- BMD
-
bone mineral density
- CD
-
coeliac disease
- MTHFR
-
methylenetetrahydrofolate reductase
- RCT
-
randomised controlled trials
Osteoporosis, a metabolic skeletal disease characterised by reduced bone mass and deterioration of bone micro-architecture, predisposes affected individuals to an increased risk of fragility fracture( Reference Peck, Burckhardt and Christiansen 1 ). It is a major public health problem, recently estimated to cost £5.5 billion in the UK alone( Reference Strom, Borgstrom and Kanis 2 ), with one in two women and one in five men over the age of 50 years expected to experience an osteoporotic fracture( Reference Van Staa, Dennison and Leufkens 3 ). Bone is a dynamic tissue in a constant state of remodelling. Bone formation generally outweighs bone resorption in the first three decades of life, and peak bone mass (i.e. the maximum bone mass achieved in adulthood) is accrued during this period. After this time, bone resorption is favoured and bone loss ensues, which in turn predisposes older individuals to weaker bones and increased risk of fracture( Reference Prentice 4 ).The most common osteoporotic fracture sites are at the spine, hip and wrist, with both spine and hip fractures accompanied by considerable disability and increased morbidity and mortality( Reference Kanis, McCloskey and Johansson 5 ).
The measurement of bone mineral density (BMD) through dual-energy X-ray absorptiometry scanning is currently the basis of diagnosing osteoporosis. The WHO defines osteoporosis as a BMD of less than or equal to 2·5 sd below the reference mean of a young adult with peak bone mass (i.e. a T-score of ≤−2·5 sd), and osteopenia (the precursor stage to osteoporosis) as a BMD of less than 1 sd but greater than 2.5 sd below the reference mean (i.e. a T-score between −1 and −2·5 sd)( Reference Kanis, Alexeeva and Bonjour 6 ). A BMD greater than or equal to 1 sd below the reference mean is defined as normal (i.e. a T-score of ≥−1 sd) according to the WHO criteria( Reference Kanis, Alexeeva and Bonjour 6 ). Each sd fall in BMD (i.e. per unit T-score) below the reference mean corresponds to an estimated 2-fold increase in fracture risk( Reference Cummings, Browner and Cummings 7 , Reference Marshall, Johnell and Wedel 8 ). While low BMD is an important predictor of osteoporosis, fracture incidence is the most important clinical end point for osteoporosis. In recent years, the FRAX® tool, an algorithm used to assess the fracture probability by integrating weighted clinical risk factors of disease (with or without BMD), was developed by the WHO and has been used to assess osteoporotic fracture risk( Reference Kanis, McCloskey and Johansson 9 ).
The aetiology of osteoporosis is multi-factorial, with genetic heritability estimated to account for approximately 30 % of osteoporotic fracture risk( Reference Michaelsson, Melhus and Ferm 10 ). Non-modifiable factors including age, parental history of hip fracture and genetically determined traits, such as Caucasian ethnicity and female sex, increase risk( Reference Strom, Borgstrom and Kanis 2 ). Similarly, various modifiable factors such as low body weight, physical inactivity, smoking, excessive alcohol intake and the long-term use of certain medications (e.g. glucocorticoids and some anti-convulsant drugs) increase the risk of osteoporosis. Considering the general trend towards ageing populations, and the corresponding increases in osteoporosis-related costs( Reference Strom, Borgstrom and Kanis 2 ), investigation of modifiable risk factors, along with the development of intervention strategies to reduce the incidence of disease, is vital. In terms of nutritional intervention, combined calcium and vitamin D supplementation are proven to reduce bone loss and fracture incidence( Reference Tang, Eslick and Nowson 11 ). However, it is also possible that the nutritional factors not typically linked to bone health could play protective roles. Emerging evidence in generally healthy cohorts worldwide suggests a protective effect of certain B-vitamins, and a detrimental effect of homocysteine and the 677C→T polymorphism in the gene encoding the folate-metabolising enzyme methylenetetrahydrofolate reductase (MTHFR), on bone health and fracture risk( Reference van Wijngaarden, Doets and Szczecinska 12 , Reference Wang and Liu 13 ).
