INTRODUCTION
Over a third of the human population is currently infected by one or more species of parasitic helminth (Hotez et al. Reference Hotez, Brindley, Bethony, King, Pearce and Jacobson2008). Chronically-infected hosts must strike a balance between anti-parasite protective responses and limiting immune-mediated pathology (Hoffmann et al. Reference Hoffmann, Wynn and Dunne2002), whilst parasites have developed strategies to prolong intra-host survival and fecundity. Throughout their co-evolutionary history these forces, as often in concert as in opposition, have driven diversity in both parasites (Maizels et al. Reference Maizels, Gomez-Escobar, Gregory, Murray and Zang2001) and the genetics of the host immune response (Fumagalli et al. Reference Fumagalli, Pozzoli, Cagliani, Comi, Riva, Clerici, Bresolin and Sironi2009; Maizels, Reference Maizels2009), the latter especially evident in the human population. Experimental models are invaluable in mechanistic studies of helminth-induced immune responses in vivo; however, reductionist laboratory models inevitably seek to minimize variation in the host, the context of infection and polymorphism in the parasite itself. Moreover, integral differences in the immune systems of different host species (Mestas and Hughes, Reference Mestas and Hughes2004) and the inability of many anthropophilic helminths to naturally infect laboratory animals mean that current experimental models do not reflect the complexity of human helminth immunobiology. Most importantly, models cannot easily replicate the major sources of human immune heterogeneity such as parasite transmission dynamics (Mutapi et al. Reference Mutapi, Ndhlovu, Hagan and Woolhouse1997), distribution of intermediate hosts (Gryseels et al. Reference Gryseels, Polman, Clerinx and Kestens2006), distinct intra-population exposure patterns (Rudge et al. Reference Rudge, Stothard, Basanez, Mgeni, Khamis, Khamis and Rollinson2008), therapeutic interventions (Mutapi et al. Reference Mutapi, Burchmore, Mduluza, Foucher, Harcus, Nicoll, Midzi, Turner and Maizels2005) and concurrent or previous infections with other pathogen species (Correa-Oliveira et al. Reference Correa-Oliveira, Golgher, Oliveira, Carvalho, Massara, Caldas, Colley and Gazzinelli2002).
This review highlights differences in the human acquired immune response to a variety of nematode and trematode infections of public health importance and explores some of the factors contributing to these variations, particularly differences arising from helminth life-history traits.
COMMON FEATURES OF THE ACQUIRED IMMUNE RESPONSE
The most prevalent human helminthiases are caused by nematode species including filarial worms (Brugia malayi, Onchocerca volvulus and Wuchereria bancrofti) and soil-transmitted helminths (Hotez et al. Reference Hotez, Brindley, Bethony, King, Pearce and Jacobson2008): Ascaris lumbricoides, Trichuris trichiura, hookworm (Ancyclostoma duodenale and Necator americanus), Strongyloides spp., and Enterobius vermicularis. Of the trematodes, Schistosoma spp. are of greatest public health importance, with the 3 predominant species (S. haematobium, S. japonicum and S. mansoni) accounting for over 200 million current infections worldwide (Gryseels et al. Reference Gryseels, Polman, Clerinx and Kestens2006). In addition, and beyond the scope of this article, there are many important human cestode parasites which have been expertly discussed elsewhere (Zhang et al. Reference Zhang, Ross and McManus2008).
The principal cellular mediators of human-helminth interactions are CD4+ T cells, which can differentiate into alternative lineages once activated; Th1, Th2, Th17 and T regulatory (Treg), as summarized in Fig. 1. Selective differentiation is first driven by innate antigen-presenting cells (APC) but, as responses mature during the course of chronic infection, cytokine-mediated cross-regulation between T cell subsets becomes increasingly important. Differences in acquired immune responses to helminth infection can arise via heterogeneity in how parasites initially interact with host cells, polarize local and systemic responses and/or modulate effector responses in chronic infection. There are two principal immunological features believed to be common amongst helminth infections. (1) Polarization of CD4+ T cells towards a Th2 phenotype. In humans, this phenotype is associated with production of interleukins (IL-)4, 5, 9, 10 and 13 (Turner et al. Reference Turner, Faulkner, Kamgno, Cormont, Van Snick, Else, Grencis, Behnke, Boussinesq and Bradley2003; Jackson et al. Reference Jackson, Turner, Rentoul, Faulkner, Behnke, Hoyle, Grencis, Else, Kamgno, Boussinesq and Bradley2004b; Quinnell et al. Reference Quinnell, Pritchard, Raiko, Brown and Shaw2004), secretion of IgE and IgG4 isotypes by plasma cells (Hagan et al. Reference Hagan, Blumenthal, Dunn, Simpson and Wilkins1991) and activation of downstream effector cells such as eosinophils (reviewed by (Klion and Nutman, Reference Klion and Nutman2004)). (2) Immunosuppression of both worm-specific and generalized immune responses. Inducible mechanisms include secretion of suppressive cytokines, such as IL-10 and TGF-β, and expansion of regulatory cell populations, particularly Tregs (Doetze et al. Reference Doetze, Satoguina, Burchard, Rau, Loliger, Fleischer and Hoerauf2000; Watanabe et al. Reference Watanabe, Mwinzi, Black, Muok, Karanja, Secor and Colley2007; Babu et al. Reference Babu, Bhat, Kumar, Lipira, Kumar, Karthick, Kumaraswami and Nutman2009). The progression of host responses from effector Th2 to a so-called ‘modified Th2’ phenotype associated with elevated levels of Treg-associated molecules and reduced Th2 effector cytokine responses (Maizels and Yazdanbakhsh, Reference Maizels and Yazdanbakhsh2003), suggests that both features play a functional, and potentially cross-regulatory, role in helminth immunobiology.

Fig. 1. Summary of the major CD4+ T cell differentiation pathways following activation in the periphery. Cytokines and transcription factors involved in T cell polarization are shown in boxes and within cells respectively. Effector cell types and cytokines associated with each CD4+ T cell phenotype are given adjacent to relevant cells. Th0 – naïve T cell, * RORγt – murine transcription factor, the human orthologue is RORC2. Figure adapted from Deenick and Tangyne (Reference Deenick and Tangye2007) and Diaz and Allen (Reference Diaz and Allen2007).
Despite similarities in the immune responses elicited by different helminths, immunological studies often yield contradictory results in different human populations and different species of helminth infection, which are discussed below. To an extent this is unsurprising as expanding research into human helminthiases has inevitably led to a greater appreciation of the intricacies of their immunobiology. The discovery of new cell populations including Treg and Th17 and the more recent description of IL-5 and IL-9 producing CD4+ T cells, which differentiate from Th2 cells in an IL-33 or TGF-β/IL-4-dependent manner respectively, has led to a re-evaluation of the classical Th1-Th2 paradigm (Dardalhon et al. Reference Dardalhon, Awasthi, Kwon, Galileos, Gao, Sobel, Mitsdoerffer, Strom, Elyaman, Ho, Khoury, Oukka and Kuchroo2008; Kurowska-Stolarska et al. Reference Kurowska-Stolarska, Kewin, Murphy, Russo, Stolarski, Garcia, Komai-Koma, Pitman, Li, McKenzie, Teixeira, Liew and Xu2008; Veldhoen et al. Reference Veldhoen, Uyttenhove, Van Snick, Helmby, Westendorf, Buer, Martin, Wilhelm and Stockinger2008). Furthermore the CD4+ T cell axis can be regulated both by effector cytokines from CD4+ T cell populations themselves (IFNγ, IL-4 and IL-21 (Wilson et al. Reference Wilson, Jones, Mwatha, Kimani, Booth, Kariuki, Vennervald, Ouma, Muchiri and Dunne2008; Babu et al. Reference Babu, Bhat, Kumar, Lipira, Kumar, Karthick, Kumaraswami and Nutman2009)) and innate and adaptive non-T cell populations, which both present antigen to activate T cells and contribute significant levels of these same cytokines. Helminth-induced alternatively activated macrophages exhibit particular plasticity in this respect (Jenkins and Allen, Reference Jenkins and Allen2010). Studies showing that Th2 IL-4 and IL-5 responses can be dissociated in human nematode (Sartono et al. Reference Sartono, Kruize, Kurniawan, Maizels and Yazdanbakhsh1997) and trematode (Scott et al. Reference Scott, Turner, Mutapi, Woolhouse, Chandiwana, Mduluza, Ndhlovu and Hagan2000) infections indicate that even established immune phenotypes involve heterogeneous effector responses to helminth infection. As more data become available on human anti-helminth responses, particularly in terms of the more recently described cytokines (e.g. IL-17, IL-21, IL-23, IL-33), comparative studies between helminth groups will be better able to identify similarities and differences in these responses.
HETEROGENEITY IN PROTECTIVE IMMUNE RESPONSES
Protective immunity in human helminthiases encompasses a range of overlapping mechanisms: (a) complete elimination of parasites (sterile immunity), (b) resistance to de novo infection (non-sterile immunity, also called ‘concomitant immunity’) and (c) reduction of immunopathology (tolerance). The former two processes can be grouped as anti-parasite immunity, whilst the latter involves immune-mediated regulation of pathological effector responses. The high prevalence of chronic helminth infection in endemic populations suggests that sterile immunity is rarely generated (Hotez et al. Reference Hotez, Brindley, Bethony, King, Pearce and Jacobson2008). However, the decline in infection intensity at an earlier age in populations with high infection intensity (Woolhouse, Reference Woolhouse1998) and more rapid development of resistance to re-infection post-treatment in individuals with long-term exposure to infection (Black et al. Reference Black, Mwinzi, Muok, Abudho, Fitzsimmons, Dunne, Karanja, Secor and Colley2010) indicates that non-sterile immunity, though slow to develop, does occur with cumulative exposure. Conversely, since the majority of helminth infections are asymptomatic, it is clear that tolerance of low-level infection can be readily elicited to limit immunopathology (Dessein et al. Reference Dessein, Kouriba, Eboumbou, Dessein, Argiro, Marquet, Elwali, Rodrigues, Li, Doumbo and Chevillard2004). The balance between anti-parasite and anti-pathology responses is inevitably shaped by the specific immunopathogenesis of different helminth species. Contrary to phylogenetic distinctions between nematodes and trematodes, one of the main functional delineations between helminth infections is that between species where adult worms reside in the gastrointestinal (GI) tract and those that inhabit host tissues.
