Introduction
California is a major producer of many specialty fruit commodities in the United States, including more than 99% of the nation’s nectarines, plums, prunes, raisins, and table grapes (USDA-NASS 2024). Among those, grapes are the most valued crop in California, with more than US$5.5 billion in farmgate value from 350,000 ha of wine, table, and raisin grapes (CDFA 2024). The state is recognized as the fourth largest wine producing region in the world (CDFA 2024; Wine Institute 2024). Collectively, stone fruits such as peach, nectarine, plum, and prune are grown on 40,000 ha with a value of US$750 million (CDFA 2024; USDA-NASS 2024). The Sacramento Valley and the Northern San Joaquin Valley are the major California production regions for these fruit crops.
California is also the second largest rice producer in the United States, with more than 200,000 ha in production (Galvin et al. Reference Galvin, Inci, Mesgaran, Brim-DeForest and Al-Khatib2022), which contributes more than US$1 billion to the economy and 25,000 rice-related jobs in the state (CDFA 2024). The primary rice production area is based in the Sacramento and Northern San Joaquin valleys and typically is water-seeded and grown in continuously flooded conditions during the growing season (UCANR 2023).
Weed competition can dramatically reduce rice yields (Hill et al. Reference Hill, Williams, Mutters and Greer2006), and unmanaged weeds also cause harvest difficulties, host pests and diseases, and increase the weed seedbank (Strand Reference Strand2013). Alongside cultural management methods such as planting certified weed-free rice seed, using a high seeding rate, and continuous water management, herbicides are crucial for weed management in rice fields (Inci and Al-Khatib Reference Inci and Al-Khatib2024). Once rice fields are flooded, herbicides are generally applied on the day of seeding or before the 2-leaf rice growth stage. Most California rice growers follow up with at least one postemergence herbicide application during the season, usually around the mid-tillering stage of rice. That herbicide application usually occurs in May and June, depending on the planting date, rice variety, and environmental conditions (UCANR 2023).
During May and June, grapevine growth stages range from bloom to veraison (Bettiga Reference Bettiga2013). Also currently, many varieties of stone fruits are at a growth stage when the endocarp (pit) hardening process begins, and the fruit size increases (LaRue and Johnson Reference LaRue and Johnson1989). In the Sacramento Valley, the growth stages of these sensitive fruit crops coincide with the mid-tillering stage in rice, when herbicides are applied (Bettiga Reference Bettiga2013; LaRue and Johnson Reference LaRue and Johnson1989), which increases the risk of off-target damage to these crops.
Herbicide drift is the physical movement of spray droplets through the air at the time of application or soon thereafter to any site other than the intended target (UCANR 2024). Under most circumstances, off-target herbicide exposure occurs at rates from less than 1/100× up to 1/33× of the field application rate of the herbicide (Galla et al. Reference Galla, Hanson and Al-Khatib2019). Significant drift events are most frequently associated with relatively high air temperature and wind speed, low relative humidity, small spray droplet size, and relatively short distances to nearby nontarget crops (Whithaus Reference Whithaus2016). The concerns about rice herbicide drift to off-target crops in the Sacramento Valley have been increasing among growers, crop consultants, and researchers (UCANR 2023).
Florpyrauxifen-benzyl (CAS: 1390661-72-9) is a synthetic auxin-type herbicide (categorized as a Group 4 herbicide by the Herbicide Resistance Action Committee and Weed Science Society of America) and was recently registered (EPA Registration 62719-743) for use on rice in California. Florpyrauxifen-benzyl can provide selective control of grasses, as well as good control of sedges and broadleaf weeds, which is novel among herbicides in this class (Miller and Norsworthy Reference Miller and Norsworthy2018). Triclopyr (CAS: 55335-06-3) is also widely used to control sedges and broadleaf weeds in rice fields. Triclopyr is a pyridyloxy-carboxylate auxin-type herbicide commercially available as in triethylamine salt and butoxyethyl ester formulations. When synthetic-auxin herbicides are applied to susceptible plants, growth abnormalities, leaf epinasty, tissue swelling, stem curling, chloroplast damage, membrane and vascular system damage, wilting, and necrosis are commonly observed, which ultimately leads to plant death (Grossmann Reference Grossmann2010).
Synthetic auxins are known for their off-target injuries to vegetables, fruit and nut trees, field and forage crops, ornamentals, and vines (Egan et al. Reference Egan, Barlow and Mortensen2014; Haring et al. Reference Haring, Ou, Al-Khatib and Hanson2022; Inci et al. Reference Inci, Hanson and Al-Khatib2024; Warmund et al. Reference Warmund, Ellersieck and Smeda2022). In regions like the Sacramento Valley with complex cropping systems, it is important to understand the relative sensitivity of crops to simulated drift rates of florpyrauxifen-benzyl, particularly considering the economic impact of California grapevine and wine industries. To steward florpyrauxifen-benzyl around the time of its initial registration in the state, this research was conducted to compare the onset of foliar symptoms from simulated florpyrauxifen-benzyl and triclopyr drift rates onto grapevine, peach, and plum. Additionally, florpyrauxifen-benzyl at simulated drift rates was evaluated on stone fruit and grape, and grape yield was determined in response to florpyrauxifen-benzyl. Triclopyr was included in the grape experiments to allow comparison of florpyrauxifen-benzyl against a grower standard herbicide with the same mode of action.