Role of B-vitamins in homocysteine metabolism
The major determinant of plasma homocysteine concentration is the biomarker status of the metabolically related B-vitamins, primarily folate, and to lesser extent vitamins B12 and B6 (Fig. 1). Homocysteine is formed by the demethylation of dietary methionine. Homocysteine may then be metabolised to form cystathionine as the part of the trans-sulphuration pathway, requiring a vitamin B6 co-factor, or regenerate methionine, and in turn S-adenosyl methionine (a universal methyl donor). The latter reaction is catalysed by methionine synthase and requires 5-methyltetrahydrofolate, the principal circulating form of folate and vitamin B12 in its co-factor form, methylcobalamin. The formation of 5-methyltetrahydrofolate is catalysed by the enzyme MTHFR, which has the riboflavin-derived FAD as a prosthetic group. Supplementation with folic acid can lower homocysteine concentrations by an estimated 25 %, with vitamins B12 and B6 having additional homocysteine lowering effects (i.e. by a further 7 % each) in folate replete, and folate and riboflavin replete individuals, respectively( Reference McKinley, McNulty and McPartlin 14 , 15 ).
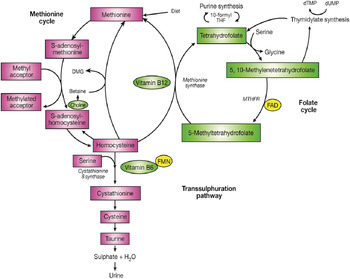
Fig. 1. (Colour online) The metabolism of homocysteine. MTHFR, methylenetetrahydrofolate reductase; DMG, dimethylglycine.
The 677C→T polymorphism in MTHFR is widely reported as the most common genetic determinant of mildly elevated plasma homocysteine concentrations in healthy populations( Reference Schwahn and Rozen 16 ). Individuals with the homozygous mutant TT genotype experience a decrease in MTHFR enzyme activity, believed to be through the loss of FAD( Reference Yamada, Chen and Rozen 17 ), leading to hyperhomocysteinemia (>15 μmol/l)( Reference Malinow, Bostom and Krauss 18 , Reference Frosst, Blom and Milos 19 ) and low erythrocyte folate concentrations( Reference Frosst, Blom and Milos 19 , Reference Molloy, Daly and Mills 20 ). Riboflavin supplementation has however been shown to significantly lower homocysteine concentrations specifically in individuals with the MTHFR 677TT genotype( Reference McNulty, Dowey le and Strain 21 ). The frequency of the MTHFR 677TT genotype varies greatly worldwide, ranging from 4 to 18 % in the US populations, 10 % in many European populations and up to 32 % in Mexico( Reference Botto and Yang 22 , Reference Wilcken, Bamforth and Li 23 ).
Hyperhomocysteinemia mainly reflects low or deficient status of the relevant B-vitamins, or the MTHFR genotype, but it can also reflect a decline in renal function with age( Reference Refsum, Smith and Ueland 24 ).
B-vitamins, homocysteine and bone health
Historical evidence
Homocystinuria, a rare autosomal recessive disease, is a condition in which the metabolism of homocysteine is severely impaired leading to excessive accumulation of homocysteine in the blood (>100 μmol/l) and urine( Reference Mudd, Skovby and Levy 25 ). The most common cause of homocystinuria is cystathionine-β-synthase deficiency. Among the major clinical features of the disease (including mental retardation and severe occlusive vascular disease) premature osteoporosis is well described, and homocystinuric patients are reported to have a 50 % increased risk of developing osteoporosis by the age of 16( Reference Mudd, Skovby and Levy 25 ). This premature onset of osteoporosis lends support to the hypothesis that mild elevations of homocysteine (and/or impaired status of related B-vitamins), may play a role in the aetiology of osteoporosis in the general population( Reference Maron and Loscalzo 26 ).
Early studies in patients with pernicious anaemia provided the first evidence to support a specific role for vitamin B12 deficiency in osteoporosis. One small study in post-menopausal women with pernicious anaemia (n 21) reported lower BMD in patients compared with controls( Reference Eastell, Vieira and Yergey 27 ). Further evidence from a slightly larger cohort of post-menopausal women (n 131) demonstrated an almost 3-fold increase in the risk of osteoporotic fracture among pernicious anaemia patients compared with the general community( Reference Goerss, Kim and Atkinson 28 ).