Anti-parasite immune responses
Expulsion of GI nematodes is dependent on highly polarised Th2 responses (reviewed by (Jackson et al. Reference Jackson, Friberg, Little and Bradley2009; Jenkins and Allen, Reference Jenkins and Allen2010)) and elevated titres of systemic and parasite-specific Th2 cytokines are negatively associated with infection intensity in untreated populations (Turner et al. Reference Turner, Faulkner, Kamgno, Cormont, Van Snick, Else, Grencis, Behnke, Boussinesq and Bradley2003). Th2-induced smooth muscle hypercontractility and mucus secretion by goblet cells are known to facilitate clearance of murine GI nematode infections (Finkelman et al. Reference Finkelman, Shea-Donohue, Morris, Gildea, Strait, Madden, Schopf and Urban2004), but these physical means of worm expulsion are absent in the tissues. The immunological relevance of these differences has not been tested in humans.
Resistance to re-infection by GI nematodes post-treatment also tends to be unequivocally Th2-mediated (Jackson et al. Reference Jackson, Turner, Rentoul, Faulkner, Behnke, Hoyle, Grencis, Else, Kamgno, Bradley and Boussinesq2004a,Reference Jackson, Turner, Rentoul, Faulkner, Behnke, Hoyle, Grencis, Else, Kamgno, Boussinesq and Bradleyb; Quinnell et al. Reference Quinnell, Pritchard, Raiko, Brown and Shaw2004). Post-treatment resistance to hookworm and T. trichiura infection is associated with pre-treatment levels of IL-5 (Jackson et al. Reference Jackson, Turner, Rentoul, Faulkner, Behnke, Hoyle, Grencis, Else, Kamgno, Bradley and Boussinesq2004a; Quinnell et al. Reference Quinnell, Pritchard, Raiko, Brown and Shaw2004) and negative associations have also been shown between IL-5/IL-13 and re-infection with A. lumbricoides and T. trichiura (Jackson et al. Reference Jackson, Turner, Rentoul, Faulkner, Behnke, Hoyle, Grencis, Else, Kamgno, Boussinesq and Bradley2004b). However, even between GI nematode species the relationship between Th2 cytokines and resistance to re-infection has been shown to vary according to parasite life-history. For example despite multiple shared risk factors for co-infection with A. lumbricoides and T. trichiura, pre-treatment Th2 cytokine titres were only associated with post-treatment resistance to the latter (Jackson et al. Reference Jackson, Turner, Rentoul, Faulkner, Behnke, Hoyle, Grencis, Else, Kamgno, Bradley and Boussinesq2004a). Furthermore, parasite-specific IL-10 was found to be an indicator of species-specific susceptibility, being negatively associated with T. trichiura but positively associated with A. lumbricoides egg counts (Jackson et al. Reference Jackson, Turner, Rentoul, Faulkner, Behnke, Hoyle, Grencis, Else, Kamgno, Bradley and Boussinesq2004a).
Th2-type responses are also involved in protective immunity in tissue-dwelling helminths; however, chronically infected individuals tend to mount Th1-Th2 mixed responses (Joseph et al. Reference Joseph, Jones, Kimani, Mwatha, Kamau, Kazibwe, Kemijumbi, Kabatereine, Booth, Kariuki, Ouma, Vennervald and Dunne2004; Mutapi et al. Reference Mutapi, Winborn, Midzi, Taylor, Mduluza and Maizels2007), thought to be involved in limiting infection intensity (Turaga et al. Reference Turaga, Tierney, Bennett, McCarthy, Simonek, Enyong, Moukatte and Lustigman2000). In an O. volvulus-endemic population in Cameroon, despite a predominant Th2 bias, putative immunity correlated with elevated titres of parasite-specific Th1 (IFNγ), Th2 (IL-5) and innate (granulocyte-macrophage colony-stimulating factor (GM-CSF)) effector cytokines (Turaga et al. Reference Turaga, Tierney, Bennett, McCarthy, Simonek, Enyong, Moukatte and Lustigman2000). Similarly, schistosome-specific IFNγ production by peripheral blood mononuclear cells (PBMC) correlates with immunity to schistosomiasis (Viana et al. Reference Viana, Sher, Carvalho, Massara, Eloi-Santos, Pearce, Colley, Gazzinelli and Correa-Oliveira1994). Mixed Th1-Th2 cytokine responses are observed in chronic trichuriasis in the gut but have not been significantly associated with infection intensity (Faulkner et al. Reference Faulkner, Turner, Kamgno, Pion, Boussinesq and Bradley2002).
Antibody-mediated protection from helminths is typically attributed to elevated titres of parasite-specific IgE (Faulkner et al. Reference Faulkner, Turner, Kamgno, Pion, Boussinesq and Bradley2002; Pearce and MacDonald, Reference Pearce and MacDonald2002), whilst a low IgE:IgG4 is associated with susceptibility to schistosome (Hagan et al. Reference Hagan, Blumenthal, Dunn, Simpson and Wilkins1991) and GI infections (Figueiredo et al. Reference Figueiredo, Barreto, Rodrigues, Cooper, Silva, Amorim and Alcantara-Neves2010). However, positive correlations between parasite-specific IgE and other isotypes (Viana et al. Reference Viana, CorreaOliveira, Dossantos, Massara, Colosimo, Colley and Gazzinelli1995) and studies identifying more pronounced associations between infection intensity and non-IgE isotypes (Webster et al. Reference Webster, CorreaOliveira, Gazzinelli, Viana, Fraga, Silveira and Dunne1997) suggest that there is redundancy in antibody-mediated protective immunity. In addition, given the potentially immunopathogenic outcomes of IgE-mediated cellular effector responses (Nutman and Kumaraswami, Reference Nutman and Kumaraswami2001; Cooper et al. Reference Cooper, Chico, Sandoval and Nutman2004), it is unsurprising that propagation of alternate antibody isotypes in chronically infected hosts may be favourable. A variety of studies investigating other antibody isotypes in schistosomiasis provide conflicting evidence for their role in resistance to infection with different species. For example, whilst polyclonal adult worm-specific IgA titres decline with age and infection intensity in S. haematobium endemic areas (Mutapi et al. Reference Mutapi, Ndhlovu, Hagan and Woolhouse1997), the opposite pattern has been observed for S. japonicum-specific IgA in the Philippines (Acosta et al. Reference Acosta, McManus, Aligui, Olveda and Tiu2004). Similarly, whilst the former study found that S. haematobium adult worm-specific IgG1 increased with age and peaked in individuals with low infection intensity (Mutapi et al. Reference Mutapi, Ndhlovu, Hagan and Woolhouse1997), IgG1 titres were significantly positively associated with intensity of S. mansoni infection in Brazil (de Jesus et al. Reference De Jesus, Araujo, Bacellar, Magalhaes, Pearce, Harn, Strand and Carvalho2000) and Kenya (Naus et al. Reference Naus, Kimani, Ouma, Fulford, Webster, Van Dam, Deelder, Butterworth and Dunne1999). The role of IgM in anti-schistosome responses is also controversial as adult worm and egg-specific IgM increase with S. haematobium and S. japonicum infection intensity in some populations (Mutapi et al. Reference Mutapi, Ndhlovu, Hagan and Woolhouse1997; Acosta et al. Reference Acosta, McManus, Aligui, Olveda and Tiu2004), but adult-worm-specific titres were lowest in individuals patently infected with S. mansoni elsewhere (Viana et al. Reference Viana, CorreaOliveira, Dossantos, Massara, Colosimo, Colley and Gazzinelli1995; Caldas et al. Reference Caldas, Correa-Oliveira, Colosimo, Carvalho, Massara, Colley and Gazzinelli2000). Potential sources of variation in protective immunity within and between schistosome-infected populations are discussed in the following sections.
For filarial infections larvae-specific antibodies acquired with age have been shown to confer resistance to re-infection post-treatment (Day et al. Reference Day, Grenfell, Spark, Kazura and Alpers1991b); however, the immunogenic epitopes within the larval proteome could not be identified (Day et al. Reference Day, Gregory and Maizels1991a). Few studies have directly compared schistosome and nematode-specific antibody responses; however, studies to date suggest that differences do exist. W. bancrofti microfilaria and circulating antigen negative individuals were found to have the lowest parasite-specific IgG4:IgE (Jaoko et al. Reference Jaoko, Simonsen, Meyrowitsch, Estambale, Malecela-Lazaro and Michael2006) as has been observed in schistosome studies (Hagan et al. Reference Hagan, Blumenthal, Dunn, Simpson and Wilkins1991). However, unlike in chronic schistosomiasis, the ratios did not differ between communities with high and low intensity infection indicating that relative changes in these isotypes may be less dependent on host exposure history (Jaoko et al. Reference Jaoko, Simonsen, Meyrowitsch, Estambale, Malecela-Lazaro and Michael2006). IgG2 also appears to limit infection intensity when directed against W. bancrofti microfilaria (Jaoko et al. Reference Jaoko, Simonsen, Meyrowitsch, Estambale, Malecela-Lazaro and Michael2006), but is ineffective against schistosome larvae (Demeure et al. Reference Demeure, Rihet, Abel, Ouattara, Bourgois and Dessein1993).
The specific target of antibody responses is particularly important for development of protective immunity, although the majority of field studies focus on crude antigen preparations. Early schistosome studies identified isotype-specific antibody responses to different antigen types within the parasite proteome (Langley et al. Reference Langley, Kariuki, Hammersley, Ouma, Butterworth and Dunne1994), but it was not until the advent of mass spectrometric analysis that parasite peptides recognized by different antibody isotypes could be identified within crude preparations (Mutapi et al. Reference Mutapi, Burchmore, Mduluza, Foucher, Harcus, Nicoll, Midzi, Turner and Maizels2005, Reference Mutapi, Burchmore, Mduluza, Midzi, Turner and Maizels2008). Relative exposure of immunogenic peptides clearly plays a role in cumulative development of protective antibodies. For example, antibodies against the larval surface are associated with protection against W. bancrofti (Day et al. Reference Day, Gregory and Maizels1991a) and antibodies targeting cryptic antigens released by dying adult worms may influence the course of schistosome infection (Woolhouse and Hagan, Reference Woolhouse and Hagan1999). It remains unclear whether there is a single antibody isotype that uniformly protects against helminth infection and it seems likely that redundancy within host antibody responses, variation between parasite species and/or host populations have all contributed to isotype-specific variations observed in the field.
