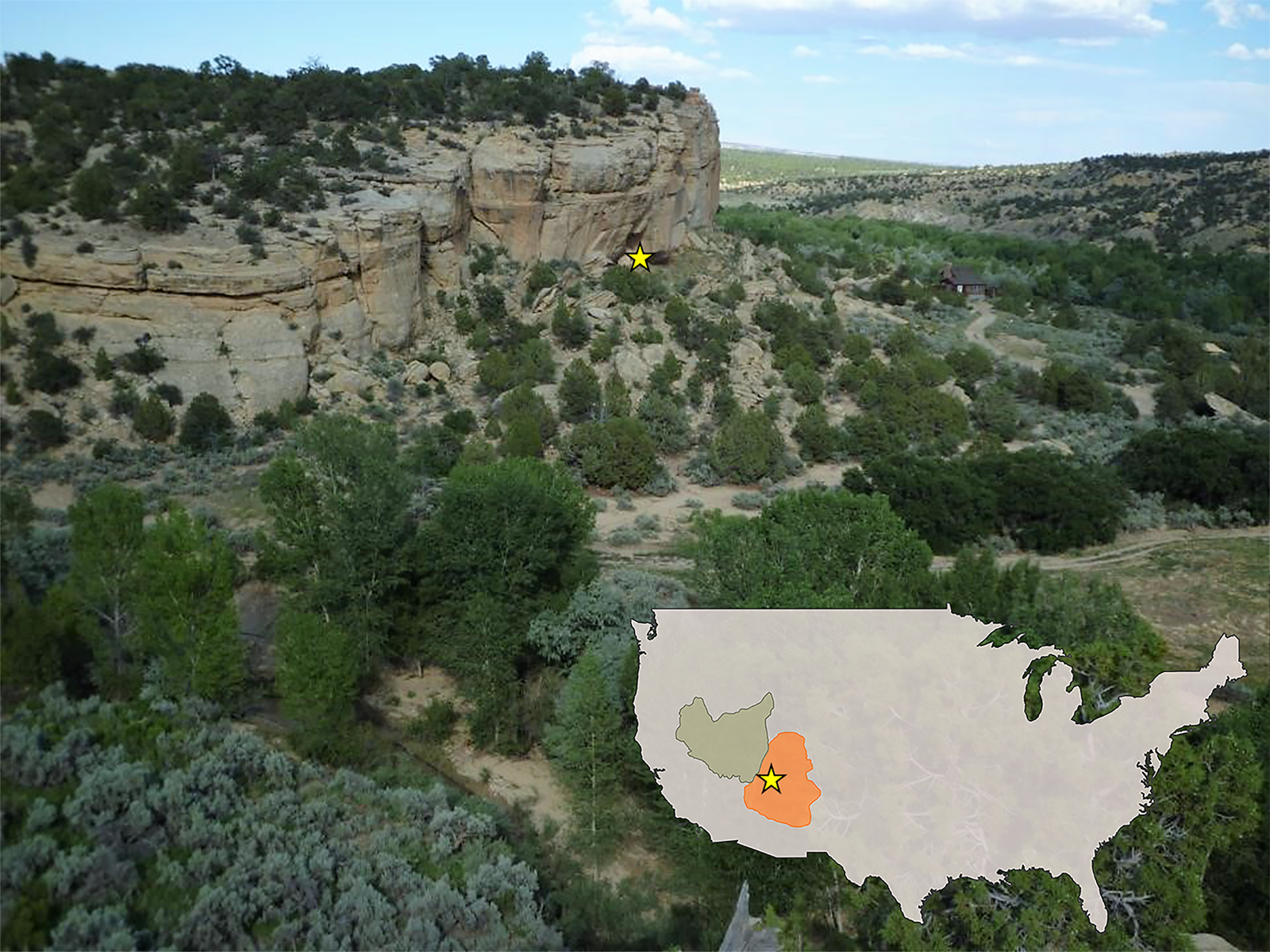
Figure 1. Location of North Creek Shelter (NCS) in Escalante on the Colorado Plateau in southern Utah (indicated by yellow star). Inset map shows the physiographic boundaries of the Great Basin (green) and Colorado Plateau (orange), and the yellow star is the approximate location of NCS.

Figure 2. Stratigraphic profile of North Creek Shelter (adapted from Janetski et al. Reference Janetski, Bodily, Newbold and Yoder2012).
The assumption that these use surfaces represent human activity areas and therefore behavioral patterns over time comes from several criteria that Janetski and colleagues (Reference Janetski, Bodily, Newbold and Yoder2012) describe. For example, compacted and reddened sediments underlie several use surfaces throughout strata II–V. Artifacts as well as pit and hearth features lie flat and originate from the same surface, measuring just a few centimeters from the reddened sediments. The patterned distribution of artifacts and features (especially those from strata II–V) were sealed rapidly by depositional events during the early Holocene (see Janetski et al. Reference Janetski, Bodily, Newbold and Yoder2012; Louderback et al. Reference Louderback, Kiahtipes and Janetski2020). Furthermore, refitting efforts have found that matches occur within these discrete use surfaces.