Evidence from observational studies
In the past decade, numerous large observational studies have provided evidence to support a role for homocysteine and/or low B-vitamin status in fracture risk (Table 1)( Reference van Wijngaarden, Doets and Szczecinska 12 , Reference Dai, Wang and Ang 29 – Reference McLean, Jacques and Selhub 37 ). In 2004, two landmark studies performed in the Netherlands and the USA produced consistent findings in terms of the magnitude of increased risk of fracture associated with mild elevations in homocysteine( Reference van Meurs, Dhonukshe-Rutten and Pluijm 36 , Reference McLean, Jacques and Selhub 37 ). In subsequent years, further studies from the USA, the Netherlands and Norway similarly demonstrated increases in fracture risk associated with elevated homocysteine concentrations( Reference Enneman, Swart and van Wijngaarden 31 – Reference Gjesdal, Voliset and Ueland 33 , Reference Dhonukshe-Rutten, Pluijm and de Groot 35 ). Confirming these findings, a recent meta-analysis involving nine prospective studies and 14 863 participants reported a relative risk of 1.59 (95 % CI 1.30, 1.96) and 1.67 (95 % CI 1.17, 2.38) for all fractures and hip fractures, respectively, in those within the highest homocysteine quartile compared with the lowest( Reference Yang, Hu and Zhang 30 ). Most recently, a dose–response meta-analysis including eight observational studies and 11 511 participants estimated a 4 % increase in fracture risk for every 1 μmol/l increase in homocysteine concentration( Reference van Wijngaarden, Doets and Szczecinska 12 ). Given the close metabolic relationship of the B-vitamins with homocysteine, studies have also demonstrated inverse associations between the status of the B-vitamins, folate, vitamins B12 and B6 and fracture risk in large cohorts of men and women( Reference Dai, Wang and Ang 29 , Reference Gjesdal, Voliset and Ueland 33 – Reference Dhonukshe-Rutten, Pluijm and de Groot 35 ).
Table 1. Observational evidence to support a role for homocysteine (Hcy) and B-vitamin status in fracture risk
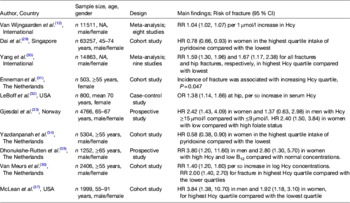
NA, not applicable; RR, relative risk; HR, hazard ratio.
Observational studies have also reported the significant associations of homocysteine concentrations and/or B-vitamin status with BMD (or loss of BMD over time; Table 2)( Reference Kim, Koh and Ahn 38 – Reference Cagnacci, Baldassari and Rivolta 44 ). Evidence from large USA and Norwegian cohorts demonstrated a significant inverse association of homocysteine concentrations with BMD( Reference Gjesdal, Vollset and Ueland 40 , Reference Morris, Jacques and Selhub 42 ). Additionally, a recent 3-year longitudinal study in a Korean population reported an accelerated rate of bone loss in a dose-dependent manner with increasing homocysteine concentration, an effect that was shown in men and pre-menopausal (but not post-menopausal) women( Reference Kim, Koh and Ahn 38 ). In contrast to the latter study that showed no homocysteine relationship in post-menopausal women, Zhu et al. in 2009 reported that high homocysteine concentrations were associated with greater bone loss after 4 years in 1213 post-menopausal Australian women( Reference Zhu, Beilby and Dick 39 ). Perhaps these apparently conflicting findings might be explained by the larger sample size (n 1213) and older age of the Australian women (mean age 75 years) compared with the much smaller sample (n 138) of younger (mean age 56 years) post-menopausal Korean women, as well as a longer duration of follow-up in the Australian study( Reference Kim, Koh and Ahn 38 , Reference Zhu, Beilby and Dick 39 ). In terms of the B-vitamins and BMD, analysis of the Hordaland Homocysteine Study found low plasma folate to be associated with low BMD among Norwegian women( Reference Gjesdal, Vollset and Ueland 40 ). Furthermore, the analysis of data from the Framingham Offspring Osteoporosis Study (n 2576), a large USA cohort, demonstrated a relationship between low vitamin B12 status and low BMD( Reference Tucker, Hannan and Qiao 43 ). Findings from the USA National Health and Nutrition Examination Survey (n 1550), also demonstrated that poorer vitamin B12 (but not folate) status was associated with lower BMD( Reference Morris, Jacques and Selhub 42 ). Additionally, a study of 714 older American men and women showed that the participants with deficient plasma vitamin B6 concentrations (<20 nmol/l) had greater mean annual bone loss than those with normal B6 concentrations( Reference McLean, Jacques and Selhub 41 ).