Anti-pathology immune responses
Limiting immunopathology in patent helminth infection is a combination of parasite and host-mediated processes that synergize to limit aberrant immune reactivity and damage to the host. This is necessarily a compromise as dampening immune responses to infection also limits their efficacy at clearing infection (Maizels and Yazdanbakhsh, Reference Maizels and Yazdanbakhsh2003). For tissue-dwelling helminths close association with host tissues throughout their life-cycle means that effector responses to adult worms and migrating larvae in these loci must be tightly regulated (Montenegro et al. Reference Montenegro, Miranda, Mahanty, Abath, Teixeira, Coutinho, Brinkman, Goncalves, Domingues, Domingues, Sher and Wynn1999). In chronic schistosomiasis, for example, Th2-polarized responses account for the majority of host pathologies including hepatic fibrosis (Coutinho et al. Reference Coutinho, Acosta, Wu, McGarvey, Su, Langdon, Jiz, Jarilla, Olveda, Friedman and Kurtis2007) and egg-specific responses promote granuloma formation (ElRidi et al. Reference ElRidi, Ismail, Gaafar and ElDemellawy1997), whilst Th2-mediated damage is mitigated by Th1-type (Henri et al. Reference Henri, Chevillard, Mergani, Paris, Gaudart, Camilla, Dessein, Montero, Elwali, Saeed, Magzoub and Dessein2002; Dessein et al. Reference Dessein, Kouriba, Eboumbou, Dessein, Argiro, Marquet, Elwali, Rodrigues, Li, Doumbo and Chevillard2004) and innate inflammatory cytokines (TNFα and IL-6 (Booth et al. Reference Booth, Mwatha, Joseph, Jones, Kadzo, Ireri, Kazibwe, Kemijumbi, Kariuki, Kimani, Ouma, Kabatereine, Vennervald and Dunne2004a; Wilson et al. Reference Wilson, Jones, Mwatha, Kimani, Booth, Kariuki, Vennervald, Ouma, Muchiri and Dunne2008)). The importance of Tregs in balancing Th1 and Th17 responses in human filarial infections has been recently highlighted by Babu and colleagues who identified a positive correlation between lymphoedema and Th1/Th17 cytokine and Toll-like receptor expression in individuals with low expression of Treg-associated molecules (Babu et al. Reference Babu, Bhat, Kumar, Lipira, Kumar, Karthick, Kumaraswami and Nutman2009).
Ubiquitous exposure to commensal bacteria and food antigens means that antigen-presentation and inflammatory responses are highly regulated in the gut to maintain a physical barrier between the lumen and host tissues (Mayer, Reference Mayer2000). GI nematodes are also able to actively down-regulate effector responses in the gut to maintain this regulatory environment (reviewed by Maizels and Yazdanbakhsh, Reference Maizels and Yazdanbakhsh2003). Both Ascaris spp. and Trichuris spp. infections are also associated with reduced cellular responsiveness to both non-specific agonists and parasite-specific antigens in humans (Figueiredo et al. Reference Figueiredo, Barreto, Rodrigues, Cooper, Silva, Amorim and Alcantara-Neves2010). The rebound in N. americanus-specific IFNγ observed after anti-helminthic treatment to clear infection suggests that Th1 responses may be particularly regulated in chronic hookworm infection (Quinnell et al. Reference Quinnell, Pritchard, Raiko, Brown and Shaw2004).
However, GI nematodes are not homogeneous in the immune responses that they induce and thus pose distinct immunopathological risks. T. trichiura infection induces a mixed Th1-Th2 cytokine profile (Faulkner et al. Reference Faulkner, Turner, Kamgno, Pion, Boussinesq and Bradley2002), whilst Ascaris lumbricoides infection leads to a highly Th2 environment (Cooper et al. Reference Cooper, Chico, Sandoval, Espinel, Guevara, Kennedy, Urban, Griffin and Nutman2000). It has been postulated that murine Trichuris spp. are distinct from other GI infections in specifically up-regulating Th1 cytokines as a means of evading Th2-mediated clearance (refer to review by Else, Reference Else2005); however, fostering a more mixed cytokine response may also limit pathology. IL-10 secretion is highly prevalent in T. trichiura endemic populations with 97% of a Cameroonian cohort secreting parasite-specific IL-10 and older individuals producing the highest titres of non-specific IL-10 (Faulkner et al. Reference Faulkner, Turner, Kamgno, Pion, Boussinesq and Bradley2002), suggesting that systemic immunoregulation coincides with cumulative exposure to infection.
SOURCES OF ACQUIRED IMMUNE HETEROGENEITY
Helminth life history
Helminths undergo complex life cycles leading to both physical and molecular variations during the course of an infection. Table 1 summarizes the key differences in helminth life histories that can introduce heterogeneity in the development of the host immune response to infection. Furthermore, the range of molecules and mechanisms that underlie helminth-mediated immunomodulation have been reviewed elsewhere (Maizels and Yazdanbakhsh, Reference Maizels and Yazdanbakhsh2003; Maizels et al. Reference Maizels, Pearce, Artis, Yazdanbakhsh and Wynn2009) and present a potential source of immune heterogeneity in themselves.
Table 1. Summary of parasite-factors that may influence the host acquired immune response to helminth infection (Anderson and May, Reference Anderson and May1992; Maizels et al. Reference Maizels, Bundy, Selkirk, Smith and Anderson1993; Maizels and Kurniawan-Atmadja, Reference Maizels and Kurniawan-Atmadja2002; Gryseels et al. Reference Gryseels, Polman, Clerinx and Kestens2006; Hotez et al. Reference Hotez, Brindley, Bethony, King, Pearce and Jacobson2008).
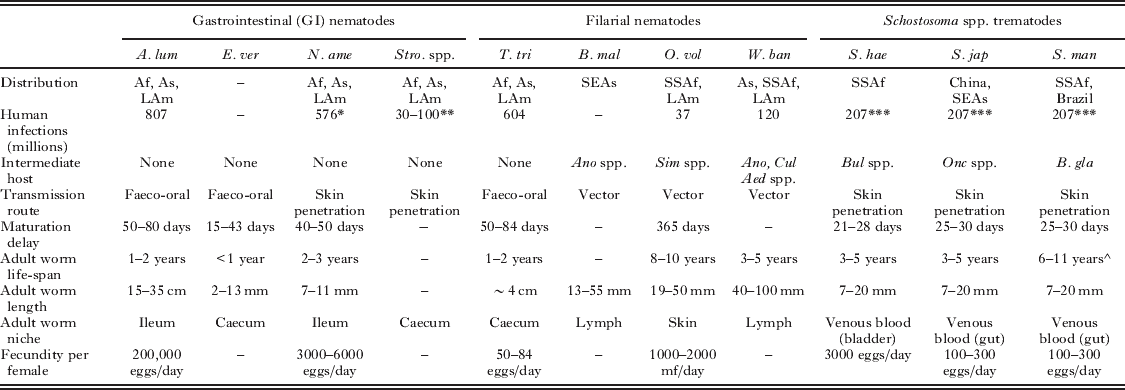
* All hookworm species.
** Strongyloides stercoralis only.
*** All S. haematobium, S. japonicum and S. mansoni infections.
^ Life-span of S. mansoni estimated using maximum likelihood techniques to assess pre- and post-treatment field data (Fulford et al. Reference Fulford, Butterworth, Ouma and Sturrock1995).
Abbreviations: A. lum – Ascaris lumbricoides, E. ver – Enterobis vermicularis, N. ame – Necator americanus, Stro spp. – Strongyloides spp., T. tri – Trichuris trichiura, B. mal – Brugia malayi, O. vol – Onchocerca volvulus, W. ban – Wuchereria bancrofti, S. hae – Schistosoma haematobium, S. jap – Schistosoma japonicum, S. man – Schistosoma mansoni, Af – Africa, As – Asia, LAm – Latin America, SEAs – South East Asia, SSAf – Sub-Saharan Africa, Ano spp. – Anopheles spp. mosquito, Aed spp. – Aedes spp. mosquito, Cul spp. – Culex spp. mosquito, B. gla – Biomphalaria glabrata (aquatic snail), Bul spp. – Bulinus spp. (aquatic snail), mf – microfilariae.
Parasite transmission route
Transmission route impacts upon where and how infection is initially detected by the immune system. Immature helminths face site-specific challenges as they invade their host, for example species that are transmitted through the skin tend to suffer immune attrition in this organ (He et al. Reference He, Salafsky and Ramaswamy2005). Amongst the schistosome species, which invade by active penetration, S. japonicum cercariae migrate most rapidly to the dermis and this species also elicits the most pro-inflammatory response in human skin (He et al. Reference He, Chen and Ramaswamy2002). In contrast S. haematobium and S. mansoni cercariae promote up-regulation of immunoregulatory proteins including IL-10 and IL-1 receptor antagonist (He et al. Reference He, Chen and Ramaswamy2002), highlighting heterogeneity in the effector and regulatory immune mechanisms elicited even by closely-related species.
Arthropod-borne larvae are also exposed to immune attrition in human cutaneous tissue when they enter during blood-feeding by the vector. However, unlike actively penetrating parasites, antigens, enzymes and immunosuppressive molecules in arthropod saliva may skew the host immune response in favour of vector-transmitted larvae (Demeure et al. Reference Demeure, Brahimi, Hacini, Marchand, Peronet, Huerre, St-Mezard, Nicolas, Brey, Delespesse and Mecheri2005). Furthermore, where a single helminth species (such as W. bancrofti) can be transmitted by multiple mosquito species of 4 different genera (Aedes, Anopheles, Culex and Mansonia) (Maizels and Kurniawan-Atmadja, Reference Maizels and Kurniawan-Atmadja2002), there is potential for the human immune response to be differently shaped in each instance. The presence of Wolbachia spp. bacterial endosymbionts in mosquito vectors and almost all filarial helminth species has also been suggested to influence host T-cell responsiveness (Brattig, Reference Brattig2004), but this is yet to be verified.
On a broader scale transmission route can determine distribution of immune-mediated morbidities within host populations. One example is the lower prevalence of Sowda in O. volvulus-infected men relative to women inhabiting the same area, which has been attributed to development of immune tolerance after repeated exposure to O. volvulus vectors during agricultural work (Brattig, Reference Brattig2004; Trpis, Reference Trpis2006).