Materials and Methods
Study Sites
Three simulated non-target drift experiments were conducted in 2020 and 2021 in newly planted orchards of peach (38.538470°N, 121.794836°W) and plum (38.539303°N, 121.794661°W) at the UC-Davis Plant Sciences Field Facility; and in an established wine grape vineyard (38.525301°N, 121.788259°W) at the UC-Davis Department of Viticulture and Enology Tyree Vineyard near Davis, CA. The orchards were established in March 2020 with ‘Coralstar’ peach on ‘Krymsk 86’ rootstock and ‘French Improved’ prune on ‘Krymsk 86’ rootstock. All peach and plum trees were planted with 6-m intrarow spacing and 4.2 m between rows. The vineyard was established in 1998 with a bilateral double-cordon-trained ‘Grenache’ wine grape planted with 1.8-m intrarow spacing and 3.6 m between rows.
The soil in the orchard location was a Yolo silt loam with the following composition: NO3–N 57 ppm; Olsen-P 26 ppm; K 351 ppm; Na 21 ppm; Ca 8 meq 100 g–1; Mg 10 meq 100 g–1; cation exchange capacity 19 meq 100 g–1; organic matter 2.7%; and pH 6.7. In the vineyard the soil was a Yolo silt loam with the following composition: NO3–N 23 ppm; Olsen-P 12 ppm; K 288 ppm; Na 12 ppm; Ca 11 meq 100 g–1; Mg 9 meq 100 g–1; cation exchange capacity 21 meq 100 g–1; organic matter 2.5%; and pH 7.1. Soil compositions were determined by the UC-Davis Analytical Laboratory, in Davis, CA. Standard commercial practices were implemented for all trees and grapevines to avoid disease and insect infestations (Bettiga Reference Bettiga2013; Buchner Reference Buchner2012; Strand Reference Strand1999). In all experiments, weeds in the interrows were mowed, and intrarow strips were treated with a mixture of rimsulfuron at 70 g ai ha–1, indaziflam at 50 g ai ha–1, oxyfluorfen at 560 g ai ha–1, and glufosinate-ammonium at 450 g ai ha–1 plus manufacturer-recommended surfactants. Irrigation was applied to all crops through a single-line drip irrigation system with emitters spaced every 30 cm.
Herbicide Applications
Florpyrauxifen-benzyl (Loyant® CA, 25 g ai L–1; Corteva Agriscience, Indianapolis, IN) treatments were applied on June 9, 2020, in the peach and plum orchards. Florpyrauxifen-benzyl and triclopyr (Grandstand™ CA, 359 g ae L–1; Corteva Agriscience) treatments were applied on June 11, 2020, in the vineyard experiment. In all experiments, florpyrauxifen-benzyl was applied to simulate drift rates of 1/200× (0.5% drift), 1/100× (1% drift), 1/33× (3% drift), and 1/10× (10% drift) of the use rate of 29.4 g ai ha–1 on rice; the vineyard experiment included triclopyr at three drift rates of 1/200×, 1/100×, and 1/33× of the use rate of 420.3 g ae ha–1 on rice (Galla et al. Reference Galla, Hanson and Al-Khatib2019). Nontreated control (NTC) plots were also included for comparison in each experiment. The florpyrauxifen-benzyl spray mixtures included methylated seed oil (Super Spread® MSO; Wilbur-Ellis, Fresno, CA) at 584 ml ha–1 and triclopyr spray mixtures included crop oil concentrate (Mor-Act® COC; Wilbur-Ellis) at 1% v v–1.
All herbicide treatments were applied to one side of the tree or vine canopy in one pass (top to bottom for trees and side to side for cordon-trained vines) with a handheld, carbon dioxide–propelled backpack sprayer calibrated to deliver 187 L ha–1 at 206 kPa pressure through XR 8004-VS nozzle tips (TeeJet® Technologies, Glendale Heights, IL). The sprayer boom had two nozzles spaced 50 cm apart, and spray was delivered based on a 3-s pass per tree or vine. Plots were sprayed early in the morning when it was not windy to avoid drift to nearby trees or vines. Environmental conditions at the time of the orchard and vineyard applications were 16 C air temperature, 58% relative humidity (RH), and 0.4 m s–1 wind speed on June 9, 2020, and 15 C air temperature, 60% RH, and 0.5 m s–1 wind speed on June 11, 2020, respectively. No in-season auxin-type herbicides were used to avoid potential confusion with florpyrauxifen-benzyl and triclopyr symptoms and injury.