For this study, dietary faunal and botanical remains are examined from cultural strata II–VI in order to capture the major climatic and vegetation changes (see Louderback et al. Reference Louderback, Kiahtipes and Janetski2020) that might affect dietary choices during the early and middle Holocene and therefore reflect patterns in the diet breadth analyses. Stone tool abundances are also examined during this time period to determine if ground stone technology was developed at the same time as low-return resources were incorporated into the diet to maximize overall caloric returns. The chronological framework used by Louderback and colleagues (Reference Louderback, Kiahtipes and Janetski2020) is also used here. Radiocarbon ages for particular substrata (IIa, IIe, IIg, IIIa, IIIb, IIIe, Va, Vh, Vm, Vt, and VId) were calibrated using OxCal (Bronk-Ramsey and Lee Reference Bronk-Ramsey and Lee2013) and then averaged for substrata that did not have an associated radiocarbon age (Supplemental Figure 1; Supplemental Table 1).
Methods
Faunal Dietary Patterns
Faunal remains were recovered from a total of 53 substrata across strata II–VI. Descriptive summaries of the NCS mammalian fauna can be found in Janetski and colleagues (Reference Janetski, Bodily, Newbold and Yoder2012), Newbold (Reference Newbold2009), and Johannson (Reference Johansson2013). Previous evaluations of NCS faunal remains did not properly account for taphonomy, thereby potentially including noncultural taxa (e.g., carnivores and rodents) in analyses of diet breadth. This was avoided by using the annotated data records and segregating specimens into human and nonhuman accumulations based on burning and cut marks as cultural markers (see Louderback Reference Louderback2014:Appendices B and D). Additionally, relative abundance indexes had been previously calculated for cultural periods (e.g., Paleoarchaic and Early Archaic) rather than for individual substrata (e.g., Va, Vb, Vc, etc.), thereby blurring any quantitative changes that may have occurred. Consequently, relative abundances were recalculated for individual substrata rather than cultural periods. Applying a more rigorous assessment to faunal cultural markers and reexamining the NCS faunal remains by individual substrata resulted in a dietary dataset different from the ones previously published (Janetski et al. Reference Janetski, Bodily, Newbold and Yoder2012; Newbold Reference Newbold2009). This new assessment excludes carnivores and rodents as dietary taxa (both of which lacked cultural markers) but keeps artiodactyls and leporids (Supplemental Table 2). Taxa were included using the lowest taxonomic category that could be assigned with confidence. Taxa represented by a single specimen in any given substratum were excluded in order to minimize the effects of an accidental movement of specimens across strata (Grayson Reference Grayson1991). The same means of counting taxa and/or specimens was applied consistently across all assemblages. The number of individual specimens (NISP) for dietary faunal remains are provided in Supplemental Table 2 and are archived in Dryad (https://datadryad.org/stash/dataset/doi:10.5061/dryad.w9ghx3fq3).
Relative Abundance and Ubiquity
Relative abundance for each dietary faunal taxon was calculated using the following formula: ([Σ NISPtaxoni/Σ NISPall faunal taxa] × 100). It was used to measure changing abundances of a particular taxon over time, and it can inform about the importance of that taxon to past human diets. Relative abundance was calculated for both dietary faunal and botanical datasets.
The ubiquity index (expressed as a percentage) describes the occurrence of a taxon based on number of features or stratigraphic units in which that resource is recovered: % of taxoni = (N of substrata with taxoni/Σ N substrata) × 100 (Popper Reference Popper, Hastorf and Popper1988). Higher percentages indicate more pervasive use over time, which therefore has implications for the stability of resource availability as well as cultural importance. This was calculated for both faunal and botanical datasets.
Botanical Dietary Patterns
Macrobotanical remains (e.g., seeds, seed coats, fruits) were recovered by flotation analysis from hearth features (n = 156) excavated from 38 substrata spanning all cultural strata (II–VI) (Louderback Reference Louderback2014; Supplemental Table 2). Because these remains come from discrete hearth features (versus general cultural fill), they are considered human subsistence refuse if there is evidence of charring.