Following initial infection, larval migration may also contribute to immune heterogeneity between GI nematodes, particularly with respect to anti-pathology immunity. Clinical measures of atopic reactivity suggest that hookworm with lung-migratory stages effectively suppress asthma and wheeze in the lung (Scrivener et al. Reference Scrivener, Yemaneberhan, Zebenigus, Tilahun, Girma, Ali, McElroy, Custovic, Woodcock, Pritchard, Venn and Britton2001; Leonardi-Bee et al. Reference Leonardi-Bee, Pritchard, Britton and Collaboration2006). Therapeutic N. americanus infection is able to dampen inflammation both in the lung and in the gut, where adult worms reside (Falcone and Pritchard, Reference Falcone and Pritchard2005; Croese et al. Reference Croese, O'Neil, Masson, Cooke, Melrose, Pritchard and Speare2006). On the other hand, Trichuris spp. which inhabit the lower intestine and do not migrate through the lung, can dampen inflammatory pathologies in the gut (Reddy and Fried, Reference Reddy and Fried2007) but are less effective at regulating clinical allergy elsewhere (Bager et al. Reference Bager, Arnved, Ronborg, Wohlfahrt, Poulsen, Westergaard, Petersen, Kristensen, Thamsborg, Roepstorff, Kapel and Melbye2010). Interestingly A. lumbricoides infection, which has a lung migratory phase, does not reduce asthmatic responses and is associated with elevated atopy (Flohr et al. Reference Flohr, Quinnell and Britton2009). There are many potential reasons for variation in the effect of GI helminth infection on clinical allergy (reviewed elsewhere (Flohr et al. Reference Flohr, Quinnell and Britton2009)) and conflicting results in existing literature (Leonardi-Bee et al. Reference Leonardi-Bee, Pritchard, Britton and Collaboration2006) and a lack of studies which directly compare GI nematode infections with distinct migratory pathways make it difficult to conclude on the source of observed variations.
Life-cycle stages
Chronically infected individuals are simultaneously exposed to 3 helminth life-cycle stages; infective larvae, adult worms and transmission stage parasites (eggs, immature larvae or microfilariae). Both proteomic (Moreno and Geary, Reference Moreno and Geary2008) and DNA micro-array studies (Jolly et al. Reference Jolly, Chin, Miller, Bahgat, Lim, DeRisi and McKerrow2007; Fitzpatrick et al. Reference Fitzpatrick, Peak, Perally, Chalmers, Barrett, Yoshino, Ivens and Hoffmann2009) have shown that these life-cycle stages are molecularly different and thus elicit stage-specific immune responses that change over time.
In experimental murine studies where the course of infection can be tracked from its acute phase, there is a clear link between the onset of egg production (week 5–6 post-infection) and a Th1-to-Th2 shift in the cellular response to schistosomiasis (Pearce and MacDonald, Reference Pearce and MacDonald2002). Although the Th1-to-Th2 shift is less clear in human infections, defined egg peptides can specifically induce Th2 responses in human basophils, DCs and T cells in vitro (Schramm et al. Reference Schramm, Falcone, Gronow, Haisch, Mamat, Doenhoff, Oliveira, Galle, Dahinden and Haas2003; Everts et al. Reference Everts, Perona-Wright, Smits, Hokke, van der Ham, Fitzsimmons, Doenhoff, van der Bosch, Mohrs, Haas, Mohrs, Yazdanbakhsh and Schramm2009). Th2 polarization by schistosome eggs also induces granuloma formation (ElRidi et al. Reference ElRidi, Ismail, Gaafar and ElDemellawy1997), which facilitates passage of eggs from venous blood into the gut/bladder and subsequent transmission to the environment (Karanja et al. Reference Karanja, Colley, Nahlen, Ouma and Secor1997; Pearce and MacDonald, Reference Pearce and MacDonald2002). Carbohydrate antigens on the schistosome egg surface also promote IgM secretion, which lacks immunological memory (Mutapi et al. Reference Mutapi, Hagan, Woolhouse, Mduluza and Ndhlovu2003), whilst responses associated with protective immunity, such as IgE, IgG1 and IgG3, tend to emerge later in infection in response antigens released from dying adult worms (Woolhouse and Hagan, Reference Woolhouse and Hagan1999).
There are notable distinctions between the type and magnitude of the cytokine response to egg-specific antigens and those directed against adult worm antigens (Joseph et al. Reference Joseph, Jones, Kimani, Mwatha, Kamau, Kazibwe, Kemijumbi, Kabatereine, Booth, Kariuki, Ouma, Vennervald and Dunne2004; Silveira et al. Reference Silveira, Gazzinelli, Alves-Oliveira, Bethony, Gazzinelli, Carvalho-Queiroz, Alvarez, Lima-Silva, Prata, LoVerde and Correa-Oliveira2004). Adult S. mansoni worms tend to elicit a mixed Th1-Th2 cytokine profile (Williams et al. Reference Williams, Montenegro, Domingues, Wynn, Teixeira, Mahanty, Coutinho and Sher1994) and are less effective at stimulating in vitro granuloma formation (IVGF) than egg antigens (ElRidi et al. Reference ElRidi, Ismail, Gaafar and ElDemellawy1997). Cross-sectional data from an S. haematobium endemic cohort suggests that adult worm-specific effector Th2 cytokines increase whilst IL-10 titres decline with age/exposure to infection (Mutapi et al. Reference Mutapi, Winborn, Midzi, Taylor, Mduluza and Maizels2007) and thus effector responses to adult worms are higher in putatively resistant individuals.
In contrast to the Th2 cytokine responses induced by schistosome eggs and infective larvae of most nematodes, schistosome cercariae stimulate Th1/inflammatory mRNA expression in mice and resistance to larval invasion post-vaccination is dependent on IFNγ (Wynn et al. Reference Wynn, Oswald, Eltoum, Caspar, Lowenstein, Lewis, James and Sher1994). Although few studies have directly compared immune responses to the three schistosome life-cycle stages in humans, cercariae-specific IgM, IgG1 and IgG4 titres are higher than those directed against adult worm antigens (Viana et al. Reference Viana, CorreaOliveira, Dossantos, Massara, Colosimo, Colley and Gazzinelli1995). The distinct immune responses elicited by different helminth life-cycle stages underpin the development of so-called concomitant immunity, whereby de novo larval infection is blocked by adult worm-induced immunity but resident adult parasites are tolerated, a theory originally formulated for schistosome infections (Smithers and Terry, Reference Smithers and Terry1967). Studies have found evidence for development of PBMC-mediated resistance to L3 invasion in O. volvulus infection in an endemic area of Cameroon (MacDonald et al. Reference MacDonald, Turaga, Harmon-Brown, Tierney, Bennett, McCarthy, Simonek, Enyong, Moukatte and Lustigman2002) and development of peripheral antibody-dependent immunity to W. bancrofti larvae with age in Papua New Guinea (Day et al. Reference Day, Gregory and Maizels1991a,Reference Day, Grenfell, Spark, Kazura and Alpersb). There is little evidence for development of concomitant immunity to GI nematode infections; however, there is a distinct paucity of data in this area.
In addition to influencing anti-parasite immunity, helminth life-cycle stage contributes to heterogeneity in anti-pathology immune responses. Immunomodulatory processes can be life cycle stage-specific as characterized by distinct cytokine and proliferative responses to their respective antigens (Geiger et al. Reference Geiger, Caldas, Mc Glone, Campi-Azevedo, De Oliveira, Brooker, Diemert, Correa-Oliveira and Bethony2007). In vitro microfilariae are able to impair cytokine expression and maturation of human dendritic cells (DCs) (Semnani et al. Reference Semnani, Sabzevari, Iyer and Nutman2001, Reference Semnani, Law, Kubofcik and Nutman2004) and schistosome egg antigens can inhibit co-stimulatory molecule expression and skew APC cytokine secretion from an inflammatory to regulatory profile (Correale and Farez, Reference Correale and Farez2009), with potential implications for the systemic CD4+ T cell phenotype. A variety of studies have also shown that cercariae excretory/secretory products actively modulate the host immune response during migration and maturation independent of egg or adult-worm-mediated processes (reviewed by Jenkins et al. Reference Jenkins, Hewitson, Jenkins and Mountford2005). However, whilst eggs, cercariae and microfilariae can impair and polarize host responses, the ability of parasites to evade and modulate immune recognition seems to increase as they mature (Nutman and Kumaraswami, Reference Nutman and Kumaraswami2001) and corresponds to large-scale switches in many suites of genes (Jolly et al. Reference Jolly, Chin, Miller, Bahgat, Lim, DeRisi and McKerrow2007) and active secretion of immunomodulatory molecules (Geiger et al. Reference Geiger, Caldas, Mc Glone, Campi-Azevedo, De Oliveira, Brooker, Diemert, Correa-Oliveira and Bethony2007). Thus, despite being exposed to the host immune system for the longest of all life-cycle stages, it is unsurprising that adult worms are so resistant to immune-mediated clearance (Maizels and Yazdanbakhsh, Reference Maizels and Yazdanbakhsh2003), while immature parasites tend to be immunogenic, more readily killed by effector immune responses (Viana et al. Reference Viana, CorreaOliveira, Dossantos, Massara, Colosimo, Colley and Gazzinelli1995; Semnani et al. Reference Semnani, Sabzevari, Iyer and Nutman2001; Medeiros et al. Reference Medeiros, Figueiredo, Almeida, Matos, Araujo, Cruz, Atta, Rego, De Jesus, Taketomi and Carvalho2003) and are responsible for the majority of morbidity in helminth infections (Maizels and Yazdanbakhsh, Reference Maizels and Yazdanbakhsh2003).
Parasite life-span
Unlike microbial infections which multiply exponentially in their hosts, helminths have evolved to invest in immune-evasive mechanisms to prolong intra-host survival and long-term fecundity (Jackson et al. Reference Jackson, Friberg, Little and Bradley2009). Effective immune evasion and suppression by adult worms mean that reactivity to the antigens of live worms is limited (Geiger et al. Reference Geiger, Caldas, Mc Glone, Campi-Azevedo, De Oliveira, Brooker, Diemert, Correa-Oliveira and Bethony2007). Thus, hosts may only experience an immunogenic ‘threshold’ stimulus of parasite antigens once adult worms die (Mutapi et al. Reference Mutapi, Burchmore, Mduluza, Midzi, Turner and Maizels2008) and resistance might be predicted to develop more slowly against long-lived worms than against shorter-lived species. The rapid switch to a protective immune profile following anti-helminthic drug treatment that kills adult worms is consistent with this hypothesis (Mutapi et al. Reference Mutapi, Hagan, Woolhouse, Mduluza and Ndhlovu2003; Watanabe et al. Reference Watanabe, Mwinzi, Black, Muok, Karanja, Secor and Colley2007). If adult helminth life-span does cause a lag in the development of protective immunity then this may contribute to the variation in the age at which peak prevalence (Brooker et al. Reference Brooker, Donnelly and Guyatt2000) and infection intensity (Fig. 2) occurs in host populations exposed to different helminths.

