As antibiotic-free animal production is in high demand, the effect of diet on intestinal health and immune function becomes an area of intense interest(Reference Sun and Kim1). The intestine is not only an important organ for nutrient digestion, absorption and metabolism but also harbours the largest number of immune cells in the body(Reference Ellen2). However, intestinal epithelial cells especially in young animals could be easily injured by inflammation and infection(Reference Liu3). Inflammation often results in intestinal mucosal damage and barrier function impairment, which negatively affects animal performance and health(Reference Xu, Chen and Wang4).
Polyphenols are secondary metabolites synthesised by plants to provide protection against invasive pathogens, such as bacteria, viruses and fungi, and also to prevent UV-induced damage to DNA and the photosynthetic apparatus(Reference Tangney and Rasmussen5). With anti-inflammatory or antioxidative activities, dietary polyphenols are well-known to exert a broad spectrum of health benefits(Reference Gessner, Ringseis and Eder6,Reference Fiesel, Gessner and Most7) . In addition, polyphenols were recently shown to alter intestinal microbiota and decrease the abundance of potentially pathogenic bacteria in faecal samples of piglets(Reference Fiesel, Gessner and Most7,Reference Jang, Sun and Chen8) .
To investigate whether dietary supplementation of holly polyphenol (HP) extracts could mitigate intestinal injury by regulating inflammation and hindgut microbiota, we fed pigs a basal diet with or without HP, followed by intraperitoneal administration of Escherichia coli lipopolysaccharide (LPS) to trigger gut inflammation and damage in the present study(Reference Xu, Chen and Wang4). We revealed that HP supplementation enhances intestinal disaccharidase activities, barrier function, the Prevotella abundance and SCFA production, while reducing the expression of proinflammatory mediators in LPS-challenged pigs.
Experimental methods
Animals and diets
Animal experiment was conducted in compliance with the Animal Scientific Procedures Act 1986 (Home Office Code of Practice. HMSO: London January 1997) and in accordance with EU regulation (Directive 2010/63/EU). Experimental procedures were approved by the Animal Care and Use Committee of Wuhan Polytechnic University (Wuhan, China). A total of twenty-four weanling crossbred castrated barrows (Duroc × Landrace × Large White, 35 (sem 1) d of age, 8·09 (sem 0·23) kg initial body weight) were used in the present study. Piglets were individually housed in stainless steel metabolic cages (1·80 × 1·10 m2) with free access to feed and water in an environmentally controlled house. The ambient temperature was maintained at 22–25°C. The maize–soyabean meal basal diet (Table 1) was formulated in accordance with the National Research Council requirements(9). The basal diet was also supplemented without or with 250 mg/kg of a commercial HP product containing 65·5 % total polyphenols with main ingredients being phenolic acids and tannins.
Table 1. Composition of the experimental diet (%, as-fed basis)
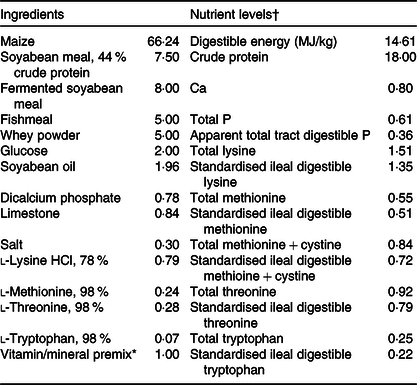
* The nutrient levels were analysed values except digestible energy, apparent total tract digestible P, standardised ileal digestible lysine, methionine, methionine + cystine, threonine and tryptophan, which are calculated values.
† Premix supplied per kg diet: retinyl acetate, 2700 μg; cholecalciferol, 62·5 μg; dl-α-tocopheryl acetate, 20 mg; menadione sodium bisulphite complex, 4 mg; riboflavin, 5·22 mg; d-calcium-pantothenate, 20 mg; niacin, 26 mg; vitamin B12, 0·01 mg; Mn (MnSO4·H2O), 40 mg; Fe (FeSO4·H2O), 75 mg; Zn (ZnSO4·7H2O), 75 mg; Cu (CuSO4·5H2O), 100 mg; iodine (CaI2), 0·3 mg; Se (Na2SeO3), 0·3 mg.
Experimental design
The experiment was a 2 × 2 factorial design as previous report described(Reference Xu, Chen and Wang4). Piglets were fed basal or HP diet for 16 d, followed by intraperitoneal injection of LPS (E. coli O55:B5; purity > 99 %; Sigma-Aldrich) at 100 μg/kg body weight in saline or the same volume of saline. The main factors included diet type (basal or HP diet) and immunological challenge (LPS or saline). Body weight and feed intake were recorded on d 1 and d 17 (4 h post-LPS challenge).
Blood and intestinal sample collection
At 4 h after injection with saline or LPS, blood samples (8 ml) were collected from the anterior vena cava into heparinised vacuum tubes. Plasma was isolated by centrifugation of the blood samples at 3000 RPM and 4°C for 10 min and subsequently transferred into two microcentrifuge tubes (2 ml). Plasma samples were stored at –80°C for further analysis. Following blood collection, all piglets were humanely killed by injection of sodium pentobarbital. Both a 3-cm and a 10-cm segment were collected from each of the mid-jejunum and mid-ileum as we previously described(Reference Xu, Wang and Wu10). The 3-cm intestinal segment was gently flushed and stored in fresh 4 % paraformaldehyde/PBS for histological analysis, while the 10-cm segment was opened longitudinally and flushed gently to remove the luminal content. The mucosal samples were collected by scraping with sterile glass slides, then rapidly frozen in liquid N2 and stored at –80°C for measurement of enzymatic activities and mRNA expression levels of disaccharidases. All intestinal samples were collected within 15 min after slaughter.