Identification of certain families is made possible by their diagnostic characteristics—such as the thick, ovoid, and large seed coats of Pinaceae or the angular, elongated, and small achenes of Asteraceae. Certain genera are also identified in this way; Chenopodium spp., for example, has lenticular, beaked, and rimmed seeds. C. berlandieri was isolated from all other Chenopodium spp. seeds because it has a distinctive reticulate pericarp and seed-coat patterning (Holmgren et al. Reference Holmgren, Holmgren and Reveal2012). Rhus sp. (sumac) seeds are ovoid with a radicle bulge (these specimens are distinctively striated, perhaps due to pericarp impression). In three cases, plant remains are identified to species: Atriplex confertifolia (shadscale) by its two-winged fruits, Achnatherum hymenoides (Indian ricegrass) by its distinctive flask-shaped achene, and Solanum jamesii (Four Corners potato) by its distinctive starch granules (Louderback and Pavlik Reference Louderback and Pavlik2017). Unidentified and indeterminate seeds and fruit are not included in the dietary analyses. The lowest possible taxonomic level was determined by using contemporary reference material from herbaria, seed reference collections, and plant specimens gathered at the study site itself. Local and regional floras are also used (e.g., Heil et al. Reference Heil, O'Kane, Reeves and Clifford2013; Welsh et al. Reference Welsh, Atwood, Goodrich and Higgins2015). Nomenclature conforms to USDA GRIN (https://www.ars-grin.gov/).
Ninety-four ground stone tools spanning strata V and VI were analyzed for starch granules. The majority of tools were made from sandstone (88%), with quartzite (6%), volcanics (5%), and granite (1%) comprising the remainder. The weight of the tools varied from 4 g to approximately 13.5 kg (see Louderback [Reference Louderback2014] for ground stone inventory and data). Starch granules were extracted following the protocols outlined in Louderback (Reference Louderback2014) and Louderback et alia (Reference Louderback, Field and Janetski2015), and granules were identified using the approached outlined in Louderback et alia (Reference Louderback, Herzog and Pavlik2017). Only starch granules with eccentric hila have been positively identified (Louderback and Pavlik Reference Louderback and Pavlik2017).
Because there is no way to determine an absolute count of starch granules, NISP is based on the number of ground stone tools that yielded identifiable granules in each substratum. For example, in substratum Vt, three of the 20 ground stone tools bore granules assigned to Solanum jamesii. Therefore, the NISP value for S. jamesii in substratum Vt is three. The NISP for dietary botanical remains are provided in Supplemental Table 2 and are archived in Dryad (https://datadryad.org/stash/dataset/doi:10.5061/dryad.w9ghx3fq3).
Relative Abundance and Ubiquity
Relative abundances and the ubiquity index were also calculated for dietary plant remains, using the same formulas mentioned above.
Abundance Indexes
The abundances of both higher- and lower-return resources are often used in archaeological studies to detect a broadening of the diet (e.g., Broughton Reference Broughton2002; Broughton et al. Reference Broughton, Cannon, Bayham and Byers2011; Codding et al. Reference Codding, Bird and Bird2010). Abundance indexes express variability in foraging efficiency by calculating the ratio of higher-return prey relative to lower-return prey, where 1.0 indicates the sample is composed entirely of higher-return prey and 0.0 indicates the sample is composed entirely of lower-return prey (Bayham Reference Bayham1979; Broughton Reference Broughton2002; Codding et al. Reference Codding, Bird and Bird2010; Grayson and Cannon Reference Grayson, Cannon and Beck1999; Nagaoka Reference Nagaoka2001).
Artiodactyl Index
Bones of artiodactyls and leporids, the two most abundant dietary remains in the NCS faunal assemblage, were used to calculate an artiodactyl index: (NISPArtiodactyls)/(NISPArtiodactyls + NISPLeporids). Artiodactyls are typically ranked higher than leporids due to their large body size (e.g., Bayham Reference Bayham1979, Broughton et al. Reference Broughton, Cannon, Bayham and Byers2011), but recent research suggests that high risks (i.e., failed pursuits) are often not taken into account (Bird et al. Reference Bird, Bird and Codding2009; Codding et al. Reference Codding, Bird and Bird2010). Furthermore, leporids have been found to be low risk and a reliable resource, thereby increasing their overall return rates (Lupo et al. Reference Lupo, Schmitt and Madsen2020; Morin et al. Reference Morin, Bird and Bird2020). This study uses body size as the primary measure of prey rank and therefore supports the suggestion that artiodactyls are ranked higher than leporids.
High-Return Plant Index
A high-return plant index was calculated and used to detect a broadening of the diet (e.g., Weiss et al. Reference Weiss, Wetterstrom, Nadel and Bar-Yosef2004). This index is the ratio of higher-return plant-part remains (i.e., Pinaceae large seeds, Quercus nuts, S. jamesii tubers) relative to lower-return plant-part remains (i.e., small seeds), where 1.0 indicates the sample is entirely higher-return and 0.0 indicates the sample is entirely lower-return.