Studies were repeated on May 31, 2021, with a different set of trees or vines in the same field (1-yr exposure study, where n = 8). In addition, the trees that were treated with florpyrauxifen-benzyl and the vines that were treated with florpyrauxifen-benzyl and triclopyr in 2020 were also retreated in 2021 to evaluate cumulative effects from 2-yr exposure (2-yr exposure study, where n = 4) (Bhatti et al. Reference Bhatti, Al-Khatib, Felsot, Parker and Kadir1995). The 2-yr exposure experiment was not repeated on the trees that were initially treated in the second year of the study. The 2-yr exposure study methodology was similar to the 1-yr exposure study described above. Environmental conditions during second-year applications were 18 C air temperature, 50% RH, and 0.6 m s–1 wind speed. Because the two tree crops were newly planted for this experiment, the grapevine was the only crop with fruit present at the time of herbicide application; berry diameters were 5 to 10 mm, both on June 11, 2020, and May 31, 2021.
Data Collection and Experimental Design
Experiments were arranged in a randomized complete block design with four replicates, where an individual tree or vine was an experimental unit. One-year and 2-yr studies were arranged as separate experiments with a nonadjacent layout in orchards and vineyards. As a buffer, one nontreated tree or three consecutive vines between treated plots was included in the 2020 orchard experiments and in both years in the vineyard.
Trees and vines were observed for visual injury symptoms at 6, 12, 24, 48, and 72 h after herbicide treatment and 7, 14, 21, 28, 35, 42, and 90 d after treatment (DAT). Symptomology descriptions of the treated foliage were determined according to University of California Integrated Pest Management Herbicide Symptoms guidelines (UCANR 2024). Injury was rated on a scale where 0% = no injury and 100% = death (Al-Khatib et al. Reference Al-Khatib, Parker and Fuerst1992; Bhatti et al. Reference Bhatti, Al-Khatib, Felsot, Parker and Kadir1995; Sciumbato et al. Reference Sciumbato, Chandler, Senseman, Bovey and Smith2004) according to the following scale:
-
0% = Normal size growth; green pigmentation of all leaves; identical appearance to NTC.
-
1%–4% = Normal-sized leaves; less than 5% of the leaves have only one discernible chlorotic spot; overall canopy has faint but indistinct symptoms.
-
5%–9% = Slight reduction in leaf size; two to five diffuse chlorotic spots visible on 5% to 10% of the leaves; up to 5% of leaf curling and crinkling at only young leaves.
-
10%–29% = Reduction in leaf size up to 5%; growth restriction and chlorosis at interveinal tissue; symptoms moderate to severe on 10% to 30% of the leaves; less than 30% of the leaf surface chlorotic; 5% to 10% of necrosis, leaf curling, and crinkling; adjacent chlorotic areas merge and result in necrosis at the interveinal areas; up to 5% shoot curling.
-
30%–49% = Reduction in leaf size from 5% to 10%; shoot tip growth restricted; symptoms severe on 30% to 50% of the leaves; up to 50% of the leaves with chlorosis; from 10% to 25% necrosis; from 5% to 10% moderate to severe curling at shoots and stems.
-
50%–69% = Reduction in leaf size from 10% to 25%; growth significantly restricted; symptoms very severe on 50% to 70% of the leaves; up to 70% of leaf surface chlorotic; from 25% to 50% necrosis, leaf curling, and crinkling; up to 10% stunting and irregular growth at the overall canopy; interveinal tissue-restricted; noticeable stem discoloring with dark red-brown spots up to 15% of the young branches.
-
70%–89% = Growth severely restricted; symptoms very severe on 70% to 90% of the leaves; up to 90% of leaf surface chlorotic; necrosis becomes the primary indicator of plant injury; distinguishable leaf loss; from 10% to 50% stunting at the overall canopy; severe leaf distortion and malformation; obvious stem discoloring with dark red-brown-black spots up to 50% of the branches; terminal bud malformation and death.
-
90%–99% = Almost no development of leaf and interveinal tissues; symptoms extremely severe on all the leaves; epinasty is extreme throughout the leaves, chlorosis and necrosis widespread; more than 50% of stunting; extremely damaged appearance.
-
100% = Plant dead.
Herbicide symptoms on treated grapevines, peach, and plum trees were compared with NTC plants at each observation. Photos were taken from the treated side of the canopy throughout the growing season to ensure consistency in evaluations. Furthermore, trunk diameters from peach and plum trees were measured using a digital caliper with ±25 µm accuracy at approximately 25 cm above the ground before the spring growth started in April (spring data) and at the end of the summer (fall data) approximately 140 DAT (Martín-Palomo et al. Reference Martín-Palomo, Corell, Girón, Andreu, Trigo, López-Moreno, Torrecillas, Centeno, Pérez-López and Moriana2019). Tree growth was expressed through trunk diameter growth as a percent increase based on the following formula:

where
$$Y$$
is the percent increase of trunk diameter,
$${{\rm{{\rm X}}}_f}$$
= trunk diameter in fall,
$${{\rm{{\rm X}}}_s}$$
= trunk diameter in spring. The relative change in trunk diameter of herbicide treated trees was compared with the diameter change in NTC trees.