Measurement of plasma cytokines and hormones
Plasma concentrations of cytokines and hormones including TNF-α, IL-6, insulin, growth hormone, cortisol and glucagon were measured using commercial ELISA kits as we described(Reference Xu, Chen and Wang4,Reference Xu, Liu and Long11) .
Intestinal morphometric measurement
The fixed intestinal segments were processed, embedded in paraffin, sectioned at 5-um thickness and stained with haematoxylin–eosin. Villus height and crypt depth were measured at 40× magnification. A minimum of ten well-oriented and intact villi were selected. Villus height was measured from the tip of a villus to the villus-crypt junction, while crypt depth was defined as the depth of the invagination between adjacent villi as described(Reference Xu, Wang and Wu10).
Intestinal mucosa disaccharidase activities
Disaccharidase activities in the supernatant of intestinal mucosa were determined as previously described(Reference Xu, Chen and Wang4) using a glucose kit (no. A082-1 for lactase, no. A082-2 for sucrase and no. A082-3 for maltase; Nanjing Jiancheng Bioengineering Institute). The protein concentrations of intestinal mucosa were determined using Coomassie Brilliant Blue G-250 with bovine serum albumin as the standard.
mRNA abundance analysis by real-time PCR
The methods for total RNA isolation, quantification, reverse transcription and real-time PCR were the same as Pi et al.(Reference Pi, Liu and Shi12). The primer pairs used are presented in Table 2 to amplify the target genes. The 2–△△CT method was used to analyse the mRNA abundance of the target genes relative to a housekeeping gene (GAPDH)(Reference Livak and Schmittgen13). In our study, GAPDH displayed no variation among four treatments. Relative mRNA abundance of each target gene was normalised to the pigs receiving basal diet and treated with 0·9 % NaCl.
Table 2. Primer sequences used for real-time PCR

COX2, cyclo-oxygenase 2; GAPDH, glyceraldehyde-3-phosphate dehydrogenase; HSP70, heat shock protein 70; IRAK1, IL-1 receptor-associated kinase; MyD88, myeloid differentiation factor 88; NOD1, nucleotide-binding oligomerisation domain protein 1; NOD2, nucleotide-binding oligomerisation domain protein 2; RIPK2, receptor-interacting protein kinase 2; TLR4, toll-like receptor 4; TRAF6, TNF receptor-associated factor 6; ZO-1, zona occludins 1.
SCFA analysis
The concentrations of SCFA in caecal and colonic digesta were analysed as previously described(Reference Wang, Wang and Wang14) using GC (HP 6890 Series, Hewlett Packard) equipped with a HP 19091N-213 column with 30·0 m × 0·32 mm internal diameter (Agilent). The injector and detector temperatures were 185 and 210°C, respectively.
Microbial diversity analysis
DNA extraction, PCR amplification, DNA quantification and sequencing were performed commercially in accordance with the standardised protocol of Shanghai Majorbio Bio-Pharm Technology. In brief, microbial DNA was extracted from caecal digesta with a FastDNA Spin Kit (no. 116560200; MP Biomedicals). The V3–V4 region of the bacteria 16S rRNA gene was amplified by PCR using 338F/806R barcoded primers. PCR amplification products were quantified with a QuantiFluorTM-ST Handheld Fluorometer with UV/Blue Channels (Promega Corporation), normalised and then sequenced on Miseq (Illumina). Operational taxonomic units (OTU) were clustered with 97 % similarity cut-off using Usearch (version 7.1; http://drive5.com/uparse/). The taxonomy of each 16S rRNA gene sequence was analysed using RDP Classifier (version 2.2; http://sourceforge.net/projects/rdp-classifier/) against the Silva bacterial 16S rRNA database (Release128; http://www.arb-silva.de) with confidence threshold of 70 %. Analyses for rarefaction curves, and calculation of the Good’s coverage, ACE, Chao, and Simpson diversity indices were performed using the MOTHUR software (version v.1.30.1; http://www.mothur.org/wiki/Schloss_SOP#Alpha_diversity).
Statistical analysis
The growth performance data were analysed with Student’s t test using SAS (SAS Institute Inc.). Other data were analysed as a 2 × 2 factorial design using the GLM procedure of SAS. The individual piglet was the experimental unit for all parameters. The factors in the model included diet type, LPS challenge and their interaction. Least-squares means were derived for all treatments and were compared using the PDIFF (adjusted Tukey) and STDERR options of SAS. Results are expressed as least-squares means with their standard errors. When diet type × LPS challenge was significant (P < 0·05) or showed a strong trend (0·05 ≤ P ≤ 0·1), post hoc testing was performed using least significant difference (LSD) multiple comparison tests. In addition, partial least-squares discriminant analysis was performed using the R package ‘mixOmics’ to compare bacterial composition between four groups.
Results
Growth performance
Throughout the entire 16 d experiment (before LPS challenge), no differences were observed for average daily gain (486 v. 461 g), average daily feed intake (1057 v. 894 g) and feed:gain ratio (2·17 v. 1·94) between control and HP diet groups (Table 3).
Table 3. Effects of holly polyphenols on growth performance of weanling piglets (n 6 (1 piglet/pen))
(Mean values with their pooled standard errors)
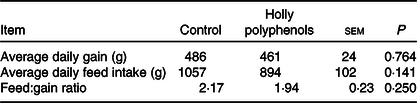
Intestinal morphology
Diet type and LPS challenge had no significant effect on intestinal villus height and crypt depth (Table 4). However, there was a significant interaction between diet type and LPS challenge for intestinal villus height:crypt depth ratio (P < 0·05). The piglets challenged with LPS had decreased villus height:crypt depth ratio compared with piglets without LPS challenge (P < 0·05). Compared with the control piglets, villus height:crypt depth ratio in HP-supplemented piglets significantly decreased (P < 0·05). Interestingly, villus height:crypt depth ratio in LPS-challenged piglets between the two diet groups had no difference.