Fig. 2. The relationship between age and infection intensity in natural human helminthiases. (A) Mean W. bancrofti microfilaria (mf) count by age group (n=156, study area: Papua New Guinea, method: microscopy of 2 ml Giemsa-stained blood). Reprinted from the American Journal of Tropical Medicine and Hygiene (Day et al. Reference Day, Grenfell, Spark, Kazura and Alpers1991b), with permission from the managing editor. (B) Mean hookworm (A. duodenale and N. americanus) egg counts per gram faeces (EPG) by age group (n=631, study area: China, method: Kato-Katz thick smear). Reprinted from the Journal of Parasitology (Gandhi et al. Reference Gandhi, Chen, Khoshnood, Xing, Li, Liu, Zhan, Xue, Tong, Wang, Wang, He, Chen, Xiao, Hawdon and Hotez2001), with permission from Allen Press Publishing Services. (C) Mean T. trichiuris eggs per gram of faeces by age group (n=96, study area: Cameroon, method: Kato-Katz thick smear). Reprinted from the Journal of Infectious Diseases (Faulkner et al. Reference Faulkner, Turner, Kamgno, Pion, Boussinesq and Bradley2002), with permission from the University of Chicago Press. (D) Age-infection intensity profiles of S. haematobium egg counts per 10 ml of urine from an area of low infection prevalence (dashed line) and an area of high infection prevalence (solid line) group (n=133 and 147, study area: Zimbabwe, method: filtration of 10 ml of urine) (re-drawn from Mutapi et al. Reference Mutapi, Ndhlovu, Hagan and Woolhouse1997). Reprinted from Parasitology Today (Woolhouse, Reference Woolhouse1998), with permission from Elsevier and John Wiley and Sons Ltd.
Additionally, whilst some helminths have been known to survive for extremely long periods (Vermund et al. Reference Vermund, Bradley and Ruiztiben1983), most die before reaching their maximum longevity. It is possible that the relatively short average helminth life-span in immunocompetent individuals reaps the maximal reproductive success relative to the physiological cost to the parasite of evading and modulating the host immune response. In opposition to this, studies in hookworm suggest that the immune response has only a limited impact on parasite longevity (reviewed by Loukas and Prociv, Reference Loukas and Prociv2001). Species with indirect life cycles, such as Schistosoma spp. and vector-borne helminths, tend to be long-lived (Table 1). Thus, once adult worms have adapted to their optimal host niche, they can produce offspring over a long period and, whilst they are immunogenic, adult worms do not appear to be the primary targets of the anti-helminth immune response in humans or in animal models (Smithers and Terry, Reference Smithers and Terry1967). This may be particularly true of O. volvulus, which has an average adult life-span of 8–10 years, but can have a maturation delay in the human host of over a year (Anderson and May, Reference Anderson and May1985). For short-lived worms, such as E. vermicularis, maturation and oviposition occurs much earlier, with an associated shift in stage-specific immune responses (Anderson and May, Reference Anderson and May1985).
GENETIC HETEROGENEITIES IN HELMINTH INFECTION
Host genetics
The human immune system has evolved in the context of helminth infection and this relationship has led to significant changes in our immune genes (Fumagalli et al. Reference Fumagalli, Pozzoli, Cagliani, Comi, Riva, Clerici, Bresolin and Sironi2009; Maizels, Reference Maizels2009). A recent comprehensive study of 91 interleukin genes in 52 human populations found evidence for balancing selection in the evolution of the human immune response driven by helminth ‘species richness’, i.e. the greater diversity of helminths to which a population has been exposed, the greater diversity observed in interleukin alleles (Fumagalli et al. Reference Fumagalli, Pozzoli, Cagliani, Comi, Riva, Clerici, Bresolin and Sironi2009). These findings are consistent with helminths differentially affecting the human acquired immune response at all levels of the CD4+ T cell axis, including gene families involved in inflammation in the skin, mucosal immunity, cell proliferation and survival and Th2 cytokines (Fumagalli et al. Reference Fumagalli, Pozzoli, Cagliani, Comi, Riva, Clerici, Bresolin and Sironi2009). This is compounded by earlier studies indicating that many of the key genetic loci controlling the balance of effector and regulatory responses are polymorphic in the human population (Reference QuinnellQuinnell, 2003) and that certain human genes are associated with predisposition to infection with specific helminth parasites (Hoerauf et al. Reference Hoerauf, Kruse, Brattig, Heinzmann, Mueller-Myhsok and Deichmann2002; Peisong et al. Reference Peisong, Mao, Enomoto, Feng, Gloria-Bottini, Bottini, Shirakawa, Sun and Hopkin2004; Kouriba et al. Reference Kouriba, Chevillard, Bream, Argiro, Dessein, Arnaud, Sangare, Dabo, Beavogui, Arama, Traore, Doumbo and Dessein2005).
Additive genetic effects (heritability) significantly influence human infections with A. lumbricoides (Williams-Blangero et al. Reference Williams-Blangero, VandeBerg, Subedi, Aivaliotis, Rai, Upadhayay, Jha and Blangero2002b), T. trichiura (Williams-Blangero et al. Reference Williams-Blangero, McGarvey, Subedi, Wiest, Upadhayay, Rai, Jha, Olds, Wu and Blangero2002a), and S. mansoni (Bethony et al. Reference Bethony, Williams, Blangero, Kloos, Gazzinelli, Soares, Coelho, Alves-Fraga, Williams-Blangero, Loverde and Correa-Oliveira2002), whilst other studies have found host genetics to be less influential relative to exposure history (King et al. Reference King, Blanton, Muchiri, Ouma, Kariuki, Mungai, Magak, Kadzo, Ireri and Koech2004). This conflicting evidence suggests that helminth distribution, allele frequency and host behaviour in each locality may determine the relative importance of heritable factors in patterns of infection and immunity (Ellis et al. Reference Ellis, Raso, Li, Rong, Chen and McManus2007). Familial clustering of infection can further confound identification of genetic factors associated with helminth infection since in utero sensitization via transfer of helminth antigens and soluble immune factors from infected mothers to their offspring is independent of genotype (Eloi-Santos et al. Reference Eloi-Santos, Novato-Silva, Maselli, Gazzinelli, Colley and Correa-Oliveira1989; Lammie et al. Reference Lammie, Hitch, Allen, Hightower and Eberhard1991; Novato-Silva et al. Reference Novato-Silva, Gazzinelli and Colley1992) and co-habiting families often have similar exposure patterns (Smith et al. Reference Smith, DeKaminsky, Niwas, Soto and Jolly2001). T. trichiura and A. lumbricoides for example were found to be significantly associated with shared living conditions; however, when infection distribution was investigated in more depth, only T. trichiura infection intensity was heritable (Ellis et al. Reference Ellis, Raso, Li, Rong, Chen and McManus2007). In addition, populations in historically stable endemic areas may exhibit less heritable variation in host susceptibility if long-term selection against genotypes less favourable for a specific infection has occurred (King et al. Reference King, Blanton, Muchiri, Ouma, Kariuki, Mungai, Magak, Kadzo, Ireri and Koech2004), particularly where other species of helminth infection are uncommon (Fumagalli et al. Reference Fumagalli, Pozzoli, Cagliani, Comi, Riva, Clerici, Bresolin and Sironi2009).
Multiple gene loci have now been implicated in determining the degree of resistance to helminthiases and while many appear to relate to individual helminth species (reviewed by Quinnell, Reference Quinnell2003), there are also loci controlling multiple susceptibilities, as is the case for IL-13 polymorphisms associated with predisposition to S. haematobium (Kouriba et al. Reference Kouriba, Chevillard, Bream, Argiro, Dessein, Arnaud, Sangare, Dabo, Beavogui, Arama, Traore, Doumbo and Dessein2005) and O. volvulus (Hoerauf et al. Reference Hoerauf, Kruse, Brattig, Heinzmann, Mueller-Myhsok and Deichmann2002).
Parasite genetics
Widespread genetic variation between parasite species is an equally important source of heterogeneity in the development of acquired immune responses to infection. Since parasitic nematodes have arisen from several independent evolutionary pathways their classification within the Phylum Nematoda belies the huge diversity apparent from their distinct life-histories, physiology and proteomes (Dorris et al. Reference Dorris, De Ley and Blaxter1999). Comparisons between nematode worms at the genomic level have identified multiple species-specific gene sequences and transcription patterns, even between phylogenetically close organisms (Parkinson et al. Reference Parkinson, Mitreva, Whitton, Thomson, Daub, Martin, Schmid, Hall, Barrell, Waterston, McCarter and Blaxter2004). Among the 3 major human Schistosoma spp. differences in immunologically relevant antigens have been found. Notably the leading anti-schistosome vaccine candidate antigen; glutathione-S-transferase (GST), of S. mansoni differs from that of S. haematobium (Trottein et al. Reference Trottein, Godin, Pierce, Sellin, Taylor, Gorillot, Silva, Lecocq and Capron1992), though the impact of this on human immunity is unknown.