Table 2. Observational evidence to support a role for homocysteine (Hcy) and B-vitamin status in low bone mineral density (BMD) and bone loss
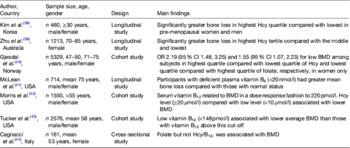
Some studies, however, have not reported associations between B-vitamins status (and/or homocysteine) and BMD( Reference Rumbak, Zizic and Sokolic 45 ), suggesting that the increased osteoporotic fracture risk may be independent of an effect on BMD. Indeed, Van Meurs et al. ( Reference van Meurs, Dhonukshe-Rutten and Pluijm 36 ) found a significant increase in fracture risk associated with the highest (compared with the lowest) quartile of homocysteine concentrations, but no significant differences in BMD values across homocysteine quartiles. Conversely, some studies have found an association of B-vitamin biomarkers with BMD but not with fracture risk. For example, the analysis of an Australian population by Zhu et al. demonstrated a significant association of homocysteine with BMD but not with fracture risk; although the latter study, however, may have lacked sufficient statistical power to observe an effect on fracture( Reference Zhu, Beilby and Dick 39 ). Additionally, some studies have found an effect on bone (through fracture risk and/or BMD) for folate but not for vitamin B12 ( Reference Gjesdal, Voliset and Ueland 33 , Reference Cagnacci, Baldassari and Rivolta 44 ) or an effect for vitamin B12 but not for folate( Reference Morris, Jacques and Selhub 42 ). Perhaps any beneficial effect of the B-vitamins may be the most evident in those with compromised status, and therefore the B-vitamin (i.e. folate or vitamin B12) that emerges as a risk factor may be more reflective of the presence of sub-optimal vitamin status in the population being studied. For instance, folate status may vary greatly between populations dependent on whether the folic acid fortification policy in place is mandatory (e.g. USA), voluntary (e.g. most European countries) or not permitted on any basis (e.g. Scandinavia). Apart from differences in the vitamin status, disparities in findings between studies may reflect fundamental differences in the populations under investigation including, although not limited to, differences in ethnicity, sex, age and menopausal status. Furthermore, methodological constraints pertaining to sample size, inclusion and exclusion criteria, methods of measuring B-vitamin status (such as the use of dietary intake data as opposed to more robust blood biomarker measures) and choice of outcome measures, may also contribute to inconsistent findings among studies( Reference Herrmann, Peter Schmidt and Umanskaya 46 ).
Genetic evidence
Epidemiological studies investigating the relationship between the MTHFR 677C→T polymorphism and bone health add further evidence to support the view that impaired folate metabolism (and the related phenotypes of elevated homocysteine and lower folate concentrations( Reference Frosst, Blom and Milos 19 , Reference Molloy, Daly and Mills 20 )) may be implicated in adverse outcomes (Table 3)( Reference Zhu, Beilby and Dick 39 , Reference Yazdanpanah, Uitterlinden and Zillikens 47 – Reference Miyao, Morita and Hosoi 53 ).
Table 3. The relationship between the 677C→T polymorphism in MTHFR and bone health outcomes bone mineral density (BMD) and fracture risk
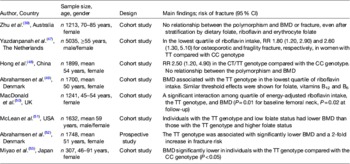
MTHFR, methylenetetrahydrofolate reductase; RR, relative risk.
The TT and CT genotypes refer to individuals homozygous and heterozygous for the 677C→T polymorphism in MTHFR respectively, whereas the CC genotype refers to homozygosity for the wild type.