Table 4. Effects of holly polyphenols on ileal morphology after lipopolysaccharide (LPS) challenge in weanling pigs (n 6 (1 piglet/pen))
(Mean values with their pooled standard errors)

a,b Mean values within a row with unlike superscript letters were significantly different (P < 0·05).
Intestinal disaccharidase activities
There was a significant interaction between diet type and LPS challenge for the jejunal lactase activity (P < 0·05, Table 5). Dietary HP increased the jejunal lactase activity of the piglets challenged with LPS (P < 0·05). LPS challenge reduced the activities of sucrase, maltase and lactase in the ileum (P < 0·05). The piglets fed HP had higher activities of sucrase and lactase in the jejunum (P < 0·05) and sucrase in the ileum (P = 0·071) compared with piglets fed the control diet.
Table 5. Effect of holly polyphenols on the intestinal disaccharidase activities after lipopolysaccharide (LPS) challenge in weanling pigs (U/mg protein) (n 6 (1 piglet/pen))
(Mean values with their pooled standard errors)
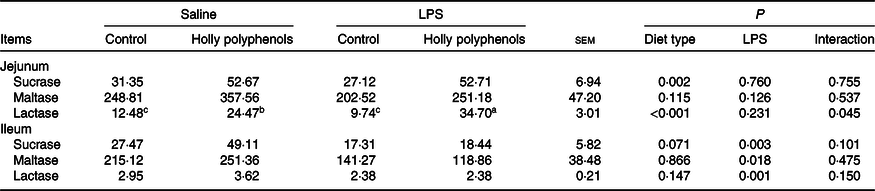
a,b,c Mean values within a row with unlike superscript letters were significantly different (P < 0·05).
Plasma inflammatory cytokines and hormone concentrations
There was no significant interaction between diet type and LPS challenge for plasma inflammatory cytokine and hormone concentrations (Table 6). LPS-challenged piglets had higher concentrations of TNF-α, IL-6, cortisol and glucagon compared with non-challenged piglets (P < 0·05). Meanwhile, the insulin level of LPS-challenged piglets significantly decreased (P < 0·05). Supplementation with HP significantly reduced the concentrations of TNF-α, IL-6 and insulin (P < 0·05). However, the piglets fed HP diets had a higher concentration of plasma glucagon (P = 0·067).
Table 6. Effect of holly polyphenols on plasma TNF-α, IL-6, insulin, growth hormone (GH), cortisol and glucagon concentrations after lipopolysaccharide (LPS) challenge in weanling pigs (n 6 (1 piglet/pen))
(Mean values with their pooled standard errors)

ND, not determined.
mRNA expression of intestinal tight junction proteins
There was a significant interaction between diet type and LPS challenge for jejunal mRNA expression of claudin-1 (P < 0·001, Table 7). Supplementation with HP significantly increased mRNA expression of claudin-1 in the jejunum of LPS-challenged piglets (P < 0·05). HP also enhanced mRNA expression of zona occludens 1 in the jejunum (P = 0·098). On the other hand, LPS reduced mRNA abundance of jejunal and ileal occludin and ileal claudin-1 (P < 0·05), while HP restored the LPS-induced suppression of the claudin-1 and occludin expression in the ileum (P < 0·05).
Table 7. Effects of holly polyphenols on intestinal mRNA expression of tight junction proteins after lipopolysaccharide (LPS) challenge in weanling pigs (n 6 (1 piglet/pen))
(Mean values with their pooled standard errors)
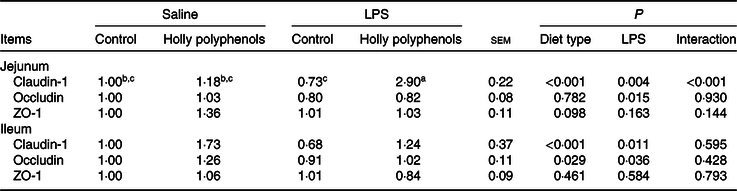
ZO-1, zona occludins 1.
a,b,c Mean values within a row with unlike superscript letters were significantly different (P < 0·05).
mRNA abundance of intestinal inflammatory mediators
Relative to control piglets, LPS-challenged piglets had higher mRNA abundance of jejunal toll-like receptor 4, receptor interacting protein kinase 2, cyclo-oxygenase 2 and heat shock protein 70 (HSP70) (P < 0·05, Table 8). There was a significant diet type × LPS challenge interaction observed for mRNA abundance of jejunal nucleotide-binding oligomerisation domain protein 1 (P < 0·05). LPS also increased mRNA abundance of ileal toll-like receptor 4, TNF-α receptor-associated factor 6, IL-1 receptor-associated kinase 1, nucleotide-binding oligomerisation domain protein 2, receptor interacting protein kinase 2, and cyclo-oxygenase 2 (P < 0·05). HP supplementation, on the other hand, suppressed the expression of ileal TNF-α receptor-associated factor 6 (P = 0·05). There was a significant diet type × LPS challenge interaction observed for ileal HSP70 (P < 0·05). Supplementation with HP significantly increased mRNA expression of ileal HSP70 in the LPS-challenged piglets (P < 0·05).