The return rates of geophytes from western North America can range widely from 50 kcal/hr to up to 4,000 kcal/hr (Couture et al. Reference Couture, Ricks and Housley1986; Ugan and Rosenthal Reference Ugan and Rosenthal2016). The tubers included in this study come from Solanum jamesii, a wild potato that grows across the Four Corners region (Kinder et al. Reference Kinder, Adams and Wilson2017; Louderback and Pavlik Reference Louderback and Pavlik2017). A small population of S. jamesii is located approximately 150 m from NCS. Although return rate data are not yet available, this highly nutritious and productive perennial would have provided a reliable source of carbohydrates and minerals throughout the year. Handling costs would most likely have been minimal because its tubers are often found just below the litter layer in oak and conifer stands. Furthermore, S. jamesii tubers do not necessarily need to be cooked or roasted in order to be consumed. Various cooking and processing techniques for the tubers include drying, storing in a pit, and grinding into flour to make a bread (Castetter and Opler Reference Castetter and Opler1936). Given this information, S. jamesii tubers probably rank highly among geophytes with respect to post-encounter return rates.
Chipped Stone Tool Index
A chipped stone tool index was applied to determine when ground stone tools were employed at NCS. It is the ratio of chipped stone tools (i.e., projectile points) relative to ground stone tools (i.e., manos and metates), where 1.0 indicates the sample is composed entirely of chipped stone tools and 0.0 indicates the sample is composed entirely of ground stone tools. The NISP for chipped and ground stone tools are provided in Supplemental Table 2 and are archived in Dryad (https://datadryad.org/stash/dataset/doi:10.5061/dryad.w9ghx3fq3).
Climate Index
A climate index is calculated using the ratio between conifer and Amaranthaceae pollen analyzed from NCS sediments (Supplemental Table 2 and Dryad https://datadryad.org/stash/dataset/doi:10.5061/dryad.w9ghx3fq3), where 1.0 indicates the sample is composed entirely of conifer pollen (Abies, Picea, Pseudotsuga, Pinus, indicating cool conditions) and 0.0 indicates the sample is composed entirely of Amaranthaceae pollen (arid conditions; Louderback et al. Reference Louderback, Kiahtipes and Janetski2020). Other climate indexes could have been used in this study, including the cool-adapted/warm-adapted conifer ratio based on NCS plant macrofossils (Louderback et al. Reference Louderback, Kiahtipes and Janetski2020) or regional pollen and plant macrofossil records, such as Anderson and colleagues (Reference Anderson, Hasbargen, Koehler and Feiler1999), Betancourt (Reference Betancourt1984), Withers and Mead (Reference Withers and Mead1993), or Coats and colleagues (Reference Coats, Cole and Mead2008). All of these regional records corroborate the Holocene vegetation shifts and increasing aridity observed at NCS.
Temporal Sequencing
The artiodactyl and high-return plant indexes along with the chipped stone tool and climate indexes are plotted through time. A generalized linear model (GLM) of the binomial family (with quasi-likelihood estimation) and a “logit” link function was applied (McCullagh and Nelder Reference McCullagh and Nelder1989) and plotted using the R Programming Language (R Core Team 2019). The R code is published at Zenodo (https://doi.org/10.5281/zenodo.5123350). The quasibinomial GLM applies to proportional data and is therefore used for all indexes in this study.
Effects of Climate on Dietary Choices
Artiodactyl, chipped stone tool, and high-return plant indexes were regressed against the climate index to determine the effects of climate on dietary choices. If diet breadth and/or a shift to more expensive technology and low-return resources are coincident with climate change, then negative relationships should be apparent. A quasibinomial GLM with a “logit” link function was applied to each plot using the R Programming Language (R Core Team Reference Rhode2019). The R code is published at Zenodo (https://doi.org/10.5281/zenodo.5123350).
Results
Faunal Dietary Patterns
NISP and NTAXA for dietary faunal remains are presented in Supplemental Table 2. Six different dietary taxa were identified in the NCS faunal remains. High-ranked fauna include Antilocapra americana (pronghorn), Cervus elaphus (elk), Odocoileus hemionus (mule deer), and Ovis canadensis (bighorn sheep). Low-ranked fauna include Lepus sp. (hares) and Sylvilagus sp. (cottontail rabbits).
Relative Abundance and Ubiquity
Abundances of dietary faunal remains vary through time, ranging from one to over 350 bones in a single substratum. The lowest abundances occur throughout strata IV (10,500–10,000 cal BP) and VI (~7400–6700 cal BP). Higher abundances occur in strata II, III (11,300–10,500 cal BP), and V (9800–7600 cal BP). Deer make up 76% of the assemblage, regardless of when they were deposited, making this dietary resource both abundant and ubiquitous (87%; Figure 3). In contrast, Sylvilagus, Lepus, and Ovis bones are ubiquitous (85%, 66%, and 47%, respectively) but not very abundant (16%, 4%, and 4%, respectively). This suggests constant but low-level use. Other dietary faunal taxa (Antilocapra and Cervus) are neither abundant (totaling less than 0.7%) nor ubiquitous (11% and 8%, respectively), suggesting opportunistic or ephemeral use.