Grapes were hand-harvested when berries in NTC plots reached ∼20°Brix (1% soluble solids), a common practice for the grapevine industry in the Northern San Joaquin and Sacramento valleys (Bettiga Reference Bettiga2013). Grape clusters were harvested from all treated vines and NTC vines and weighed for total fruit yield and sugar content from a fruit subsample determined with a handheld refractometer (Haring et al. Reference Haring, Ou, Al-Khatib and Hanson2022).
Statistical Analysis
Visible injury ratings and trunk diameter data were subjected to ANOVA using the agricolae (de Mendiburu Reference de Mendiburu2024), emmeans (Searle et al. Reference Searle, Speed and Milliken1980), and dplyr (Wickham et al. Reference Wickham, François, Henry, Müller and Vaughan2023) packages in RStudio software (v. 2024.04.2+764; R Core Team 2024), and Tukey’s honestly significant difference test were used at α = 0.05 to separate means using the multcomp package (Bretz et al. Reference Bretz, Hothorn and Westfall2010) when applicable. Treatment by exposure interactions were analyzed and presented separately due to the different sample numbers (1-yr exposure study n = 8 and 2-yr exposure study n = 4). The herbicide and fractional drift rates were considered fixed factors, while year and replication were considered random factors. We used Type II Wald F-tests with the Kenward-Roger degrees-of-freedom method and Type III with Satterthwaite’s method when the confidence level was 0.95, and the significance level was α = 0.05 for both ANOVA types. Grape yield and °Brix were analyzed with analysis of covariance at α = 0.05 (Kniss and Streibig Reference Kniss and Streibig2018). Visual illustration was generated using the ggplot2 package in RStudio software (v. 3.5.1) when needed (Wickham et al. Reference Wickham, Navarro and Pedersen2024).
Results and Discussion
Because there were no significant interactions between year and treatment (data not shown), the visual symptom data for 2020 and 2021 were combined for presentation (Tables 1, 2, and 3). Generally, florpyrauxifen-benzyl and triclopyr symptoms were apparent on all treated vines (Figures 1 and 2), and florpyrauxifen-benzyl symptoms were apparent on all treated trees (Figures 3 and 4), with symptoms increasing as the herbicide rate increased (Tables 1, and 3). However, the florpyrauxifen-benzyl symptoms were more severe on grapevine than peach and plum, and the most apparent symptoms were observed when the 1/10× florpyrauxifen-benzyl rate was applied. Furthermore, the time to develop florpyrauxifen-benzyl symptoms at all rates was shorter on grapevine than on peach and plum. All crops had slightly more injury after 2 yr of exposure, which may suggest cumulative injury.
Table 1. Grapevine injury following simulated drift rates of florpyrauxifen-benzyl and triclopyr in 2020 and 2021. a, b
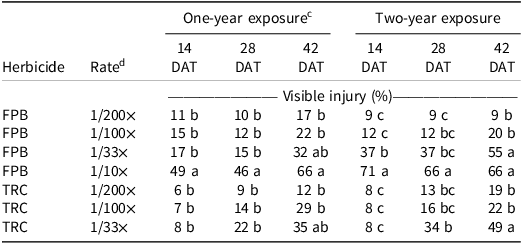
a Abbreviations: DAT, days after treatment; FPB, florpyrauxifen-benzyl; TRC, triclopyr.
b Means within a column not followed by the same letter are significantly different at P ≤ 0.05 using Tukey’s honestly significant difference test.
c One-year exposure: Vines were treated in 2020, and the study was repeated on different vines in 2021, where sample size n = 8. Two-year exposure: The vines that were treated in 2020 were retreated in 2021, where sample size n = 4.
d Florpyrauxifen-benzyl rate is expressed as a fraction of the rice use rate, 29.4 g ai ha–1. Triclopyr rate is expressed as a fraction of the rice use rate, 420.3 g ae ha–1.
Table 2. Peach injury following simulated drift rates of florpyrauxifen-benzyl in 2020 and 2021. a, b
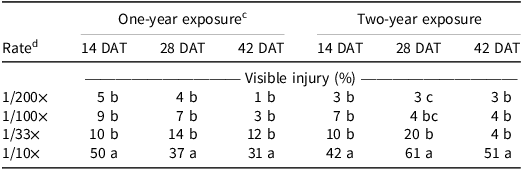
a Abbreviation: DAT, days after treatment.
b Means within a column not followed by the same letter are significantly different at P ≤ 0.05 using Tukey’s honestly significant difference test.
c One-year exposure: Trees were treated in 2020, and the study was repeated on different trees in 2021, where sample size n = 8. Two-year exposure: The trees that were treated in 2020 were retreated in 2021, where sample size n = 4.
d Florpyrauxifen-benzyl rate is expressed as a fraction of the rice use rate, 29.4 g ai ha–1.