Table 8. Effects of holly polyphenol supplementation on intestinal mRNA expression of inflammatory pathways after lipopolysaccharide (LPS) challenge in weanling pigs (n 6 (1 piglet/pen))
(Mean values with their pooled standard errors)
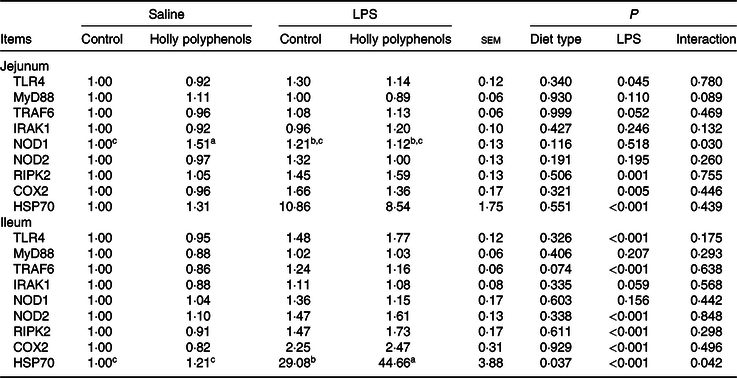
TLR4, toll-like receptor 4; MyD88, myeloid differentiation factor 88; TRAF6, TNF receptor-associated factor 6; IRAK1, IL-1 receptor-associated kinase; NOD1, nucleotide-binding oligomerisation domain protein 1; NOD2, nucleotide-binding oligomerisation domain protein 2; RIPK2, receptor-interacting protein kinase 2; COX2, cyclo-oxygenase 2; HSP70, heat shock protein 70.
a,b,c Mean values within a row with unlike superscript letters were significantly different (P < 0·05).
Bacterial composition in the caecal and colonic digesta
In total, 983 024 and 937 361 high-quality sequence reads were obtained from forty-eight caecal and colonic digesta samples, respectively. The Good’s coverage of each group was over 99 %, and the rarefaction curves showed clear asymptotes, indicating a near complete sampling of the community. Table 9 shows the bacterial community richness (ACE and Chao) and the diversity indices (Shannon). There were no significant differences in most indices, except that LPS challenge increased Shannon index in the caecal digesta (P < 0·05). Core OTU analysis among the four groups was shown in Fig. 1. In the caecum, there were 561, 524, 527 and 508 core OTU in each of these four individual groups, respectively, with a total of 428 core OTU common to all groups (Fig. 1(a)). Meanwhile, there were 584, 537, 522 and 521 core OTU in each of these four individual groups, respectively, with a total of 454 core OTU common to all groups in the colon (Fig. 1(b)).
Table 9. Effects of holly polyphenol supplementation on bacterial communities diversity in caecal and colonic digesta after lipopolysaccharide (LPS) challenge in weanling pigs (n 6 (1 piglet/pen))
(Mean values with their pooled standard errors)
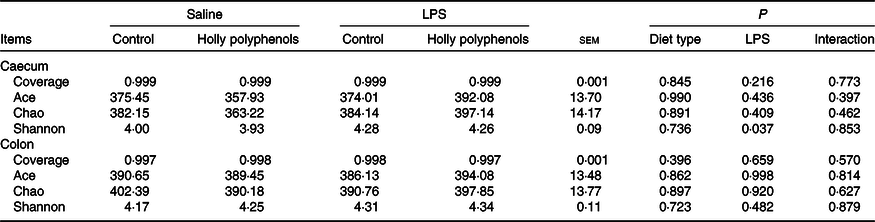
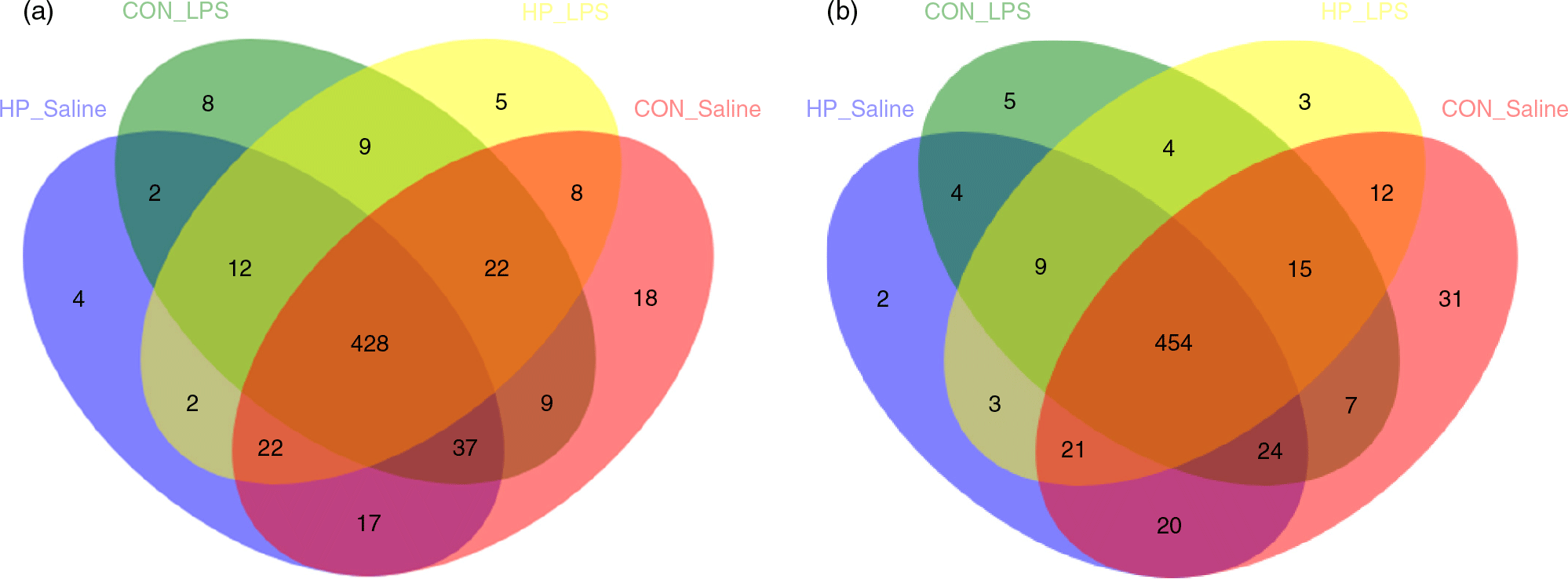
Fig. 1. Venn diagram of core operational taxonomic units in the caecal (a) and colonic (b) digesta after lipopolysaccharide (LPS) challenge in weanling pigs. CON_Saline, piglets fed the control diet and injected with saline; HP_Saline, piglets fed holly polyphenols and injected with saline; CON_LPS, piglets fed the control diet and challenged with LPS; HP_LPS, piglets fed holly polyphenols and challenged with LPS (n 6; 1 pig/pen).