Convincingly evidence from a recent meta-analysis of twenty studies (3525 cases and 17 909 controls) found that the 677C→T polymorphism was associated with BMD at all measured sites, and with a 23 % increased risk for all fractures in individuals with the MTHFR 677TT genotype compared with those with the CT or CC genotypes( Reference Wang and Liu 13 ). In 2000, the first report linking the 677C→T polymorphism in MTHFR with low BMD was published. This study of 307 healthy post-menopausal Japanese women demonstrated that those with the TT genotype (18·6 % of participants) had lower BMD than those with the CC genotype( Reference Miyao, Morita and Hosoi 53 ). Confirming these findings in a European population, the Danish Osteoporosis Prevention Study demonstrated that among 1748 post-menopausal Danish women, BMD at the spine and hip were significantly lower, and fracture incidence more than two times greater, in individuals with the TT genotype (affecting 8·7 % of participants) compared with the CT or CC genotypes( Reference Abrahamsen, Madsen and Tofteng 52 ). One subsequent study reported that individuals with the TT genotype and low folate status (<9 nmol/l), had a lower BMD compared with those with the CC or CT genotypes and low folate status, suggesting an important gene–nutrient interaction( Reference McLean, Karasik and Selhub 51 ). Furthermore, Gjesdal et al. in 2007 reported an almost 3-fold increased risk of fracture among Norwegian men and women with the TT genotype and low folate status compared with those with the TT genotype and high folate status( Reference Gjesdal, Voliset and Ueland 33 ).
Considering that riboflavin plays an integral role in homocysteine metabolism specifically in those with the MTHFR 677TT genotype( Reference McNulty, Dowey le and Strain 21 ), riboflavin intakes have also been investigated in relation to this polymorphism and bone health. In a longitudinal study, involving a subset of 1241 Scottish women from the Aberdeen Osteoporosis Screening Study, low riboflavin intake in combination with the TT genotype had an adverse effect on femoral neck BMD, at baseline and after a mean follow-up of 6·6 years( Reference Macdonald, McGuigan and Fraser 50 ). In support of these findings, further analysis of the Danish Osteoporosis Prevention Study cohort concluded that the BMD in individuals with the TT genotype was only significantly reduced with low dietary intakes of several B-vitamins including folate, B12, B6 and riboflavin( Reference Abrahamsen, Madsen and Tofteng 49 ). In terms of fracture risk, evidence from the Rotterdam Study (n 5035) demonstrated that women with the TT genotype in the lowest quartile of riboflavin intakes had 1·8 times greater risk of osteoporotic fracture, and 2·6 times greater risk of fragility fracture (defined as a fracture at the hip, pelvis or proximal humorus), than those in the same quartile of riboflavin intakes with the CC genotype( Reference Yazdanpanah, Uitterlinden and Zillikens 47 ). Such findings provide evidence to support a detrimental effect of the polymorphism combined with low B-vitamin intakes on robust bone health outcomes (i.e. BMD and fracture risk), and indicate that B-vitamins may have the potential to modulate any negative effect of this polymorphism on bone health.
To date the majority of bone studies that have considered gene–nutrient interactions have stratified B-vitamin status using dietary intake data, which are prone to a number of sources of error( Reference Zhu, Beilby and Dick 39 , Reference Hong, Hsu and Terwedow 48 ). For instance, Hong et al. in 2007 reported an increased fracture risk associated with the MTHFR 677TT genotype, but no effect of the polymorphism on BMD, even after stratification using food groups representative of B-vitamin intakes( Reference Hong, Hsu and Terwedow 48 ). Additionally Zhu et al. in 2009 failed to find an interactive effect of the polymorphism combined with low B-vitamin intakes on BMD or indeed fracture risk( Reference Zhu, Beilby and Dick 39 ). Perhaps these null findings might be explained by the inadequacy of B-vitamin intakes to accurately represent B-vitamin status. Other factors, such as differences in ethnicity (considerable variation in the frequency of this polymorphism between populations) and variation in the age of the populations investigated, may also contribute to inconsistencies among studies. Unfortunately, however, a recent meta-analysis showing an association between the MTHFR 677TT genotype and BMD and fracture risk, did not address any potential interactive effects of the polymorphism with low B-vitamin status and/or intakes, as most of the studies included did not provide the relevant data to allow this sub-analysis to be performed( Reference Wang and Liu 13 ).