Table 3. Plum injury following simulated drift rates of florpyrauxifen-benzyl in 2020 and 2021. a, b
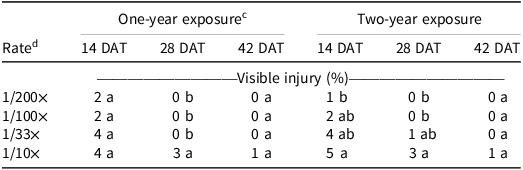
a Abbreviation: DAT, days after treatment.
b Means within a column not followed by the same letter are significantly different at P ≤ 0.05 using Tukey’s honestly significant difference test.
c One-year exposure: Trees were treated in 2020, and the study was repeated on different trees in 2021, where sample size n = 8. Two-year exposure: The trees that were treated in 2020 were retreated in 2021, where sample size n = 4.
d Florpyrauxifen-benzyl rate is expressed as a fraction of the rice use rate, 29.4 g ai ha–1.
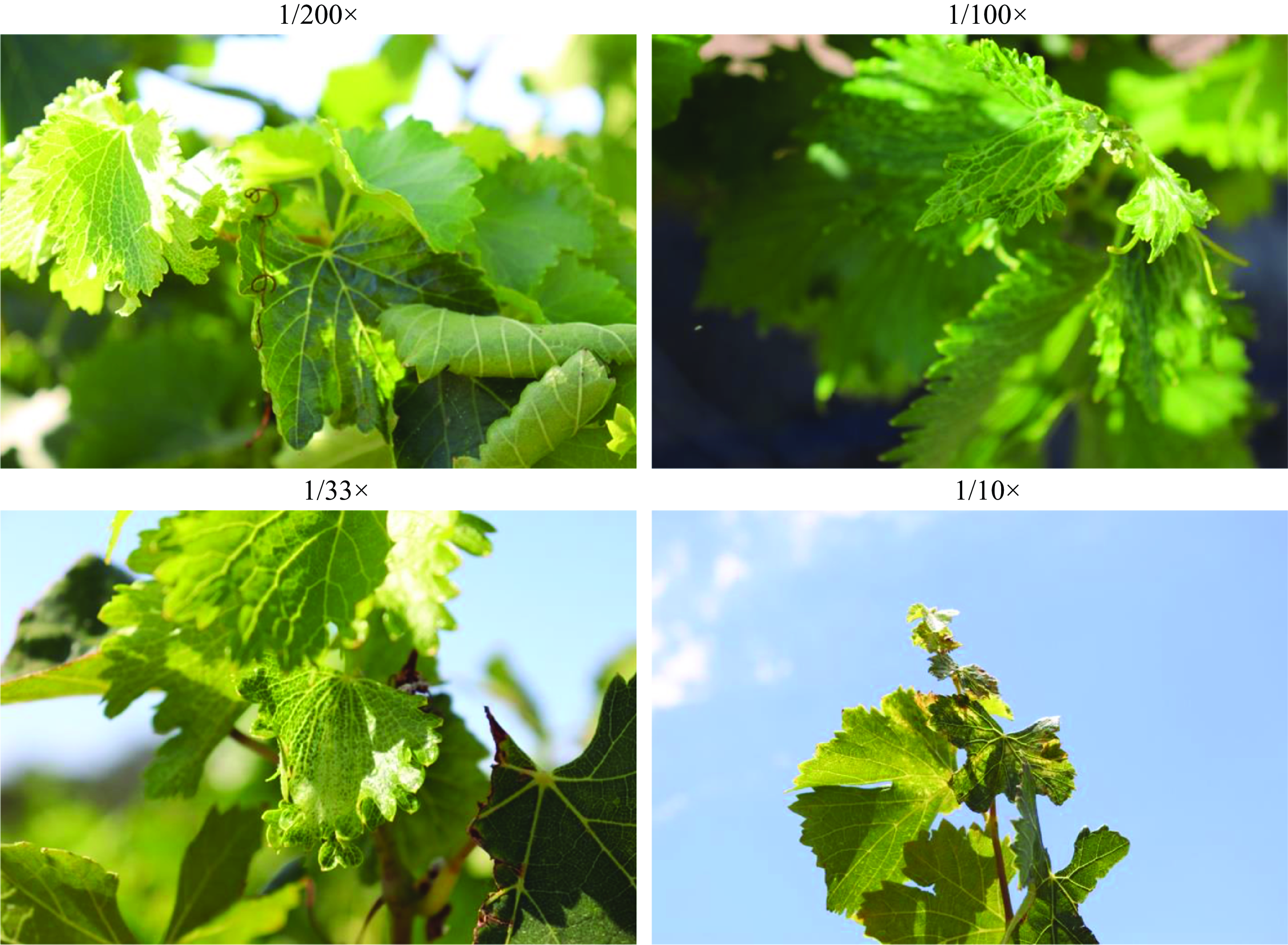
Figure 1. Chlorosis, epinasty, leaf crinkling, necrosis, and shoot curling symptoms on grapevine 28 d after treatment with florpyrauxifen-benzyl at 1/200×, 1/100×, 1/33×, and 1/10× simulated drift rates. The use rate of florpyrauxifen-benzyl on rice is 29.4 g ai ha–1. Photos were taken June 28, 2021, in the 2-yr exposure study.