Bacterial compositions in the caecum and colon of the four treatments were analysed at the phylum and genus levels (Figs. 2 and 3, Table 10). At the phylum level, the caecal and colonic microbiota mainly consisted of Firmicutes, Bacteroidetes, Actinobacteria and Proteobacteria. In the caecum, there was no significant difference in the microbiota among the four groups. However, LPS challenge increased the abundance of Firmicutes (P < 0·05) and Actinobacteria (P = 0·094) and reduced the abundance of Bacteroidetes (P < 0·05) in the colon. HP supplementation had a higher abundance of Firmicutes and reduced Bacteroidetes in the colon (P < 0·05). Furthermore, top thirty bacterial genera were further analysed (Fig. 4). LPS challenge reduced relative abundance of Prevotella_9 in the colon. HP increased relative abundance of Prevotella_9 in the caecum and Lactobacillus both in the caecum and colon of LPS-challenged pigs.

Fig. 2. Relative abundance of the bacterial composition in the caecal (a) and colonic (b) digesta in weanling pigs at the phylum level. CON_Saline, piglets fed the control diet and injected with saline; HP_Saline, piglets fed holly polyphenols and injected with saline; CON_LPS, piglets fed the control diet and challenged with lipopolysaccharide; HP_LPS, piglets fed holly polyphenols and challenged with lipopolysaccharide (n 6; 1 pig/pen). , Firmicutes;
, Bacteroidetes;
, Actinobacteria;
, Proteobacteria;
, others; (b)
, Firmicutes;
, Bacteroidetes;
, Actinobacteria;
, Proteobacteria;
, Spirochaetae;
, others.
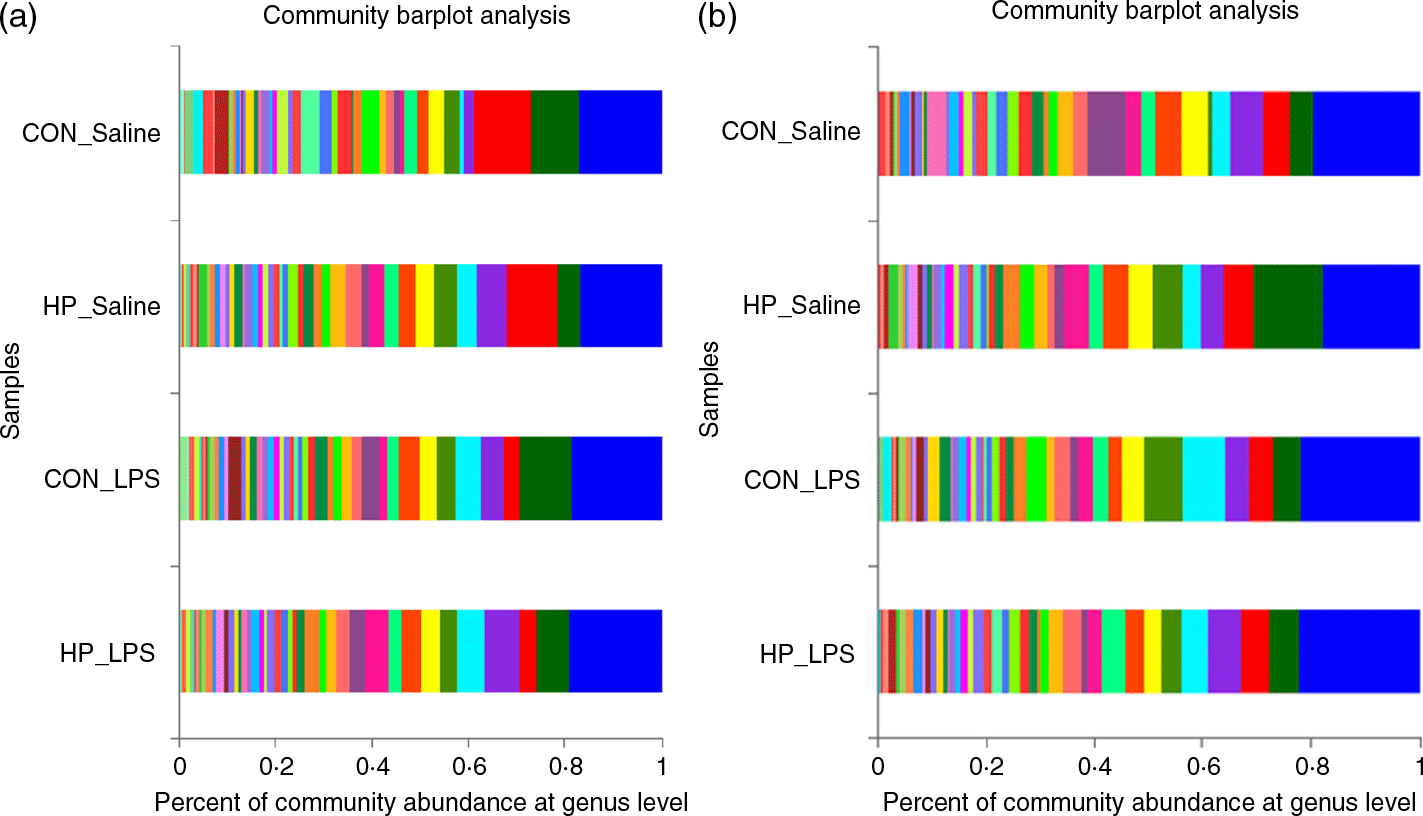
Fig. 3. Relative abundance of the bacterial composition in the caecal (a) and colonic (b) digesta in weanling pigs at the genus level. CON_Saline, piglets fed the control diet and injected with saline; HP_Saline, piglets fed holly polyphenols and injected with saline; CON_LPS, piglets fed the control diet and challenged with lipopolysaccharide; HP_LPS, piglets fed holly polyphenols and challenged with lipopolysaccharide (n 6; 1 pig/pen). (a) , Prevotellaceae_NK3B31_group;
, Prevotella_9;
, Blautia;
, Lactobacillus;
, unclassified_f_Lachnospiraceae;
, Rikenellaceae_RC9_gut_group;
, Faecalibacterium;
, norank_f_bacteroidales_S24-7_group;
, Megamonas;
, Megasphaera;
, Subdoligranulum;
, Ruminococcus_1;
, Intestinibacter;
, Phascolarctobacterium;
, Acidaminococcus;
, Ruminococcaceae_UCG-005;
, Prevotella_7;
, Prevotella_1;