Randomised controlled trials
Evidence in the form of randomised controlled trials (RCT) is limited. One notable RCT, however, found a 75 % reduction in the risk of hip fractures among 628 post-stroke Japanese patients in response to combined folic acid and vitamin B12 supplementation for 2 years, despite no statistical differences in the number of falls experienced in both the treatment and placebo groups( Reference Sato, Honda and Iwamoto 54 ). Conversely one RCT investigating osteoporotic fracture in stroke patients treated with B-vitamins for more than 2 years, and performed as part of the VITATOPS (VITAmins to Prevent Stroke) study, reported no benefit of B-vitamin treatment on osteoporotic fracture( Reference Gommans, Yi and Eikelboom 55 ). As acknowledged by the authors however, this trial was insufficiently powered to detect any potential beneficial effect due to the small number of fracture events( Reference Gommans, Yi and Eikelboom 55 ). Another RCT, using participants with pre-existing CVD from the Heart Outcomes Prevention Evaluation 2 trial, failed to find a beneficial effect of B-vitamin supplementation on fracture risk( Reference Sawka, Ray and Yi 56 ). It is important to note however, that the folate levels were approximately five times higher in the Heart Outcomes Prevention Evaluation 2 trial participants than in the Japanese stroke patients and so perhaps additional folate was unlikely to confer any further benefit to these individuals( Reference Sawka, Ray and Yi 56 ). It is possible that certain B-vitamins, particularly folate in countries with no folic acid fortification policy, can exert protective effects on bone health that are related to, or independent of, their homocysteine lowering effects.
Potential mechanisms linking B-vitamin biomarkers with bone
There is currently no clear mechanism to explain the potential role of the B-vitamins and/or homocysteine in bone health, however various hypotheses have been investigated.
Evidence to support an effect of homocysteine on bone
Evidence from a rat model suggests that hyperhomocysteinemia induces accumulation of collagen-bound homocysteine in bone resulting in decreased bone strength( Reference Herrmann, Tami and Wildemann 57 ). In support of this relationship, an early hypothesis by McKusick suggested homocysteine had a detrimental effect on bone strength by way of disrupting collagen cross-linking (integral to bone strength)( Reference Lubec, Fang-Kircher and Lubec 58 ). Consistent with McKusick's theory, an in vitro study by Kang & Trelstad in 1973, found that homocysteine interfered with collagen cross-links from purified rat skin collagen( Reference Kang and Trelstad 59 ) and this is supported by a recent cell study demonstrating a mechanistic effect of homocysteine on lysyl oxidase (an enzyme required for collagen cross-linking)( Reference Thaler, Agsten and Spitzer 60 ). Additionally, a small study in homocystinuric patients (n 10) and controls provided evidence that premature osteoporosis among patients could be explained by disruption of collagen cross-linking as opposed to a reduction in collagen syntheses( Reference Lubec, Fang-Kircher and Lubec 58 ). A case–control study by Saito et al. in 2006 comparing bone biopsies from twenty-five hip fracture cases and twenty-five post-mortem age- and sex-matched controls found significantly higher homocysteine concentrations, lower vitamin B6 concentrations and lower collagen cross-links in the hip fracture cases compared with controls( Reference Saito, Fujii and Marumo 61 ). However, while convincing, evidence to support a role for elevated homocysteine in the reduction of collagen cross-links as a causal mechanism in fracture risk remains inconclusive.
In vitro studies have also investigated a potential effect of homocysteine on bone turnover. Evidence exists to support a stimulatory effect of mildly elevated homocysteine concentrations (as low as 10 μmol/l) on osteoclastic activity, thereby increasing bone resorption( Reference Herrmann, Widmann and Colaianni 62 , Reference Koh, Lee and Kim 63 ) and an inhibitory effect of homocysteine on bone formation( Reference Kim, Koh and Lee 64 ). Consistent with such in vitro evidence, plasma homocysteine was shown to correlate positively with markers of bone resorption, but not bone formation, in peri- and post-menopausal women( Reference Herrmann, Kranzlin and Pape 65 ). Further observational evidence to support a relationship between homocysteine and markers of bone resorption has been described( Reference McLean and Hannan 66 ).