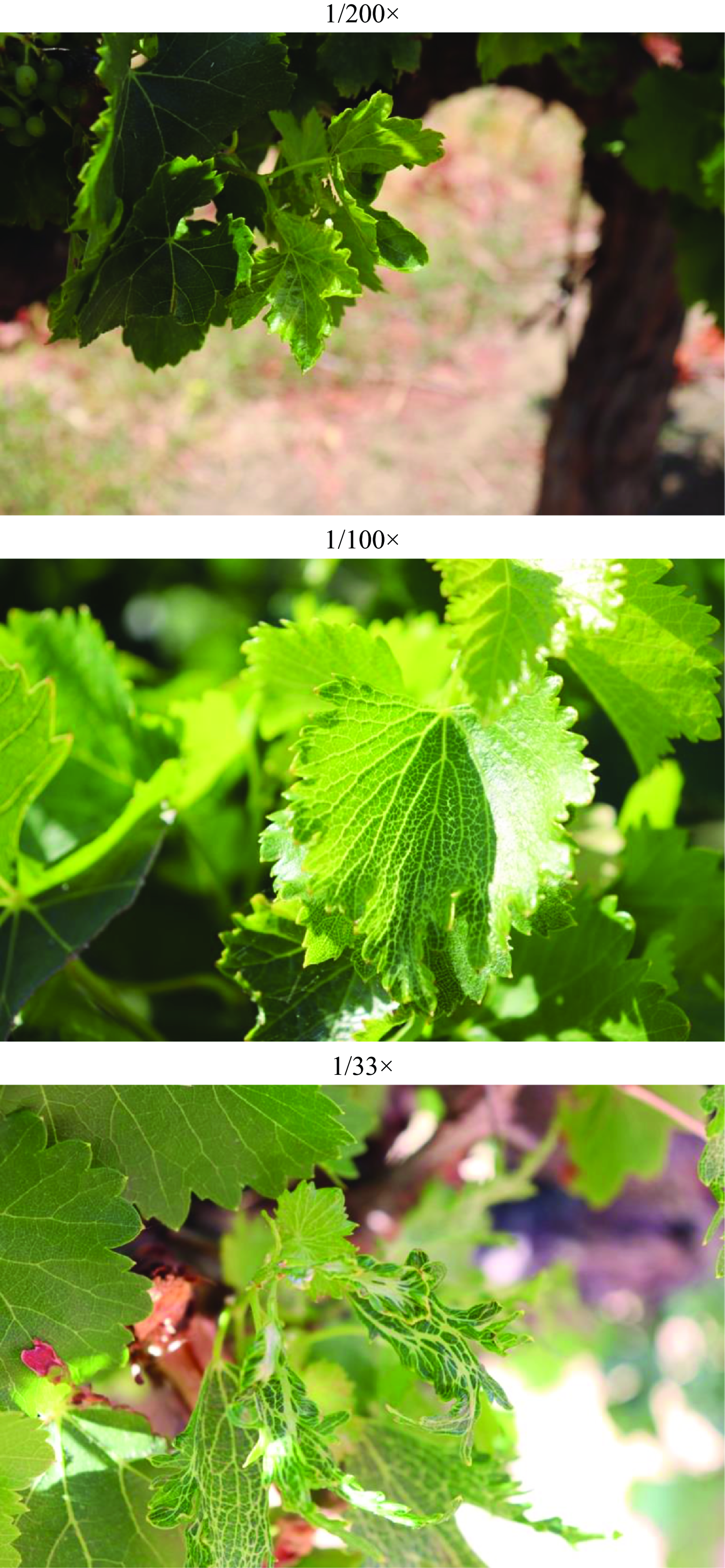
Figure 2. Chlorosis, epinasty, leaf crinkling, leaf narrowing and twisting symptoms 28 d after treatment with triclopyr at 1/200×, 1/100×, and 1/33× simulated drift rates. The use of triclopyr on rice is 420.3 g ae ha–1. Photos were taken June 28, 2021, in the 2-yr exposure study.

Figure 3. Chlorosis, epinasty, and necrosis symptoms on peach 28 d after treatment with florpyrauxifen-benzyl at 1/200×, 1/100×, 1/33×, and 1/10× simulated drift rates. The use rate of florpyrauxifen-benzyl on rice is 29.4 g ai ha–1. Photos were taken June 28, 2021, in the 2-yr exposure study.

Figure 4. Chlorosis, epinasty, and stem curling symptoms on plum 28 d after treatment with florpyrauxifen-benzyl at 1/200×, 1/100×, 1/33×, and 1/10× simulated drift rates. The use rate of florpyrauxifen-benzyl on rice is 29.4 g ai ha–1. Photos were taken June 28, 2021, in the 2-yr exposure study.