The existence of helminth homologues of human proteins (Pastrana et al. Reference Pastrana, Raghavan, FitzGerald, Eisinger, Metz, Bucala, Schleimer, Bickel and Scott1998; Gomez-Escobar et al. Reference Gomez-Escobar, Gregory and Maizels2000) and analyses that indicate more rapid evolution among parasite secreted proteins are compatible with the idea that parasitic helminths are diversifying fastest among antigens exposed to (and interacting with) the host immune system (Hoekstra et al. Reference Hoekstra, Visser, Otsen, Tibben, Lenstra and Roos2000; Harcus et al. Reference Harcus, Parkinson, Fernandez, Daub, Selkirk, Blaxter and Maizels2004). The relatively recent completion of the first parasitic nematode (B. malayi; Ghedin et al. Reference Ghedin, Wang, Spiro, Caler, Zhao, Crabtree, Allen, Delcher, Guiliano, Miranda-Saavedra, Angiuoli, Creasy, Amedeo, Haas, El-Sayed, Wortman, Feldblyum, Tallon, Schatz, Shumway, Koo, Salzberg, Schobel, Pertea, Pop, White, Barton, Carlow, Crawford, Daub, Dimmic, Estes, Foster, Ganatra, Gregory, Johnson, Jin, Komuniecki, Korf, Kumar, Laney, Li, Li, Lindblom, Lustigman, Ma, Maina, Martin, McCarter, McReynolds, Mitreva, Nutman, Parkinson, Peregrin-Alvarez, Poole, Ren, Saunders, Sluder, Smith, Stanke, Unnasch, Ware, Wei, Weil, Williams, Zhang, Fraser-Liggett, Slatko, Blaxter and Scott2007) and trematode genome projects (S. mansoni; Berriman et al. Reference Berriman, Haas, LoVerde, Wilson, Dillon, Cerqueira, Mashiyama, Al-Lazikani, Andrade, Ashton, Aslett, Bartholomeu, Blandin, Caffrey, Coghlan, Coulson, Day, Delcher, DeMarco, Djikeng, Eyre, Gamble, Ghedin, Gu, Hertz-Fowler, Hirai, Hirai, Houston, Ivens, Johnston, Lacerda, Macedo, McVeigh, Ning, Oliveira, Overington, Parkhill, Pertea, Pierce, Protasio, Quail, Rajandream, Rogers, Sajid, Salzberg, Stanke, Tivey, White, Williams, Wortman, Wu, Zamanian, Zerlotini, Fraser-Liggett, Barrell and El-Sayed2009) and S. japonicum (Zhou et al. Reference Zhou, Zheng, Chen, Zhang, Wang, Guo, Huang, Zhang, Huang, Jin, Dou, Hasegawa, Wang, Zhang, Zhou, Tao, Cao, Li, Vinar, Brejova, Brown, Li, Miller, Blair, Zhong, Chen, Hu, Wang, Zhang, Song, Chen, Xu, Xu, Ju, Huang, Brindley, McManus, Feng, Han, Lu, Ren, Wang, Gu, Kang, Chen, Chen, Chen, Wang, Yan, Wang, Lv, Jin, Wang, Pu, Zhang, Zhang, Hu, Zhu, Wang, Yu, Wang, Yang, Ning, Beriman, Wei, Ruan, Zhao, Wang, Liu, Wang, Zheng, Zhang, Wang, Han and Seque2009) will inevitably yield greater insights into the evolution of genetic diversity between different helminth species.
Genetic diversity within helminth species may partly explain the slow development of protective immunity in endemic populations. It has been proposed that natural infections consist of several genetically distinct parasite strains (Galvani, Reference Galvani2005), evidence for which comes from variations in the non-coding sequence identified via genome-wide scans (Hunt et al. Reference Hunt, Knox, Le Jambre, McNally and Anderson2008; Redman et al. Reference Redman, Grillo, Saunders, Packard, Jackson, Berriman and Gilleard2008). The effect of helminth strains on the host immune response has been investigated in theoretical models based on field studies of A. lumbricoides, N. americanus, S. haematobium and T. trichiura infections, which surmise that challenge with multiple strains delays development of resistance to infection in human populations because a different protective response must be mounted against each parasite genotype separately (Galvani, Reference Galvani2005).
INDIVIDUAL EXPOSURE HISTORY
The immune environment to which de novo helminth infections are exposed is not independent of a host's infection history (Woolhouse and Hagan, Reference Woolhouse and Hagan1999). This was first demonstrated in a study of Sudanese canal workers hyper-exposed to S. mansoni infection, which showed that those who had been occupationally exposed for over 10 years were more resistant to infection than newly recruited workers (Satti et al. Reference Satti, Lind, Vennervald, Sulaiman, Daffalla and Ghalib1996). More recently a direct comparison between 2 occupationally exposed male cohorts, found that chronically exposed individuals develop resistance to S. mansoni re-infection after significantly fewer rounds of praziquantel treatment than those with a shorter exposure history (Black et al. Reference Black, Mwinzi, Muok, Abudho, Fitzsimmons, Dunne, Karanja, Secor and Colley2010).
It is well known that in endemic populations worm burdens accumulate with age to a peak intensity and decline thereafter (Fisher, Reference Fisher1934) but the age at which peak prevalence occurs (Brooker et al. Reference Brooker, Donnelly and Guyatt2000) and the relative decline post-peak varies according to helminth species (Fig. 2). For example, intensity of W. bancrofti (Fig. 2A) and hookworm (Fig. 2B) infections tends to be highest in adults rather than children (Day et al. Reference Day, Grenfell, Spark, Kazura and Alpers1991b; Gandhi et al. Reference Gandhi, Chen, Khoshnood, Xing, Li, Liu, Zhan, Xue, Tong, Wang, Wang, He, Chen, Xiao, Hawdon and Hotez2001). In contrast, T. trichiuria (Fig. 2C) and schistosome (Fig. 2D) infection intensity peaks in childhood, after which worm burdens decline (Mutapi et al. Reference Mutapi, Ndhlovu, Hagan and Woolhouse1997; Faulkner et al. Reference Faulkner, Turner, Kamgno, Pion, Boussinesq and Bradley2002). Host behaviour may partially explain these differences, for example the risk of hookworm infection is highest in adults due to occupation-related exposure (Bradley and Chandiwana, Reference Bradley and Chandiwana1990), whilst exposure to schistosomiasis is determined by contact with water from an early age during washing and domestic activities (Rudge et al. Reference Rudge, Stothard, Basanez, Mgeni, Khamis, Khamis and Rollinson2008).
Evidence that the host immune response is important in shaping age-related variation includes the observation that peak worm burdens occur at a younger age in regions of high infection intensity than in regions with low or intermediate intensity, a phenomenon called the ‘peak shift’ (Woolhouse, Reference Woolhouse1998) (Fig. 2D). It is clear that, at least for long-term residents of an area endemic for a particular species of helminth, age is effectively a proxy of exposure history and cumulative exposure to parasite antigens over time can trigger changes in the immune response (Mutapi et al. Reference Mutapi, Burchmore, Mduluza, Midzi, Turner and Maizels2008). The latter assertion is supported by immuno-epidemiological studies in a variety of helminthiases indicating that cellular and humoral changes correlate with development of resistance to infection with age. For example in an S. haematobium-infected population, cross-sectional data showed that cytokine responses switch from a regulatory IL-10 response in younger individuals to an effector IL-5 response in older individuals (Mutapi et al. Reference Mutapi, Winborn, Midzi, Taylor, Mduluza and Maizels2007). Longitudinal studies of W. bancrofti-infected subjects in Papua New Guinea showed that adults are relatively resistant to parasite accrual (Day et al. Reference Day, Grenfell, Spark, Kazura and Alpers1991b) and that this could be attributed to parallel age-specific acquisition of anti-larval antibodies (Day et al. Reference Day, Gregory and Maizels1991a). As discussed above, development of this form of concomitant immunity may be one explanation for the lower prevalence of these species in adults (Day et al. Reference Day, Gregory and Maizels1991a,Reference Day, Grenfell, Spark, Kazura and Alpersb; MacDonald et al. Reference MacDonald, Turaga, Harmon-Brown, Tierney, Bennett, McCarthy, Simonek, Enyong, Moukatte and Lustigman2002).
In areas of high intensity transmission, people are exposed to infection from a very early age and these patterns vary according to parasite species (Sousa-Figueiredo et al. Reference Sousa-Figueiredo, Basanez, Mgeni, Khamis, Rollinson and Stothard2008). A recent study in Zanzibar found that whilst schistosome infections were rare in pre-school children, soil-transmitted helminths were already highly prevalent (Sousa-Figueiredo et al. Reference Sousa-Figueiredo, Basanez, Mgeni, Khamis, Rollinson and Stothard2008). Variation in maternal infection status may also introduce variation in the in utero exposure patterns and potentially lead to long-lasting effects on the anti-helminth immune response of their offspring. For example, Steel and colleagues showed that pre-natal priming during maternal helminth infection has far-reaching effects on anti-filarial immunity including clonal deletion of parasite-specific T cells (Steel et al. Reference Steel, Guinea, McCarthy and Ottesen1994) and life-long susceptibility to infection (Steel and Nutman, Reference Steel and Nutman2003). Cellular studies in endemic populations have shown that umbilical cord blood lymphocytes (CBL) from neonates born of W. bancrofti-infected mothers mount parasite-specific cytokine responses similar to those of maternal PBMCs and in contrast to neonates born of un-infected mothers (Malhotra et al. Reference Malhotra, Ouma, Wamachi, Kioko, Mungai, Omollo, Elson, Koech, Kazura and King1997). An extension of this study found that schistosome and B. malayi-specific cytokine responses persisted in childhood and significantly affected the CD4+ T cell polarization of Bacillus Calmette-Guerin (BCG) vaccine-specific responses in 2 to 10 year olds (Malhotra et al. Reference Malhotra, Mungai, Wamachi, Kioko, Ouma, Kazura and King1999). However, an investigation of CBL responses to N. americanus and O. volvulus antigens found no evidence for specific polarization of Th1 and Th2 responses in the offspring of helminth-infected mothers (Pit et al. Reference Pit, Polderman, Schulz-Key and Soboslay2000). To date only a very few studies have directly compared the effect of different helminth species on neonatal immunity, though researchers hypothesize that tissue-dwelling helminths may be associated with a greater trans-placental transfer of antigens (Eloi-Santos et al. Reference Eloi-Santos, Novato-Silva, Maselli, Gazzinelli, Colley and Correa-Oliveira1989; Novato-Silva et al. Reference Novato-Silva, Gazzinelli and Colley1992; Malhotra et al. Reference Malhotra, Ouma, Wamachi, Kioko, Mungai, Omollo, Elson, Koech, Kazura and King1997). Since maternal exposure patterns will inevitably affect neonatal and early post-natal exposure the contribution of parasite transmission route and the associated behavioural risk factors for infection to immune heterogeneity (discussed above) should not be overlooked in early life.