, Terrisporobacter;
, Ruminococcaceae_UCG-008;
, Anaerovibrio;
, Parabacteroides;
, Collinsella;
, norank_f_Prevotellaceae;
, Cooprococcus_3;
, Prevotella_2;
, Ruminococcaceae_UCG-002;
, Ruminococcaceae_UCG-014;
, Dialister;
, Oribacterium;
, [Eubacterium]_hallii_group;
, Dorea;
, [Eubacterium]_coprostanoligenes_group;
, Selenomonas;
, Clostridium_sensu_stricto_1;
, Catenibacterium;
, unclassified_c_Bacteroidia;
, Streptococcus;
, Prevotellaceae_UCG-003;
, Catenisphaera;
, Holdemanella;
, Treponema_2;
, Escherichia-Shigella;
, others. (b)
, Prevotella_9;
, Faecalibacterium;
, Blautia;
, Prevotellaceae_NK3B31_group;
, Lactobacillus;
, unclassified_f_Lachnospiraceae;
, Subdoligranulum;
, Intestinibacter;
, Phascolarctobacterium;
, Terrisporobacter;
, Cooprococcus_3;
, [Eubacterium]_coprostanoligenes_group;
, Alloprevotella;
, Anaerovibrio;
, Ruminococcus_1;
, Sarcina;
, Parabacteroides;
, Solobacterium;
, Ruminococcaceae_UCG-008;
, Leeia;
, Holdemanella;
, norank_f_bacteroidales_S24-7_group;
, Dorea;
, Ruminococcaceae_UCG-005;
, Collinsella;
, Clostridium_sensu_stricto_1;
, Megamonas;
, Dialister;
, [Eubacterium]_hallii_group;
, Prevotella_7;
, Prevotellaceae_UCG-003;
, Streptococcus;
, Ruminococcaceae_UCG-014;
, Anaerotruncus;
, Selenomonas;
, Intestinimonas;
, Ruminococcaceae_NK4A214_group;
, Ruminococcaceae_UCG-002;
, Megasphaera;
, Escherichia-Shigella;
, others.
Table 10. Effects of holly polyphenol supplementation on bacterial composition in caecal and colonic digesta after lipopolysaccharide (LPS) challenge in weanling pigs (n 6 (1 piglet/pen))
(Mean values with their pooled standard errors)
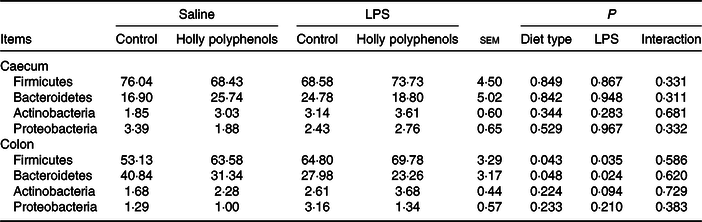
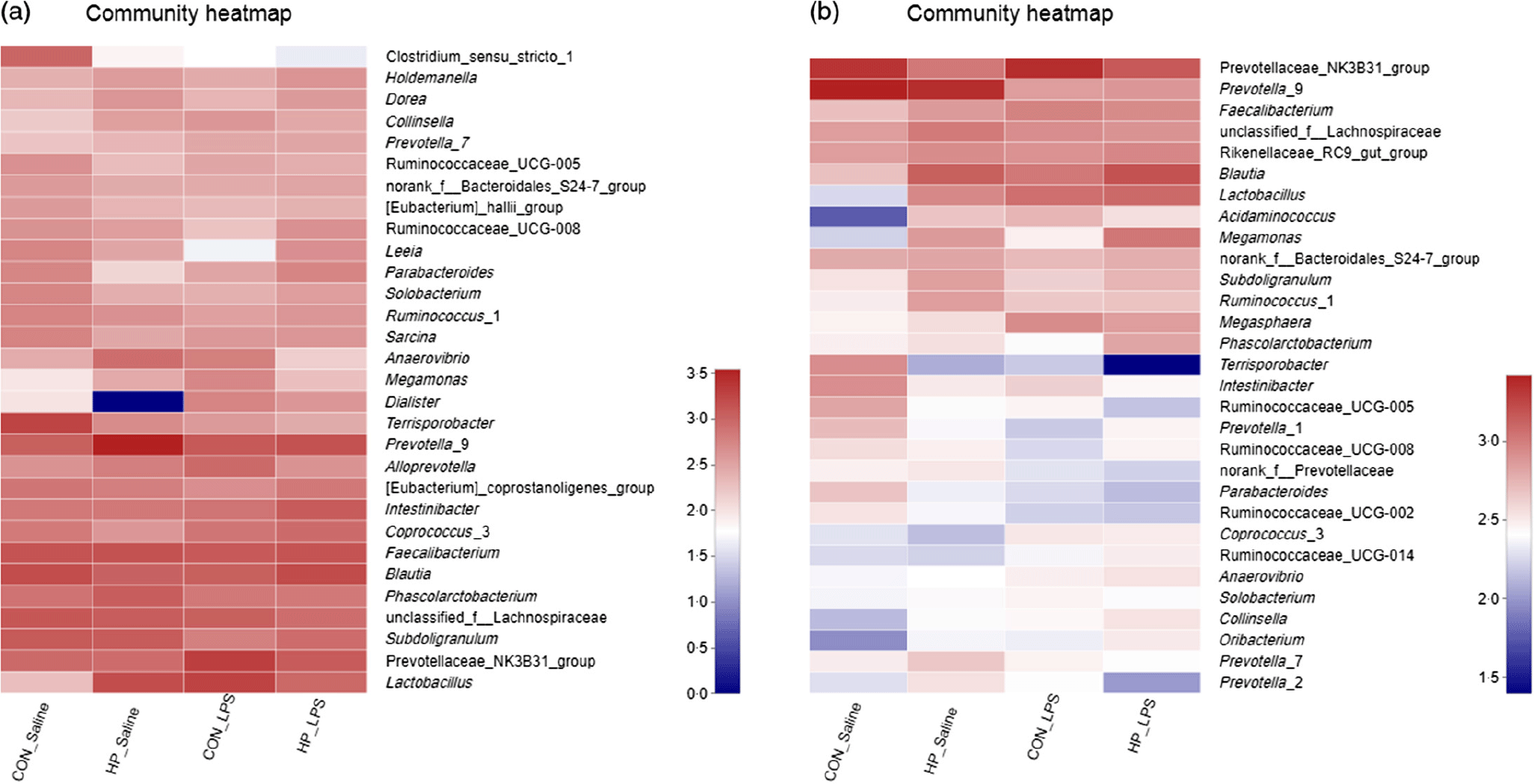
Fig. 4. Heatmap of relative abundance of top thirty bacterial genera in the caecal (a) and colonic (b) digesta in weanling pigs. CON_Saline, piglets fed the control diet and injected with saline; HP_Saline, piglets fed holly polyphenols and injected with saline; CON_LPS, piglets fed the control diet and challenged with lipopolysaccharide; HP_LPS, piglets fed holly polyphenols and challenged with lipopolysaccharide (n 6; 1 pig/pen).
SCFA concentrations in caecal and colonic digesta
LPS increased the concentrations of butyrate and isovalerate both in the caecum and colon, as well as valerate in the colon (P < 0·05, Table 11). HP significantly increased the concentrations of caecal valerate and colonic acetate and isovalerate in LPS-challenged piglets (P < 0·05).