Florpyrauxifen-benzyl injury symptoms were observed 3 DAT on grapevine and gradually peaked by 42 DAT. Grapevine symptoms were noticeable on both the treated and nontreated sides of the vines, and the developing leaves and shoots exhibited more symptoms than fully developed leaves and shoots. The injury symptoms on both sides of the canopy might be due to the cordon training that kept the vine canopy relatively narrower than the tree crops, which allowed more spray solution to reach the nontreated side of the plant. Grapevine symptoms included chlorosis, chlorotic spot, epinasty, leaf curling, leaf narrowing, leaf crinkling, necrosis, necrotic spots, shoot curling, and twisting (Figure 1). Initial chlorosis symptoms turned to necrosis within 7 to 14 DAT, and eventually to necrotic spots and holes in the leaf. Chlorosis and epinasty, especially in the interveinal areas, and necrosis were characteristic at the 1/33× and 1/10× rates. Drift from the 1/10× rate of florpyrauxifen-benzyl caused the most severe symptoms to grapevines, including deformation of grape clusters and individual berries—such as irregular color, shape, and size of berries—compared with the NTC vines. Some of the damaged berries dropped later in the season and appeared abnormal or misshapen due to the abortion of individual berries giving the clusters a physically damaged appearance at the 1/10× florpyrauxifen-benzyl rate. Two-year treated grapevine showed more abnormal clusters and reduced foliage growth with necrosis throughout the 2021 season. However, even at this high simulated drift rate, these vines gradually recovered by 90 DAT due to a lack of symptoms on the new leaves, except on vines that had been treated with the 1/10× florpyrauxifen-benzyl rate, for which injury symptoms remained throughout the season (data not shown). Triclopyr injury symptoms were observed at 7 DAT for grapevines and gradually peaked at 42 DAT. In general, grapevine injury symptoms from triclopyr were similar to florpyrauxifen-benzyl injury symptoms at the same rates (Figure 2).
Grapevine visible injury ratings tended to be highest at the 1/10× rate of florpyrauxifen-benzyl throughout the observation period (Table 1). Other florpyrauxifen-benzyl treatments caused similar injury levels to one another, except the 1/33× rate at 42 DAT. Similar to the 1/33× florpyrauxifen-benzyl rating at 42 DAT, triclopyr also caused injury of 35% at a 1/33× drift rate. Our results indicated lower levels of visible injury to grapevines from triclopyr at 1/100× and 1/33× rates compared with previous research (Haring et al. Reference Haring, Ou, Al-Khatib and Hanson2022; Roberto et al. Reference Roberto, Genta, Dalazen and Leles2021). This variation could be the result of the application timing, adjuvant selection, environmental conditions at the time of application, and the maturity of vines.
Grapevines treated with 1/33× and 1/10× florpyrauxifen-benzyl and 1/33× triclopyr rates 2 yr in a row had up to approximately 50% yield reduction (Table 4) compared with the NTC. Yield was 22.1 kg vine–1 in the 1-yr exposure study and 19.3 kg vine–1 in the 2-yr exposure study. However, the grape yield from plots treated with 1/200× and 1/100× drift rates of both florpyrauxifen-benzyl and triclopyr was not different from that of the NTC. Furthermore, grape sugar content tended to increase as florpyrauxifen-benzyl and triclopyr fractional drift rates increased (Table 4), but it was different from the NTC only with the 1/10× rate of florpyrauxifen-benzyl in the 1-yr exposure study, florpyrauxifen-benzyl at the 1/33× and 1/10× rates, and triclopyr at the 1/33× rate in the 2-yr exposure study. The highest florpyrauxifen-benzyl rate increased °Brix up to ∼20% compared with NTC vines that were ∼20°Brix at harvest. The higher sugar content with greater herbicide rates were similar in previous research (Haring et al. Reference Haring, Ou, Al-Khatib and Hanson2022).
Table 4. Grape yield and sugar concentration response to florpyrauxifen-benzyl and triclopyr simulated drift rates. a, b

a Abbreviations: FPB, florpyrauxifen-benzyl; NTC, nontreated control; TRC, triclopyr.
b Means within a column not followed by the same letter are significantly different at P ≤ 0.05 using Tukey’s honestly significant difference test.
c One-year exposure: Vines were treated in 2020, and the study was repeated on different vines in 2021, where sample size n = 8. Two-year exposure: The vines that were treated in 2020 were retreated in 2021, where sample size n = 4.
d Florpyrauxifen-benzyl rate is expressed as a fraction of the rice use rate, 29.4 g ai ha–1. Triclopyr rate is expressed as a fraction of the rice use rate, 420.3 g ae ha–1.
e One degree Brix is 1 g of sucrose in 100 g of solution (1°Brix = 1% sugar).
Peach trees exhibited symptoms of florpyrauxifen-benzyl exposure at 7 DAT, and symptoms generally peaked at 14 DAT (Table 2). The injury was apparent on the treated side of the peach canopy, particularly on developing leaves and terminal buds, and initially appeared as chlorosis and leaf curling, and included stunting at the 1/10× rate (Figure 3). At approximately 14 DAT, leaf curling became more severe, and young shoots also showed curling symptoms. Shoot curling, stunting, and twisting were more apparent at the 1/33× and 1/10× rates than at the lower rates. Peach visible injury ratings were up to 50% at 14 DAT with the 1/10× florpyrauxifen-benzyl drift rate in the 1-yr exposure study (Table 2). In the 2-yr exposure study, florpyrauxifen-benzyl at the 1/10× rate resulted in 61% injury at 28 DAT. However, injury symptoms dissipated through 42 DAT, and peach trees appeared normal at the end of the growing season except for trees that were treated with the 1/10× florpyrauxifen-benzyl rate. Peach trees treated with the 1/10× florpyrauxifen-benzyl rate were stunted throughout the growing season and had noticeable symptoms in the following spring.