ANTI-HELMINTHIC TREATMENT
Anti-helminthic treatment can effectively clear infection and thus artificially disrupts host-parasite immunoepidemiology, for example immune reactivity has been shown to peak shortly after treatment in many species of helminth infection (Quinnell et al. Reference Quinnell, Pritchard, Raiko, Brown and Shaw2004; Watanabe et al. Reference Watanabe, Mwinzi, Black, Muok, Karanja, Secor and Colley2007). Following treatment, however, endemic populations become re-infected and the highest re-infection rates are consistently seen in individuals who carried high worm burdens before treatment suggesting pre-disposition to infection in certain hosts (Bundy et al. Reference Bundy, Cooper, Thompson, Didier and Simmons1988; Tingley et al. Reference Tingley, Butterworth, Anderson, Kariuki, Koech, Mugambi, Ouma, Siongok and Sturrock1988; Chandiwana et al. Reference Chandiwana, Woolhouse and Bradley1991).
In addition to clearing infection, even a single dose of chemotherapy can lead to changes in the host immune response to helminths. Successful treatment has been shown to enhance the proportion of effector T cells (Watanabe et al. Reference Watanabe, Mwinzi, Black, Muok, Karanja, Secor and Colley2007), increase the range of parasite proteins recognized by host antibodies and induce a more rapid switch to protective antibody isotypes (Mutapi et al. Reference Mutapi, Hagan, Woolhouse, Mduluza and Ndhlovu2003) in schistosomiasis patients. This post-treatment rebound in immune responsiveness could be due to heightened DC activation in an environment of high parasite death (Watanabe et al. Reference Watanabe, Mwinzi, Black, Muok, Karanja, Secor and Colley2007) and/or recovery of normal immune function following removal of immunosuppressive parasite excretory/secretory products (Maizels and Yazdanbakhsh, Reference Maizels and Yazdanbakhsh2003).
Furthermore, the large variation in treatment regimens employed in mass-treatment programmes, including the drug administered, number of treatments (single or repeated dose), age-ranges targeted (e.g. school-age children, whole population) and method of administration (e.g. school-based, hospital/clinic-based), is also a potential source of variation in host immunity within endemic populations. Notably, many treatment programmes exclude children under the age of 5 due to a perceived risk of side-effects, despite evidence that children can become both infected and a source of transmission from a very young age (Opara et al. Reference Opara, Udoidung and Ukpong2007). Most also employ single-dose regimens, despite evidence that repeated treatment may be more effective at augmenting protective immunity (Black et al. Reference Black, Mwinzi, Muok, Abudho, Fitzsimmons, Dunne, Karanja, Secor and Colley2010) potentially by periodically ‘boosting’ development of immunological memory (Woolhouse and Hagan, Reference Woolhouse and Hagan1999). Whilst the long-term effects of anti-helminthic treatment on the host acquired immune response remain unclear, the desired reduction in parasite prevalence and transmission inevitably impacts upon helminth immunoepidemiology.
CO-INFECTION
Co-infection adds a further level of complexity to host-pathogen interactions and is highly prevalent in some communities, particularly in sub-Saharan Africa (Raso et al. Reference Raso, Luginbuhl, Adjoua, Tian-Bi, Silue, Matthys, Vounatsou, Wang, Dumas, Holmes, Singer, Tanner, N'Goran and Utzinger2004; Brooker et al. Reference Brooker, Clements, Hotez, Hay, Tatem, Bundy and Snow2006). Here we focus on how co-infections of particular public health significance contribute to heterogeneity in the anti-helminth immune response and how these effects vary depending on the species of helminth infection.
Helminth-helminth co-infection
Certain combinations of helminth co-infection are more common in human populations than others, notably several nematode pairs including: A. lumbricoides and T. trichiura (Faulkner et al. Reference Faulkner, Turner, Behnke, Kamgno, Rowlinson, Bradley and Boussinesq2005; Ellis et al. Reference Ellis, Raso, Li, Rong, Chen and McManus2007), A. lumbricoides and hookworm (Fleming et al. Reference Fleming, Brooker, Geiger, Caldas, Correa-Oliveira, Hotez and Bethony2006) and O. volvulus and T. trichiura (Faulkner et al. Reference Faulkner, Turner, Behnke, Kamgno, Rowlinson, Bradley and Boussinesq2005). Interactions between schistosomes and nematodes in co-infection are more variable, with evidence for co-aggregation with hookworms (Fleming et al. Reference Fleming, Brooker, Geiger, Caldas, Correa-Oliveira, Hotez and Bethony2006) and T. trichiura (Ellis et al. Reference Ellis, Raso, Li, Rong, Chen and McManus2007), but no significant association with A. lumbricoides infection intensity (Tchuem Tchuente et al. Reference Tchuem Tchuente, Behnke, Gilbert, Southgate and Vercruysse2003; Fleming et al. Reference Fleming, Brooker, Geiger, Caldas, Correa-Oliveira, Hotez and Bethony2006; Ellis et al. Reference Ellis, Raso, Li, Rong, Chen and McManus2007). Co-infection also tends to be associated with higher worm burdens than those seen in single species infections (Tchuem Tchuente et al. Reference Tchuem Tchuente, Behnke, Gilbert, Southgate and Vercruysse2003). A. lumbricoides-T. trichiura co-infected individuals were also found to have higher IgG4:IgE ratios than their singly-infected counterparts (Figueiredo et al. Reference Figueiredo, Barreto, Rodrigues, Cooper, Silva, Amorim and Alcantara-Neves2010).
Several explanations for the variable effect of one helminth species on the likelihood of co-infection with another have been proposed, although it is unlikely that these factors act in isolation. Firstly, similarities in parasite life cycle might mean that certain species share common behavioural or genetic risk factors for infection (Ellis et al. Reference Ellis, Raso, Li, Rong, Chen and McManus2007; Pullan et al. Reference Pullan, Bethony, Geiger, Cundill, Correa-Oliveira, Quinnell and Brooker2008). Alternatively, since studies in animals and humans show that patent infection with one helminth species can depress both the humoral and cellular responses of the host to challenge by other parasites (Brady et al. Reference Brady, O'Neill, Dalton and Mills1999; Correa-Oliveira et al. Reference Correa-Oliveira, Golgher, Oliveira, Carvalho, Massara, Caldas, Colley and Gazzinelli2002) it is possible that these synergistic interactions result from non-specific ‘bystander suppression’ (Brady et al. Reference Brady, O'Neill, Dalton and Mills1999) or immunosuppression directed against cross-reactive antigens expressed by closely-related species (Geiger et al. Reference Geiger, Massara, Bethony, Soboslay, Carvalho and Correa-Oliveira2002). S. mansoni-exposed but patently uninfected Brazilian subjects, identified by egg and adult worm-specific antibodies, were found to have impaired responses to Ascaris spp. and hookworm infections (Correa-Oliveira et al. Reference Correa-Oliveira, Golgher, Oliveira, Carvalho, Massara, Caldas, Colley and Gazzinelli2002), suggesting that prior exposure to schistosomiasis may have a lasting impact on host responses to other helminths. Experimental studies comparing S. mansoni infection with antigen (adult worm and egg homogenates) administration, suggest that immunosuppressive mechanisms rely on the presence of active infection (Osada and Kanazawa, Reference Osada and Kanazawa2010). However there is a lack of data on the effect of exposure to one parasite on the immunobiology of subsequent infection with a different species. It is conceivable that, even after clearance of adult worms, sequestration of eggs in host tissues, cellular memory responses to cross-reactive antigens and impaired organ-function resulting from cumulative morbidity may all affect the response to subsequent helminthiases.
Helminth-malaria co-infection
Malaria is a predominantly intracellular protozoan infection and has a markedly different immunobiology to that of helminths. The host response to malaria is characterized by inflammatory cytokines causing periodic fevers and immune-mediated pathology and, though protective immunity is yet to be fully characterized, clearance of blood-stage infection positively correlates with titres of variant-specific cytophilic antibodies, particularly IgG3 (Cavanagh et al. Reference Cavanagh, Dodoo, Hviid, Kurtzhals, Theander, Akanmori, Polley, Conway, Koram and McBride2004).
Studies of the effect of malaria on immune responses to helminths are rare and tend to focus on the anti-malarial, rather than anti-helminth immune response. However, work on schistosomiasis-malaria co-infections indicate distinct effects on humoral and cellular responses (Mutapi et al. Reference Mutapi, Ndhlovu, Hagan and Woolhouse2000; Wilson et al. Reference Wilson, Jones, Mwatha, Kimani, Booth, Kariuki, Vennervald, Ouma, Muchiri and Dunne2008). On the one hand, co-infection has been associated with elevated titres of schistosome egg-specific IgE and IgG3 (Mutapi et al. Reference Mutapi, Ndhlovu, Hagan and Woolhouse2000), indicating that acute inflammatory responses to malaria may reverse helminth-mediated immune hyporesponsiveness. Consistent with this hypothesis, skewing of T cell responses by malaria can exacerbate liver and spleen pathology due to deficient regulation of schistosome egg-specific regulatory responses (Wilson et al. Reference Wilson, Jones, Mwatha, Kimani, Booth, Kariuki, Vennervald, Ouma, Muchiri and Dunne2008). Recent schistosome-malaria co-infection studies also show that co-infected children do not differ from singly infected children in their absolute numbers of circulating Tregs, but do exhibit reduced proportions of memory Tregs (Muok et al. Reference Muok, Mwinzi, Black, Carter, Ng'ang'a, Gicheru, Secor, Karanja and Colley2009). Both elevated malaria-specific IgG3 and high S. mansoni egg counts are risk factors for splenomegaly (Booth et al. Reference Booth, Vennervald, Kenty, Butterworth, Kariuki, Kadzo, Ireri, Amaganga, Kimani, Mwatha, Otedo, Ouma, Muchiri and Dunne2004b), suggesting that both severity of infection and host immunoreactivity contribute to the clinical outcome of malaria-schistosome co-infection (Diallo et al. Reference Diallo, Remoue, Schacht, Charrier, Dompnier, Pillet, Garraud, N'Diaye, Capron, Capron and Riveau2004).