Figure 3. Relative abundance and ubiquity (frequency of occurrence) for dietary faunal and botanical datasets at NCS. Relative abundances for faunal and botanical taxa were calculated separately (ΣNISPfaunal = 2,871 and ΣNISPbotanical = 1,568). Ubiquity was also calculated separately (Σsubstratafaunal = 53, Σsubstratabotanical = 38).
Botanical Dietary Patterns
A total of 21 types of dietary plant taxa were recovered from 156 hearth features at NCS. This includes 17 families, 12 genera, and three that were identified to species level. Several potentially important dietary items are absent or rare, such as roots, tubers, berries, and other plants that lack dense, easily preserved parts. Some of these, such as tubers, may not show up in the macrobotanical record simply for reasons of differential preservation, but they do show up in the form of starch granules preserved in the cracks and crevices of ground stone tools. One such tuber, Solanum jamesii, was identified and included in dietary analyses for this study (see Louderback and Pavlik Reference Louderback and Pavlik2017).
Relative Abundance and Ubiquity Index
Abundances of dietary macrobotanical remains fluctuate through time, ranging from one to more than 280 seeds, seed coats, fruits, and fragments in a single substratum. The lowest abundances occur throughout strata II and IV (11,300–10,000 cal BP). These plant materials tend to be Pinaceae seeds. Higher abundances of dietary plant remains occur in substrata IIIc and IIIe (10,800–10,600 cal BP) as well as throughout strata V and VI (10,000–6700 cal BP). Remains of Chenopodium seeds and fruits are dominant in these deposits, although they are matched in abundance by Rhus sp. seeds in substrata Vh and Vi (9300–9100 cal BP). Overall, there is a progressive increase in dietary plant remains after substratum Va (~10,000 cal BP).
Chenopodium seeds make up 68% of the assemblage, regardless of when they were deposited, making this dietary resource both abundant and ubiquitous (84%; Figure 3). In contrast, Pinaceae seed coats and Sphaeralcea sp. seeds are ubiquitous (63% and 26%, respectively) but not very abundant (5% and 1%, respectively). This suggests constant but low-level use. Rhus sp. seeds, which are the second most abundant plant remains (15%), occur in only 13% of all strata. Most other dietary plant taxa are neither abundant nor ubiquitous, suggesting opportunistic or ephemeral use. It is noteworthy that members of Chenopodiaceae and Amaranthaceae have a total ubiquity index of 97%, therefore dominating the diet through a considerable period of time.
Abundance Indexes
Artiodactyl Index
Artiodactyl index values (Figure 4) are lowest throughout stratum IV (10,500–10,000 cal BP) as well as in substrata Vt, VIa, and VId (~8100–7000 cal BP). Values are highest in strata II and III (11,300–10,600 cal BP) as well as in substrata Vf–Vs (9400–8130 cal BP). Overall, the NCS assemblage is dominated by artiodactyls that, relative to leporids, do not decline significantly over time (p = 0.78; Supplemental Table 3).

Figure 4. Artiodactyl, high-return plant, chipped stone tool, and climate indexes plotted against time using datasets from NCS. Dashed lines represent 95% confidence intervals around the GLMs (quasibinomial with a “logit” link function). Correlation coefficient (r) and probabilities (P) of the GLM are indicated.
High-Return Plant Index
High-return plant index values (Figure 4) are highest in substrata IIa, IIc, IIe, IIIg, and Vb, which date from 11,300 to 9800 cal BP and then significantly decline (p < 0.01) as small seeds increase in abundance during the early and middle Holocene (~10,000–7000 cal BP; Supplemental Table 3). It is important to note, however, that tubers of S. jamesii were also collected between 8100 and 7600 cal BP (substrata Vt, Vu, VIa) and processed on ground stone tools.
Chipped Stone Tool Index
Chipped stone tool index values (Figure 4) are highest in strata II, III, and IV, which date from 11,300 to 10,000 cal BP. Ground stone tools first appear in substratum Va (9900 cal BP) and steadily increase in abundance (p < 0.001) until 6700 cal BP (Supplemental Table 3). This increase in ground stone tool use correlates with the increased abundance of all small seeds (r = +0.60, p < 0.0001; see Louderback Reference Louderback2014).
Climate Index
Climate index values (Figure 4) are highest in strata II, III, IV, and Va (11,300–9900 cal BP), indicating cool/moist conditions. But they begin to decline significantly in substrata Vf, Vi, Vq, Vs, VIa, and Vid (9500–6700 cal BP) when conditions become more arid (p < 0.0001; Louderback et al. Reference Louderback, Kiahtipes and Janetski2020; Supplemental Table 3).
Effects of Climate on Dietary Choices
There is no significant relationship between the abundance of higher-ranked faunal resources and climate (p = 0.87), but negative relationships, albeit nonsignificant, exist between the chipped stone tools and climate (p = 0.12) as well as the high-return plant resources and climate (p = 0.14; Figure 5; Supplemental Table 3). In other words, as climate gets warmer, the abundances of ground stone tools and small seeds increase.