Injury symptoms were detectable by 7 DAT to plum, and generally peaked at 14 DAT (Table 3). Plum injury symptoms were distinguishable only on the treated side of the tree, and symptoms were more apparent on developing leaves and branches. In general, injury symptoms to plum from florpyrauxifen-benzyl were less than grapevine and peach (Figure 4). Florpyrauxifen-benzyl injury to plum included chlorosis, leaf curling, necrosis, and stem curling. In addition, minor epinasty symptoms on the tips of developing branches were observed in the following growing season at the 1/10× florpyrauxifen-benzyl rate. Visible injury ratings of plum were less than 6% at all rates for all observations in both 1-yr and 2-yr exposure studies (Table 3). The results showed that plums rapidly recovered from visible injury, even at the highest rate compared to grapevine and peach. Plum trees appeared normal throughout most of the growing season and plum was the least sensitive crop to florpyrauxifen-benzyl in this study.
Tree trunk diameter change over the year showed no significant interactions between herbicide treatment, and exposure to peach or plum trees. In both crops, the relative trunk diameter growth was not statistically different (P > 0.05) compared to the NTC trees (data not shown). This indicates that, despite foliar symptoms, trunk diameter was not affected by simulated florpyrauxifen-benzyl drift.
Overall, grapevine was more sensitive to florpyrauxifen-benzyl than peach or plum. The results regarding visible injury recovery suggest that peach and plum can recover from florpyrauxifen-benzyl drift exposure with limited long-lasting injury. Grapevine foliage can recover from a single exposure of the typical drift rates of florpyrauxifen-benzyl and triclopyr. However, florpyrauxifen-benzyl and triclopyr drift at the 1/33× and greater rates may result in significant yield reduction despite of foliage recovery from the initial injury symptoms. This simulated drift research was conducted using a constant spray volume and variable rates, which is different from actual drift scenarios in which both concentration and volume change as herbicides move off-target. Other researchers have suggested droplet concentration can dramatically affect crop injury (Banks and Schroeder Reference Banks and Schroeder2002); however, understanding the relative sensitivity of the three fruit crops species grown in proximity to California rice fields is highly relevant to stewardship of florpyrauxifen-benzyl in the Sacramento Valley.
Practical Implications
The differences between grapevine, peach, and plum responses to simulated florpyrauxifen-benzyl rates are not surprising because the absorption, translocation, and metabolism of herbicides can vary among plant species (Al-Khatib et al. Reference Al-Khatib, Parker and Fuerst1992). In addition, severe symptoms on developing leaves is common since young leaves are metabolically more active and absorb more herbicide than fully developed leaves (Al-Khatib et al. Reference Al-Khatib, Parker and Fuerst1992). Realistic herbicide drift rates under field conditions generally range from below 1/100× up to 1/33× of field use rates (Al-Khatib and Peterson Reference Al-Khatib and Peterson1999); the 1/10× florpyrauxifen rate in this study was added to simulate a worst-case scenario, considering consecutive drift events in a short interval of time, an accidental herbicide application, or herbicide-contaminated tank, events that are much less common than typical drift situations.
Due to its selective grass activity and good control of broadleaves and sedges, florpyrauxifen-benzyl is expected to be widely used in rice fields (Inci Reference Inci2024). California growers are familiar with management programs for triclopyr, another auxin-type herbicide that has been registered for use on rice for many years. This research suggests that peach and plum trees can be visibly injured by florpyrauxifen-benzyl drift but can recover; however, grapevines are more sensitive and can incur significant damage if exposure rates are sufficient. Because grapevines are more sensitive to florpyrauxifen-benzyl than the tree crops tested here, extra precautions should be considered if there are nearby vineyards. Currently, spray drift advisories for florpyrauxifen-benzyl applications allow only ground applications, whereas triclopyr is allowed to be aerially applied (Corteva Agriscience 2024). Likewise, allowable wind speed at the time of application for florpyrauxifen-benzyl is also more restrictive than triclopyr ground applications, which helps to reduce the risk of significant levels of drift from florpyrauxifen-benzyl applications.
Acknowledgments
We gratefully acknowledge the efforts of Seth Watkins and University of California weed science graduate students for their assistance with the fieldwork.
Funding
This research was funded by grants RR20-7 and RR21-9 from the California Rice Research Board, by the Melvin D. Androus Endowment, a UC-Davis Horticulture and Agronomy Graduate Student Award, and a Henry A. Jastro–Shields Graduate Research Award.
Competing Interests
The authors declare none.