More recently, evidence from a rat model suggests homocysteine induces a reduced bone blood flow and therefore ability to repair micro-damage in bone( Reference Tyagi, Kandel and Munjal 67 ). Additionally, evidence to support a relationship between elevated homocysteine and impaired physical function suggests that homocysteine could act independently of bone, by increasing fracture risk through poor physical ability( Reference van Schoor, Swart and Pluijm 68 , Reference Swart, Enneman and van Wijngaarden 69 ).
Evidence to support an effect of folate on bone
While folate has been linked to BMD and a reduced fracture risk( Reference Sato, Honda and Iwamoto 54 , Reference Cagnacci, Bagni and Zini 70 ), there is limited evidence to support a direct mechanistic effect of folate on bone, although one study reported a reduced trabecular thickness of bone in individuals with low folate status v. those with higher status( Reference Holstein, Herrmann and Splett 71 ). Folate could have an indirect role on bone remodelling cells via its metabolic link (as a methyl source) to the methylation of DNA, proteins and other molecules and there is evidence, albeit somewhat inconsistent, to support a reduced methylation capacity as a pathomechanism responsible for poor bone health( Reference Herrmann, Tami and Wildemann 57 , Reference Enneman, van der Velde and de Jonge 72 – Reference Holstein, Herrmann and Splett 74 ). Alternatively, folate may exert a protective effect on bone through its homocysteine lowering effect( Reference Sato, Honda and Iwamoto 54 ).
Evidence to support an effect of vitamins B12 and B6 on bone
Findings from a small clinical trial showed significantly lower alkaline phosphatase (a marker of bone formation) in vitamin B12 deficient patients compared with the controls, and a subsequent rise in alkaline phosphatase following B12 supplementation in the deficient patients but not in the controls( Reference Carmel, Lau and Baylink 75 ). Additionally, evidence from an in vitro study showed a stimulatory effect of vitamin B12 on alkaline phosphatase( Reference Kim, Kim and Park 76 ). Such findings suggest a protective effect of vitamin B12 on bone formation. However, a large retrospective study of 9506 pernicious anaemia patients in the UK, which showed a 74 % increased fracture risk in patients v. healthy controls, reported that the increased fracture risk remained after correction of the vitamin B12 deficiency, thereby suggesting a detrimental effect of pernicious anaemia on bone not related to low vitamin B12 status( Reference Merriman, Putt and Metz 77 ). Low vitamin B6 status was found to be associated with decreased concentrations of bone formation markers in an animal model( Reference Masse, Delvin and Hauschka 78 ). However, trials involving supplementation of folate, vitamins B12 and B6, and thereby lowering homocysteine concentrations, have failed to find a beneficial effect on bone turnover; suggesting that if a benefit of B-vitamin supplementation, or homocysteine lowering, on bone health exists, it is independent of any effect on bone turnover( Reference Green, McMahon and Skeaff 79 , Reference Herrmann, Umanskaya and Traber 80 ). Interestingly, vitamin B6 acts as an essential co-enzyme for lysyl oxidase, an enzyme required for collagen cross-linking; suggesting that low vitamin B6 may be detrimental for collagen cross-linking and result in poorer bone mechanical performance( Reference Bird and Levene 81 , Reference Masse, Rimnac and Yamauchi 82 ).
It is apparent that the interrelationship between the B-vitamins, homocysteine and bone health is complicated, and probably multi-factorial, with further trials needed to fully elucidate potential mechanisms.
Bone health in coeliac disease
Coeliac disease
Coeliac disease (CD) is a common autoimmune, inflammatory condition in genetically predisposed individuals and is induced by the ingestion of ‘gluten’, a generic term used to describe the disease activating proteins and peptides found in wheat, barley, rye and sometimes in oats( Reference Green and Jabri 83 ).The mainstay treatment of CD is the gluten-free diet. Untreated CD is characterised by villous atrophy, leading to malabsorption of nutrients, and is responsible for many of the traditional clinical manifestations of disease including diarrhoea, weight loss and vitamin deficiencies in iron, folate and calcium. The gold standard diagnosis of CD is based on the presence of villous atrophy (or more mild features of enteropathy) as determined by a well-orientated biopsy of the small intestine, with the presence of autoantibodies to gluten (endomysial antibodies and tissue transglutaminase antibodies) being screened for in the first instance( Reference Green and Jabri 83 ).