Table 11. Effects of holly polyphenol supplementation on concentrations of SCFA in caecal and colonic digesta after lipopolysaccharide (LPS) challenge in weanling pigs (mg/g) (n 6 (1 piglet/pen))
(Mean values with their pooled standard errors)
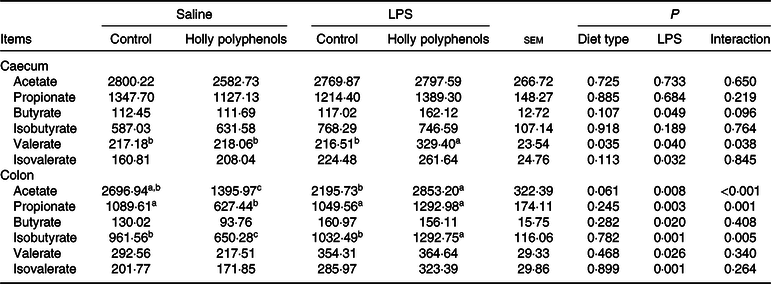
a,b,c Mean values within a row with unlike superscript letters were significantly different (P < 0·05).
Discussion
In the present study, we explored the effect of HP supplementation on intestinal integrity and proinflammatory gene expression after a 4-h E. coli LPS challenge in a piglet model. The intraperitoneal injection of LPS is widely used as an experimental model in the study of endotoxin-induced acute intestinal injury(Reference Xu, Chen and Wang4,Reference Liu, Huang and Hou15) . Polyphenols, as the secondary plant metabolites, have been proved to play a role in protection against invasive pathogens, such as bacteria, viruses and fungi(Reference Tangney and Rasmussen5). In the present study, we showed that dietary supplementation with HP improved the intestinal disaccharidase activities and mRNA expression of intestinal tight junction proteins. Furthermore, HP reduced plasma inflammatory cytokine concentration, altered microbiota composition and protected intestinal barrier integrity in LPS-challenged piglets.
HP failed to improve the growth performance of healthy piglets prior to LPS challenge, which is consistent with previous studies(Reference Zhang, Xian and Mantovani16,Reference Wang, Zhang and Peng17) . The lack of an effect from the plant polyphenols on growth performance may be due to some anti-nutritional properties of certain polyphenols such as the inactivation of certain polyphenols by dietary protein(Reference Verhelst, Schroyen and Buys18,Reference Rossi, Pastorelli and Corino19) .
Tight junctions maintain gut homoeostasis by preventing the penetration of luminal bacteria and dietary allergens into the mucosa(Reference Lee and Luk20), and intestinal injury is closely associated with changes in tight junctions(Reference Xu, Chen and Wang4). In the present study, LPS caused intestinal barrier injury in weanling piglets by decreasing intestinal mRNA expressions of claudin-1 and occludin, while HP restored barrier integrity by enhancing the expression of claudin-1, occludin and zona occludens 1 mRNA expression in LPS-challenged pigs.