Figure 5. Artiodactyl, chipped stone tool, and high-return plant indexes plotted against the climate index to determine the effects of climate on dietary choices. Dashed lines represent 95% confidence intervals around the GLMs (quasibinomial with a “logit” link function). Correlation coefficient (r) and probabilities (P) of the GLM are indicated.
Discussion
The diet breadth model (Stephens and Krebs Reference Stephens and Krebs1986) predicts that decreasing encounters with high-ranked resources should result in increasing use of lower-ranked resources, therefore reflecting adjustments in human foraging behavior. At North Creek Shelter, this prediction appears valid with respect to botanical resources because high-ranked plants such as pine nuts and acorns drop out of the diet after 9800 cal BP and are replaced with low-ranked, small seeds. The prediction is not met, however, with respect to the faunal resources where high-ranked artiodactyls are consistently abundant in the diet throughout the Holocene and are not replaced with low-ranked leporids for any sustained amount of time.
In arid regions on a global basis, increasing aridity and resultant changes in vegetation composition are offered as the ecosystem drivers of changes in dietary patterns (e.g., Edwards and O'Connell Reference Edwards and O'Connell1995; O'Connell and Hawkes Reference O'Connell, Hawkes, Winterhalder and Smith1981, Reference O'Connell and Hawkes1984; O'Connell et al. Reference O'Connell, Jones, Simms, Madsen and O'Connell1982; Rhode and Louderback Reference Rhode, Louderback, Graf and Schmitt2007; Rhode et al. Reference Rhode, Madsen and Jones2006). Increasing aridity at NCS began by 10,200 cal BP and progressed significantly by 9300 cal BP, which was indicated by a shift in vegetation from a mixed conifer forest of cool-adapted species to a semiarid woodland and shrub mosaic (Louderback et al. Reference Louderback, Kiahtipes and Janetski2020). This increased aridity coincides with greater use of small seeds and ground stone tools but not with corresponding increases in leporid abundances.
The NCS dataset contains fundamental lines of evidence encompassing diet and climate over time from a single site, which therefore allows for a true test of predictions of the diet breadth model. Other sites in the arid West that contain faunal, botanical, and stone tool datasets (e.g., Danger Cave, Bonneville Estates Rockshelter, Cowboy Cave, Sudden Shelter) were not analyzed specifically to test diet breadth predictions. Instead, they examined for differences between Paleoarchaic and Archaic subsistence patterns (Geib and Jolie Reference Geib, Jolie and Vierra2018; Madsen Reference Madsen, Graf and Schmitt2007; Rhode and Louderback Reference Rhode, Louderback, Graf and Schmitt2007; Rhode et al. Reference Rhode, Madsen and Jones2006). In examining these patterns, generalizations regarding diet breadth between Paleoarchaic and Archaic foragers were not quantitatively tested and were primarily based on a single line of evidence (e.g., ground stone tool abundance) rather than multiple indicators. In addition, some of those lines of evidence come from very different places and probably from different groups of people experiencing very different resource landscapes across time.
Two major questions arise from the current study:
(1) Why did high-ranked faunal resources not decline in the deposits of NCS when there was clear development of aridity and changes in regional vegetation? Faunal records from Great Basin archaeological sites during the late Pleistocene / early Holocene tend to be characterized by a diverse array of smaller, low-ranked prey such as birds, lagomorphs, fish, and insects (Grayson Reference Grayson1988; Hockett Reference Hockett2015; Hockett et al. Reference Hockett, Adams, Lubinski, Butler and Jenkins2017; Pinson Reference Pinson, Graf and Schmitt2007). Artiodactyls do not become abundant in those deposits until the middle Holocene (e.g., Hockett Reference Hockett2015; Pinson Reference Pinson, Graf and Schmitt2007), and they increase even more during the late Holocene (Broughton et al. Reference Broughton, Byers, Bryson, Eckerle and Madsen2008, Reference Broughton, Cannon, Bayham and Byers2011; Hockett Reference Hockett2015). At NCS, artiodactyls are as abundant during the early Holocene at 11,000 cal BP (substratum IIf, NISP = 181) as they are during the middle Holocene at 7900 cal BP (substratum Vu, NISP = 136), although abundances vary throughout these time periods. Perhaps local depletion of deer populations was regularly mitigated by immigration from distant sources. Unlike the Great Basin, the landscapes of the northern Colorado Plateau consist of nearly continuous and large areas of upland that may have provided corridors for constantly reestablishing herds in the vicinity of NCS, regardless of climate-driven vegetation change. In southern Utah, deer are now ubiquitous, occupying and moving between essentially all vegetation zones, from subalpine forests to desert scrub (Durrant Reference Durrant1952). That they would be present during the climatic shift to aridity between the early and middle Holocene is, therefore, not surprising. This has been suggested for other faunal patterns in the region (e.g., Sudden Shelter; Broughton et al. Reference Broughton, Cannon, Bayham and Byers2011; Byers and Broughton Reference Byers and Broughton2004).