Osteoporosis in coeliac disease
CD patients are well known to be at a higher risk of osteoporosis compared with the general population. A recent review reported that 38–72 % of CD patients have significantly lower BMD at presentation than non-CD populations matched for age and sex( Reference Strom, Borgstrom and Kanis 2 , Reference Larussa, Suraci and Nazionale 84 ). The gluten-free diet is expected to alleviate mucosal damage, and the corresponding malabsorption of calcium and other nutrients, and thereby results in higher BMD( Reference Pazianas, Butcher and Subhani 85 ). The evidence, however, suggests that the CD patients still fail to achieve the BMD of healthy controls( Reference Corazza, Disario and Cecchetti 86 ). Additionally, a meta-analysis involving eight studies and approximately 21 000 CD patients reported a 43 % increased fracture risk among patients compared with controls( Reference Olmos, Antelo and Vazquez 87 ). Although the aetiology of poor bone health in CD is unclear, various factors, including calcium and vitamin D malabsorption, resultant secondary hyperparathyroidism and the inability to reach peak bone mass in young adulthood, are reported to play a role( Reference Larussa, Suraci and Nazionale 84 ). More recently, a negative impact of pro-inflammatory markers (such as TNF-α and interferon-γ), which are chronically released during gut inflammation and associated with bone loss, are also believed to contribute to poor bone health in CD( Reference Tilg, Moschen and Kaser 88 ). Additionally, a lower ratio of osteoprotegerin (known to protect against excessive bone resorption) to receptor activator of NF-κB-ligand (RANKL; associated with bone resorption activity) found in treated CD patients (with recovered mucosa) compared with healthy controls, and a positive correlation between the osteoprotegerin :RANKL ratio and BMD, suggests a damaging effect of a low ratio on BMD even in treated CD patients( Reference Fiore, Pennisi and Ferro 89 ).
It is also possible that nutrients not typically linked to bone health, such as the B-vitamins, contribute a protective effect on bone in these patients. Low folate and vitamin B12 status, accompanied by elevated concentrations of homocysteine, are well described among CD patients( Reference Dickey, Ward and Whittle 90 ). Given the emergence of evidence to support a protective role of B-vitamins and a detrimental effect of homocysteine on bone health, it is possible that B-vitamin inadequacies and/or elevated concentrations of homocysteine, play a role in osteoporosis development in CD. Indeed, given the much higher incidence both of osteoporosis and B-vitamin deficiencies, CD patients comprise a unique ‘at-risk’ group to investigate in terms of a potential link between B-vitamins and bone health. A 2-year RCT supplementing CD patients with folic acid and vitamin B12 and measuring BMD (by dual-energy X-ray absorptiometry scans) pre- and post-intervention is currently underway at our centre with results expected in 2014. This trial will likely shed light as to whether a causal relationship between the B-vitamins and/or homocysteine and BMD exists.
Conclusion and future work
There is consistent observational evidence of an association of homocysteine related B-vitamins with bone health. This epidemiological evidence is further strengthened by genetic studies showing an important association between the common MTHFR 677C→T polymorphism and osteoporosis risk. However, confirmation of a causal link between B-vitamins and bone health from RCT is lacking. Further trials to investigate fracture risk or other robust outcomes such as BMD should focus on various ‘at-risk’ population groups (such as CD patients, post-menopausal women or individuals with the MTHFR 677TT genotype). Such evidence will confirm whether low B-vitamin status is causally associated with osteoporosis risk and whether there are beneficial effects of B-vitamin supplementation in bone health.
Acknowledgements
None.
Financial Support
This work was supported by funding from the Northern Ireland Department for Employment and Learning which funded the PhD studentship for M. M. C.
Conflicts of Interest
None.
Authorship
M. M. C. drafted the manuscript. H. McN., M. W., J. J. S. and W. D. critically revised the manuscript for important intellectual content. All authors have read and approved the final manuscript.