Introduction
In organic farming, perennial weed species, and in particular Cirsium arvense, can present farmers with significant challenges. This applies particularly to farms without livestock, where there is no weed management with perennial forage production. The integration of perennial forages into crop rotations has proven to be effective in managing C. arvense (Verschwele and Häusler, Reference Verschwele and Häusler2004; Zikeli and Gruber, Reference Zikeli and Gruber2017). On the other hand, conventional ploughing with a mouldboard plow (PL) (~25 cm) is considered to be a reliable method of reducing perennial weeds (Kouwenhoven et al., Reference Kouwenhoven, Perdok, Boer and Oomen2002; Bakken et al., Reference Bakken, Brandsæter, Eltun, Hansen, Mangerud, Pommeresche and Riley2009; Brandsæter et al., Reference Brandsæter, Bakken, Mangerud, Riley, Eltun and Fykse2011). Studies about reduced tillage, ie, non-inversion tillage or inversion tillage with shallower working depth than usual, in organic farming often report an increase in weed emergence and a shift in the weed community towards perennials and grasses (Peigné et al., Reference Peigné, Ball, Roger-Estrade and David2007; Armengot et al., Reference Armengot, Berner, Blanco-Moreno, Mäder and Sans2015; Nichols et al., Reference Nichols, Verhurst, Cox and Govaerts2015; Hofmeijer et al., Reference Hofmeijer, Krauss, Berner, Peigné, Mäder and Armengot2019). Although reduced tillage is often associated with non-inversion tillage, shallow ploughing is also reported to have adverse effects on weeds and the weed community (Kouwenhoven et al., Reference Kouwenhoven, Perdok, Boer and Oomen2002).
However, in addition to other techniques for reduced tillage, shallow ploughing (~12–20 cm) provides numerous advantages for the structure of the soil and soil life (Kouwenhoven et al., Reference Kouwenhoven, Perdok, Boer and Oomen2002). These include the retention of organic material on the surface, which protects the soil from wind and water erosion and promotes the proliferation of soil organisms and the enrichment of nutrients in the topsoil. Continuous biopores, ie, channels created in soil by roots and soil animals can develop in the subsoil and play an important role in the subsequent movement of water, air, and new roots (Blackwell et al., Reference Blackwell, Green and Mason1990). Energy consumption and working time are reduced with shallow ploughing compared to those with deep ploughing (Kouwenhoven et al., Reference Kouwenhoven, Perdok, Boer and Oomen2002). Water is saved through less soil disturbance. Therefore, it is expected that the application of shallow ploughing or other methods of reduced tillage will enhance the ecosystem services delivered by organic farming and make organic farming more resilient to the effects of climate change (Bloch and Bachinger, Reference Bloch and Bachinger2012; Zikeli and Gruber, Reference Zikeli and Gruber2017).
An advanced stubble PL and the stubble cleaner (SC), is designed to combine the benefits of a shallow working depth with the efficient management of weeds. Due to its shallow cutting depth and complete inversion, it could be a valuable tool in particular for the management of perennial weeds (Dierauer and Böhler, Reference Dierauer and Böhler2007). As its mouldboards are smaller than those of conventional PLs, the soil is not only turned but is also more thoroughly mixed (Schmidt, Reference Schmidt2010). Because of its shallow operating principle, the organic matter remains near the surface of the soil, which promotes tilth. The SC is especially recommended for the management of C. arvense (Heilmann, Reference Heilmann, Nordmeyer and Ulber2014). To test whether the SC is as effective in the control of C. arvense and other weeds as conventional ploughing in combination with perennial forage production, an organic field experiment was established.
For improved weed control and enhanced ecosystem services, reduced tillage may be combined with cover crops. Cover crops have the abilities to contribute to soil organic matter, improve soil structure and soil life and thereby soil fertility (Lütke Entrup, Reference Lütke Entrup2001). In addition, they have the potential to suppress weeds due to their competitive ability and allelopathy (Bàrberi, Reference Bàrberi2002; Shrestha et al., Reference Shrestha, Knezevic, Roy, Ball-Coelho and Swanton2002; Peigné et al., Reference Peigné, Ball, Roger-Estrade and David2007; Teasdale et al., Reference Teasdale, Brandsæter, Calegari, Skora Neto, Upadhyaya and Blackshaw2007). Cover crops are able to suppress weeds during their growing period as well as after termination or winterkill. However, living plants typically have a stronger weed-suppressing effect than plant residues through the absorption of light and competition with weeds for water, nutrients and space (Teasdale et al., Reference Teasdale, Brandsæter, Calegari, Skora Neto, Upadhyaya and Blackshaw2007). For organic farming systems with reduced tillage in the temperate and continental zones, cover crops that die off during winter are of special interest because no special treatment for their termination is needed. However, rapid soil cover and high biomass production during their short-growth period are important traits for cover crops that are used to control weeds (Stadler et al., Reference Stadler, Zihlmann, Scherrer, Jossi, Streit, Mayer, Alföldi, Leiber, Dubois, Fried, Heckendorn, Hillmann, Klocke, Lüscher, Riedel, Stolze, Strasser, Heijden and Willer2009; Brust et al., Reference Brust, Gerhards, Karanisa, Ruff and Kipp2011; Dorn et al., Reference Dorn, Stadler, Heijden and Streit2013). Lolium perenne (perennial ryegrass) is a perennial monocot with quick soil cover and abundant biomass production (Stadler et al., Reference Stadler, Zihlmann, Scherrer, Jossi, Streit, Mayer, Alföldi, Leiber, Dubois, Fried, Heckendorn, Hillmann, Klocke, Lüscher, Riedel, Stolze, Strasser, Heijden and Willer2009) and therefore considered well suited for weed control and tested in this study as well as the following cover crop species: Phacelia tanacetifolia (Phacelia) as a relatively undemanding non-leguminous cover crop with good weed-suppressing potential (Schmidt and Gläser, Reference Schmidt and Gläser2014), S. alba (white mustard) as a non-legume cover crop often grown by practitioners and having shown good weed suppression potential in several studies (Stadler et al., Reference Stadler, Zihlmann, Scherrer, Jossi, Streit, Mayer, Alföldi, Leiber, Dubois, Fried, Heckendorn, Hillmann, Klocke, Lüscher, Riedel, Stolze, Strasser, Heijden and Willer2009; Brust et al., Reference Brust, Gerhards, Karanisa, Ruff and Kipp2011; Dorn et al., Reference Dorn, Stadler, Heijden and Streit2013; Wittwer et al., Reference Wittwer, Dorn, Jossi, Zihlmann, van der Heijden, Neuhoff, Stumm, Ziegler, Rahmann, Hamm and Köpke2013; Brust et al., Reference Brust, Claupein and Gerhards2014), T. resupinatum (Persian clover) as a small-seeded legume, also suitable on light soils and in dry conditions (Schmidt and Gläser, Reference Schmidt and Gläser2014), and Vicia sativa (spring vetch) as a coarse-grained legume and having shown a good weed suppression potential (Dorn et al., Reference Dorn, Jossi, Heijden, Leithold, Becker, Brock, Fischinger, Spiegel, Spory, Wilbois and Williges2011; Wittwer et al., Reference Wittwer, Dorn, Jossi, Zihlmann, van der Heijden, Neuhoff, Stumm, Ziegler, Rahmann, Hamm and Köpke2013).