Another hypothesis for large game hunting throughout the Holocene is based on costly signaling and “showing off” rather than foraging efficiency (Hildebrandt and McGuire Reference Hildebrandt and McGuire2002). The show-off hypothesis suggests that male hunters gain higher fitness by choosing “risky” resources that can be shared widely. Although this might explain the consistent or increased abundance of large game resources in archaeological sites, it has been refuted by many researchers on the basis of empirical, theoretical, and methodological evidence (Broughton and Bayham Reference Broughton and Bayham2003; Byers and Broughton Reference Byers and Broughton2004; Codding and Jones Reference Codding and Jones2007; Fisher Reference Fisher2015; Grimstead Reference Grimstead2010; Gurven and Hill Reference Gurven and Hill2009). More importantly, it has also been suggested that the show-off hypothesis may not result in the highest fitness-related benefits (Hawkes Reference Hawkes and Cashdan1990, Reference Hawkes1991).
(2) What is the driver for broadening of diets at NCS if not a decline in high-ranked faunal resources? The patterns observed from the NCS faunal and botanical datasets may reflect different foraging strategies between men and women, which would explain why low-ranked plant resources became increasingly abundant in the diet without a corresponding decrease in the abundance of high-ranked artiodactyls. Gender-specific foraging in the archaeological record is something that has been explored before, and in the case of the Carson Desert in Nevada, it has been modeled and tested using archaeological data (e.g., Elston and Zeanah Reference Elston and Zeanah2002; Kelly Reference Kelly, Larsen and Kelly1995b, Reference Kelly1997; Larsen and Hutchinson Reference Larsen, Hutchinson, Hemphill and Larsen1999; Larsen and Kelly Reference Larsen and Kelly1995; Larsen et al. Reference Larsen, Ruff, Kelly, Larsen and Kelly1995; Rhode Reference Rhode2003; Schoeninger Reference Schoeninger, Larsen and Kelly1995, Reference Schoeninger, Hemphill and Larsen1999; Zeanah Reference Zeanah2004). The prevailing consensus from those studies suggests that men and women had different goals: women stayed near home base to forage in productive lowland patches, whereas men forayed into the uplands for large-game resources (Zeanah Reference Zeanah2004). More recent research (Codding et al. Reference Codding, Bird and Bird2010; Elston et al. Reference Elston, Zeanah and Codding2014) elaborates on those findings by suggesting that some undefined level of variability in acquiring large game would lead to women simultaneously foraging for more reliable but lower-ranked resources. Likewise, the lack of ground stone tools in archaeological sites prior to 10,000 cal BP (Grayson Reference Grayson2011; Madsen Reference Madsen, Graf and Schmitt2007) suggests that gender-specific foraging patterns were more “convergent” (sensu Codding et al. Reference Codding, Bird and Bird2010; Elston et al. Reference Elston, Zeanah and Codding2014) because foragers would not have stayed in one location long enough for women to invest in that technology. Sometime after 10,000 cal BP, foraging patterns of men and women would have “diverged” if acquiring game was more unpredictable and foragers were staying in one place for longer periods of time.
These predictions regarding gender-specific foraging patterns are drawn from ethnographic studies of modern foragers. For example, gender and age differences in foraging patterns have been investigated among the Aché and the Hadza. The Aché are indigenous hunter-gatherers who live in the tropical forests of eastern Paraguay, and the Hadza are traditional foragers who inhabit the arid savannah woodland of northern Tanzania. Based on ethnographic studies, both of these groups defy diet breadth expectations that foragers will maximize their mean energetic rates because reproductive fitness costs and benefits may be different for men and women (O'Connell et al. Reference O'Connell, Hawkes and Blurton-Jones1999). Aché men and women, for example, have different foraging strategies based on their goals (Codding et al. Reference Codding, Brian, Bird and Bird2011; Hawkes Reference Hawkes and Cashdan1990; Hawkes et al. Reference Hawkes, O'Connell and Blurton-Jones1991; Hill et al. Reference Hill, Kaplan, Hawkes and Hurtado1987; Hurtado et al. Reference Hurtado, Hawkes, Hill and Kaplan1985). Aché women tend to be risk averse due to family provisioning. Consequently, they forage in more reliable resource patches, accumulating more low-return resources, heavily investing in plant-processing tools, and keeping the food for themselves and their children (Hawkes Reference Hawkes1991). Aché men pursue fluctuating but highly mobile large-bodied animals, and when they are successful, their prey is shared widely (Hawkes Reference Hawkes1991). Ethnographic studies also indicate that women's foraging strategies are good indicators of diet breadth due to risk-averse foraging goals as well as climate change, whereas men's are not (Hurtado et al. Reference Hurtado, Hawkes, Hill and Kaplan1985; O'Connell and Hawkes Reference O'Connell and Hawkes1984; O'Connell et al. Reference O'Connell, Hawkes and Blurton-Jones1999). In the case of the Hadza foragers, children are able to collect their own plant resources (i.e., fruits) during the wet season. But with increases in aridity and seasonality, those plant resources may not be available for children. Therefore, Hadza women provision with resources that tend to have high handling costs (such as small seeds and tubers; Hawkes et al. Reference Hawkes, O'Connell and Blurton-Jones1997; O'Connell et al. Reference O'Connell, Hawkes and Blurton-Jones1999; Wood et al. Reference Wood, Brian, Harris, Raichlen, Pontzer, Sayre, Sancilio and Berbesque2021). This is exactly what may be registered with the NCS record, and it is probably the best explanation for the differing patterns observed in the faunal and botanical assemblages at NCS.