In individuals concurrently infected with GI nematodes and malaria, the inflammatory response to Plasmodium spp. might be expected to blunt Th2 effector responses involved in clearance of adult nematodes (Mountford and Pearlman, Reference Mountford and Pearlman1998). For example, N. americanus-specific Th2 effector cytokine and total IgE responses were lower in Papua New Guinean subjects co-infected with malaria, despite no observable effect on hookworm-specific IFNγ or cell proliferation (Quinnell et al. Reference Quinnell, Pritchard, Raiko, Brown and Shaw2004). The same study found evidence for malaria and hookworm-mediated suppression of cell proliferation, but not effector cytokine secretion, in response to bacterial antigens (Quinnell et al. Reference Quinnell, Pritchard, Raiko, Brown and Shaw2004). Thus, malaria co-infection can limit the effector response to hookworm, but may not significantly affect hookworm-mediated immunosuppressive mechanisms. Conversely, in a Malian study of malaria-W. bancrofti co-infection, filariasis was significantly associated with elevated total and malaria-specific IL-10 and reduced IFNγ (Metenou et al. Reference Metenou, Dembele, Konate, Dolo, Coulibaly, Coulibaly, Diallo, Soumaoro, Coulibaly, Sanogo, Doumbia, Wagner, Traore, Klion, Mahanty and Nutman2009). As more co-infection studies are conducted it will become possible to more directly compare effects of malaria on the acquired immune responses mounted against different helminth species.
Helminth-HIV co-infection
Human Immunodeficiency Virus (HIV) is a lymphotrophic virus that replicates in CD4+ T cells leading to incremental abrogation of this cell population and progression to Acquired Immune Deficiency Syndrome (AIDS). Both HIV and helminth infection can be considered as immunocompromising infections, and it could therefore be predicted that these effects would synergise to the detriment of co-infected hosts.
Although co-infection studies tend to focus on immune responses to HIV, there is some evidence for heterogeneity in the reciprocal effects of HIV on helminth immunobiology. For example, HIV infection is associated with up-regulation of CTLA-4 expression and anergy, which might enhance helminth-mediated Treg induction and immune evasion (Steel and Nutman, Reference Steel and Nutman2003; Leng et al. Reference Leng, Bentwich and Borkow2006). Severe immunosuppression in advanced HIV/AIDS is also linked to abnormally high intensity Strongyloides spp. infections due to increased rates of auto-infection and, in some regions, strongyloidiasis is considered to be an AIDS-related opportunistic infection (Meamar et al. Reference Meamar, Rezaian, Mohraz, Hadighi and Kia2007). Furthermore, low CD4+ T cell counts in HIV seropositive individuals has been associated with increased tissue sequestration of schistosome eggs (Karanja et al. Reference Karanja, Colley, Nahlen, Ouma and Secor1997), although the relative impact that this has on host pathology has not been investigated.
In addition to generalized immunosuppression, HIV co-infections are negatively correlated with worm-specific effector cytokine responses (Sentongo et al. Reference Sentongo, Rubaale, Buttner and Brattig1998) and may therefore reduce clearance of both adult and immature worms. HIV-positive patients co-infected with S. mansoni had lower measurable agonist-specific IL-4 and parasite and agonist-specific IL-10 than their HIV-negative counterparts, but had similar levels of IFNγ (Mwinzi et al. Reference Mwinzi, Karanja, Colley, Orago and Secor2001). This finding was linked to an increased Th1:Th2 effector cytokine ratio in HIV-positive individuals (Mwinzi et al. Reference Mwinzi, Karanja, Colley, Orago and Secor2001), which may result from preferential infection and abrogation of activated Th2 cells relative to Th1 and naïve T cells (Maggi et al. Reference Maggi, Mazzetti, Ravina, Annunziato, De Carli, Piccinni, Manetti, Carbonari, Pesce and Del Prete1994).
Disruption of CD4+ T cell polarization and regulatory mechanisms might be predicted to exacerbate helminth-related pathologies (Maggi et al. Reference Maggi, Mazzetti, Ravina, Annunziato, De Carli, Piccinni, Manetti, Carbonari, Pesce and Del Prete1994; Booth et al. Reference Booth, Mwatha, Joseph, Jones, Kadzo, Ireri, Kazibwe, Kemijumbi, Kariuki, Kimani, Ouma, Kabatereine, Vennervald and Dunne2004a), particularly in GI infections which require robust Th2 cytokine responses for clearance. However, very few studies of nematode-HIV co-infected populations have been conducted to date. HIV co-infection with schistosomiasis did not affect anti-helminthic treatment efficacy in a Kenyan cohort with high intensity S. mansoni infections (Karanja et al. Reference Karanja, Boyer, Strand, Colley, Nahlen, Ouma and Secor1998). Researchers in the latter study hypothesized that these findings were partly due to schistosomiasis preceding HIV infection and that subsequent co-infection did not affect pre-existing immune responses to schistosome antigens (Secor et al. Reference Secor, Karanja and Colley2004). The latter is of particular interest given that HIV is most prevalent in sexually-active adults and thus co-infections tend to arise after initial exposure to helminth infections, which peak in intensity in childhood. Thus, the stage of HIV infection (and associated degree of immunosuppression) and population-specific age-infection intensity distributions of different helminthiases are potential sources of heterogeneity and should be considered when investigating the immunobiology of co-infections.
CONCLUSIONS
Parasitic helminths present a diverse challenge to the immune system. Their large proteome and broad-range of antigens alone may partially explain the slow development of resistance to helminthiases (Yazdanbakhsh and Sacks, Reference Yazdanbakhsh and Sacks2010). Furthermore, the stage-specific challenges (Day et al. Reference Day, Gregory and Maizels1991a,Reference Day, Grenfell, Spark, Kazura and Alpersb; MacDonald et al. Reference MacDonald, Turaga, Harmon-Brown, Tierney, Bennett, McCarthy, Simonek, Enyong, Moukatte and Lustigman2002) and genetic diversity within single species infections (Galvani, Reference Galvani2005) can lead to heterogeneity in anti-helminth immune responses in an individual host.
When comparing different species of helminth it is clear that immune-heterogeneity can transcend phylogenetic delineations, particularly with respect to parasite life-span and intra-host niche. Long-lived parasites and species inhabiting host tissues can only exist incognito via sophisticated immunosuppressive mechanisms that may compromise fecundity and transmission of infection (Karanja et al. Reference Karanja, Colley, Nahlen, Ouma and Secor1997; Maizels and Yazdanbakhsh, Reference Maizels and Yazdanbakhsh2003; Brattig, Reference Brattig2004). Short-lived and GI parasites face different challenges resulting in characteristic immunobiology. Whilst immunity to the tissue-dwelling nematodes is associated with a mixed CD4+ T cell immune response (Turaga et al. Reference Turaga, Tierney, Bennett, McCarthy, Simonek, Enyong, Moukatte and Lustigman2000), expulsion of GI nematode species is more specifically Th2-dependent (Turner et al. Reference Turner, Faulkner, Kamgno, Cormont, Van Snick, Else, Grencis, Behnke, Boussinesq and Bradley2003). Few studies have directly compared immune responses to GI nematodes, filarial nematodes and Schistosoma spp. in single-infections, potentially due to the high prevalence of co-infection, meaning that many of the hypothesized sources of inter-specific immune heterogeneities remain to be empirically tested.
Unlike in experimental animal infections, acquired immune heterogeneity in natural infections also arises from host variables. Genetic polymorphism in the human immune system inevitably translates into heterogeneous expression of cellular and humoral responses, as is evident in clustering of immune responses within populations and individual families (Quinnell, Reference Quinnell2003; Ellis et al. Reference Ellis, Raso, Li, Rong, Chen and McManus2007). The relatively recent discovery of new CD4+ T cell subclasses and identification of further plasticity in innate effector cells has led to re-evaluation of how helminths interact with their human host (Diaz and Allen, Reference Diaz and Allen2007; Jenkins and Allen, Reference Jenkins and Allen2010). Furthermore, the influence that host exposure-history, anti-helminthic treatment and co-infection have on anti-helminth responses indicates that immune heterogeneity arises not from the host and parasite in isolation, but also from the environment in which they interact.
Despite the diversity of helminth species and their host populations identifying the immunological mechanisms underlying these distinctions has been a challenge for field studies. Helminth-specific immune responses become activated and polarized at a very young age (Eloi-Santos et al. Reference Eloi-Santos, Novato-Silva, Maselli, Gazzinelli, Colley and Correa-Oliveira1989; Lammie et al. Reference Lammie, Hitch, Allen, Hightower and Eberhard1991; Novato-Silva et al. Reference Novato-Silva, Gazzinelli and Colley1992; Steel et al. Reference Steel, Guinea, McCarthy and Ottesen1994; King et al. Reference King, Malhotra, Mungai, Wamachi, Kioko, Ouma and Kazura1998). However, field studies often exclude individuals under the age of 5, focusing instead on older individuals in the chronic stage of infection with a more ambiguous exposure and treatment history. In addition, whilst co-evolution of humans and helminths has clearly led to shared features of an anti-macroparasite response (Jackson et al. Reference Jackson, Friberg, Little and Bradley2009), it is also possible that peripheral sampling methods, co-infection and long-term systemic disease may disguise integral differences in human immune responses (Hayes et al. Reference Hayes, Bancroft and Grencis2004). The cellular targets of systemic and highly pleiotropic cytokines and site-specific immune responses are particularly difficult to define in peripheral blood samples. Thus, in addition to expansion of elegant in vitro cellular assays, a wider range of immune correlates should be measured in the field to give a broader characterization of the host immune ‘phenotype’ in which these cells act.
Anthropophilic helminths are both evolutionarily ancient and alarmingly prevalent (Hotez et al. Reference Hotez, Brindley, Bethony, King, Pearce and Jacobson2008), yet the immune responses mounted against them and the best means of treating infection remain unclear. Characterization of risk factors for infection and morbidity requires immunological measures to be considered in the context of host and environmental variables, which can be as influential as those of the parasite, and in some cases more so (Jackson et al. Reference Jackson, Turner, Rentoul, Faulkner, Behnke, Hoyle, Grencis, Else, Kamgno, Bradley and Boussinesq2004a; Ellis et al. Reference Ellis, Raso, Li, Rong, Chen and McManus2007).
ACKNOWLEDGEMENTS
The authors would like to thank the American Journal of Tropical Medicine and Hygiene, Allen Press Publishing Services, the University of Chicago Press, Elsevier and John Wiley and Sons Ltd for permission to re-print published material. Financial support was provided by the Biotechnology and Biological Sciences Research Council, The Cunningham Trust, The Carnegie Trust for the Universities of Scotland, University of Edinburgh's Moray Endowment Fund, Tenovus Scotland and The Wellcome Trust (Grant numbers WT082028MA, 076561 and 090281). The Wellcome Trust also provided funding for this article to be made available in an Open Access environment.