Disaccharides must be hydrolysed to monosaccharides by disaccharidases such as lactase, maltase and sucrase to be absorbed(Reference Weightac, Jonesac and Horna21). Therefore, the disaccharidase activities are involved in the energy metabolism and good indicators of intestinal digestive function(Reference Xu, Chen and Wang4). In the present study, the activities of lactase, sucrase and maltase in the ileum were all reduced by LPS, which is in agreement with previous studies(Reference Xu, Chen and Wang4,Reference Wang, Liu and Li22) . HP supplementation increased the sucrase activity in both the jejunum and ileum of healthy piglets and jejunal lactase in LPS-challenged piglets, suggesting that dietary polyphenols could improve the intestinal digestive function of weanling piglets. The plasma cortisol concentration was increased in the piglets challenged with LPS, which is similar to the previous studies(Reference Wright, Balaji and Hill23,Reference Schwinn, Knight and Bruckmaier24) . LPS leads to immune stress and affected nutrients digestion and metabolism. The decreased insulin and increased glucagon concentration in the plasma of piglets challenged with LPS illustrated that LPS challenge affected energy metabolism which is in accordance with the results of reduced disaccharide activities. HP decreased insulin and increased glucagon concentration in plasma which is similar to previous studies(Reference Manzano, Giron and Vilchez25,Reference Cao, Sethumadhavan and Li26) . Plant polyphenol extract is a good kind of food additive to improve insulin sensitivity and decrease plasma glucose concentration(Reference Manzano, Giron and Vilchez25,Reference Cao, Sethumadhavan and Li26) .
We hypothesised that HP exerts its beneficial effect on the intestine by reducing inflammatory response. Similar to proinflammatory cytokines such as TNF-α and IL-6, increased expression of HSP70 and cyclo-oxygenase 2 is indicative of intestinal inflammation(Reference Chen, Liu and Zhu27,Reference Westbrook, Wei and Hacke28) . In the present study, LPS challenge indeed resulted in higher TNF-α and IL-6 concentrations in plasma and higher mRNA expression of HSP70 and cyclo-oxygenase 2 in the jejunum and ileum. However, HP supplementation suppressed LPS-mediated increase in the concentrations of TNF-α and IL-6 in plasma, suggesting that HP was effective in alleviating intestinal inflammation in weanling piglets, consistent with several earlier reports(Reference Jang, Sun and Chen8,Reference Hou, Zhang and Ahmad29,Reference Gessner, Fiesel and Most30) .
Intestinal microbiota is critical in host intestinal function and immune development(Reference Ren, Chen and Yin31). We found that HP enriched Firmicutes and diminished Bacteroidetes in colonic digesta of piglets in the present study. Consistently, Jang et al. reported that oral supplementation of polyphenols increased the relative abundance of Lactobacillus, which belongs to Firmicutes(Reference Jang, Sun and Chen8). As a main contributor of SCFA, Bacteroidetes promote the balance of intestinal microbiota and play an important role in a variety of major metabolic activities involving fermentation of carbohydrates and utilisation of nitrogenous substances(Reference Zhang, Yu and Yang32). Lower abundance of Bacteroidetes in the colon suggested that HP might change bacterial fermentation.
At the genus level, LPS decreased colonic Prevotella_9 abundance, while HP increased caecal Prevotella_9 abundance, consistent with an earlier study by Fiesel et al. (Reference Fiesel, Gessner and Most7). Prevotella belongs to the family Prevotellaceae and phylum Bacteroidetes. Prevotella is a major dietary fibre fermenter and SCFA producer in the gut of pigs(Reference Zhou, Fang and Sun33,Reference Heinritz, Weiss and Eklund34) . In addition, Lactobacillus was considered beneficial for intestinal function(Reference Gibson, Beaumont, Leeds and Rowland35). Consistent with a previous study, we showed that HP increased Lactobacillus in the caecum and colon, suggesting that HP may play an important role in maintaining gut health by modulating gut microbiota composition(Reference Fiesel, Gessner and Most7).
SCFA, a group of saturated aliphatic organic acids that are comprised of no more than six hydrocarbons, are produced by fermentation of polysaccharides by anaerobic intestinal microbiota(Reference Xu, Xu and Ma36). SCFA are transported from the intestinal lumen into the circulation of the host and utilised by various organs, where they play an important role as energy substrates or signalling mediators(Reference Den Besten, Van Eunen and Groen37). Growing evidence has demonstrated that SCFA suppress the generation of proinflammatory cytokines and alleviate various inflammatory disorders(Reference Kobayashi, Mikami and Kimura38). SCFA also play a crucial role as energy sources for intestinal epithelial cells and certain bacterial species and thus improve gut function(Reference Trompette, Gollwitzer and Yadava39). In the present study, dietary HP increased the concentrations of caecal valerate and colonic acetate, propionate and isovalerate in the piglets following LPS challenge, which may help contribute to the improvement of intestinal integrity and a reduction in proinflammatory cytokine gene expression. It is noted that an increase in SCFA production is consistent with an enrichment in Prevotellaceae in response to HP supplementation.
In summary, dietary HP supplementation enhances intestinal disaccharidase activities, barrier function, the Prevotella abundance and SCFA production in LPS-challenged pigs, which may collectively help explain the effect of HP on the alleviation of intestinal inflammation and injury triggered by LPS.
Acknowledgements
This work was financially supported by the Natural Science Foundation of China (grant number 31802076), the Department of Education of Hubei Province (grant number Q20181803) and the Open Project of Hubei Key Laboratory of Animal Nutrition and Feed Science (grant number 201907).
The authors’ contributions were as follows: Y. L. designed the research; Y. L., H. H., P. H., L. W., L. Z., Q. Q. and C. Y. conducted the research; Y. L., X. W. and X. X. analysed the data; Y. L. and X. X. wrote the paper; Y. L., G. Z. and X. X. edited and revised the manuscript; Y. L. had primary responsibility for final content. All authors read and approved the final manuscript.
The authors declare that that there are no conflicts of interest.