At NCS, complementary faunal and botanical assemblages provide an opportunity to identify gender-specific foraging patterns across a long arc of time and environmental change. The current study posits that prior to 10,500 cal BP, variability in acquiring artiodactyls was low (coefficient of variation [CV] for artiodactyl index strata II = 0.18 and III = 0.13) compared to after (CV for strata IV = 0.54, V = 0.19 and VI = 0.19). This means that when NCS was surrounded in cool-adapted conifers, women were not staying in one place collecting small seeds and using ground stone tools to process them. Instead, they may have been participating in hunts and/or opportunistically collecting high-return plant food resources, such as large-seeded pine nuts. Gender-specific foraging patterns at NCS prior to 10,500 cal BP therefore seem to align with convergent patterns of labor division (Codding et al. Reference Codding, Bird and Bird2010; Elston et al. Reference Elston, Zeanah and Codding2014). After 10,500 cal BP, however, when conditions were becoming more arid, women apparently began collecting small seeds from various shrubby and herbaceous plants, as well as S. jamesii tubers (Louderback and Pavlik Reference Louderback and Pavlik2017) persisting beneath canopies and along watercourses. Ground stone tools were used to process those plant parts, thereby maximizing the overall caloric returns. Men, on the other hand, were consistently hunting artiodactyls, even though there was considerable variability in this record.
Conclusions
Although gender plays an important role in human foraging strategies (Hawkes and O'Connell Reference Hawkes and O'Connell1992; Jochim Reference Jochim1988), it is rarely addressed during archaeological studies, especially when considering diet breadth variations through time. The NCS archaeological assemblage suggests that women and men had different foraging patterns in response to environmental change. This could be tested more widely by applying optimal foraging models to many archaeological sites in the Great Basin and on the Colorado Plateau that also have complementary faunal, botanical, stone tool, and environmental datasets.
Acknowledgments
This research was supported by National Science Foundation (NSF) Doctoral Dissertation Improvement Grant (Award BCS-1262835), awarded to the author in 2012. The author would like to thank friends and colleagues who provided advice and constructive criticisms on early versions of the manuscript, including Jack Broughton, Brian Codding, Tyler Faith, Don Grayson, Kristen Hawkes, Joel Janetski, Jim O'Connell, David Rhode, and the University of Utah Archaeology Center Lab group. The author is also grateful to Bruce Pavlik for editing the manuscript and offering additional insights, as well as to Debra Martin and three anonymous reviewers. Thanks to Phoebe McNeally (Digit Lab, University of Utah) for making the map, Blake Vernon for providing R help, and Fanny Blauer for translating the abstract into Spanish. This research would not be possible without Joel Janetski's impeccable excavation of North Creek Shelter and his support throughout the author's dissertation research. And finally, thanks to the Rex family—especially Joette-Marie Rex—for hospitality, friendship, and continued access to North Creek Shelter.
Data Availability Statement
Data from this project are available and archived at Dryad: https://datadryad.org/stash/dataset/doi:10.5061/dryad.w9ghx3fq3. R code is published at Zenodo: https://doi.org/10.5281/zenodo.5123350.
Supplemental Material
For supplemental material accompanying this article, visit https://doi.org/10.1017/aaq.2021.122.
Supplemental Figure 1. Schematic diagram showing North Creek Shelter (NCS) radiocarbon age probability distributions by substratum. Calibrated ages are plotted as filled curves connected by a dotted line. Hatched curves are outlying or old wood ages (not used in this study). Probability distributions were calibrated and imported from OxCal (Bronk-Ramsey and Lee Reference Bronk-Ramsey and Lee2013). (Figure published in Louderback et al. Reference Louderback, Kiahtipes and Janetski2020.)
Supplemental Table 1. Radiocarbon Dates from North Creek Shelter.
Supplemental Table 2. Number of Individual Specimens (NISP) of Dietary Faunal and Botanical Remains, Chipped Stone and Ground Stone Tools, and Pollen from Substrata at North Creek Shelter.
Supplemental Table 3. Summary of Model Results Including the Estimate, Standard Error, T-Value and P-Value for the Parametric Terms.