Higher weed emergence due to reduced tillage does not always lead to increased yield losses (Sans et al., Reference Sans, Berner, Armengot and Mäder2011; Armengot et al., Reference Armengot, Berner, Blanco-Moreno, Mäder and Sans2015). In addition, weeds can also provide important services: the living plant cover from weeds can protect the soil surface from soil erosion (Seitz et al., Reference Seitz, Goebes, Puerta, Pereira, Wittwer, Six, Van Der Heijden and Scholten2019), weeds influence the diversity and abundance of insect populations (Altieri, Reference Altieri1999; Marshall et al., Reference Marshall, Brown, Boatman, Lutman, Squire and Ward2003), and weeds can provide food for beneficial fauna. Studies have shown that a diverse and evenly distributed weed community limits the negative effects of competitive and dominant species on crop productivity (Storkey and Neve, Reference Storkey and Neve2018; Adeux et al., Reference Adeux, Vieren, Carlesi, Barberi, Munier-Jolain and Cordeau2019). Therefore, in this study, a third emphasis was to assess the diversity and distribution of the weed communities in the different tillage/crop rotation systems.
The effects of the three tillage/crop rotation systems and the cover crop treatments on the emergence of C. arvense, the total weed emergence, and the weed diversity in the sixth year of the experiment are the subjects of this paper. The experimental results were used to test the following hypotheses:
The SC in a cereal-based crop rotation is as effective in managing C. arvense and other weeds as growing three years of alfalfa–grass in a conventional PL system and more effective than a conventional PL in a cereal-based crop rotation.
Cover crops reduce weed emergence compared to bare fallow.
The different tillage/crop rotation systems have significant effects on weed diversity and evenness.
Material and methods
Site description
The trial was conducted at ‘Domaene Frankenhausen,’ the teaching and experimental farm of the University of Kassel, in the ‘Untere Kiebitzbreite’ field (51.41 N, 9.45 E; 252 m above sea level). The soil type is Haplic Luvisol. The soil texture in the PL horizon ranges from strong clayey silt (Ut4) to very silty clay (Tu4) (Brandt et al., Reference Brandt, Wildhagen and Heß2001). The pH value in the PL horizon is 6.6, and the soil organic carbon content is 1.16%.
Trial setup
This study builds upon a trial that was designed to compare the influence of conventional tillage with reduced-tillage on the development of C. arvense in a cereal-based crop rotation (Haase and Heß, Reference Haase, Heß, Leithold, Becker, Brock, Fischinger, Spiegel, Spory, Wilbois and Williges2011). To this end, a multiyear trial was initiated in 2007 as a randomized block design with four replications. Each plot had a length of 72 m and a width of 10 m. Three different farming systems were compared: an SC system and a PL system, both in a cereal-based crop rotation and an additional PL system in a crop rotation that included alfalfa–grass ley [perennial alfalfa–grass mixture (PLALF)] (Table 1). Conventional tillage in autumn was performed with a mouldboard PL (company: Kverneland, Soest, Germany) to a depth of 25 cm and in spring with a chisel to a maximum depth of 10 cm. Reduced tillage was carried out solely with the SC (Fig. 1; company: Zobel, Roth am See, Germany) to a maximum depth of 10 cm.

Fig. 1. Stubble cleaner (picture: privat).
Table 1. Design of the crop rotation and tillage systems in the field experiment (2007–2012)

a L. perenne, P. tanacetifolia, S. alba, T. resupinatum, mixture of S. alba and T. resupinatum, V. sativa and bare fallow as control.
Alfalfa–grass mixture was sown in spring 2008 as an alternative crop rotation, as perennial forage production is a proven measure for the management of C. arvense in organic farming. The mixture consisted of 80% alfalfa and 20% grass (based on the seed weight). The cultivation of alfalfa–grass lasted three years. The alfalfa–grass was mowed two to three times per year, and the cut alfalfa–grass was removed. Tillage in this crop rotation took place after 2010 utilizing the same conventional ploughing described for the PL-crop rotation treatment. The SC was only used in the cereal-based crop rotation (Table 1).
In August 2011, ie, in the sixth year of the SC experiment, the single-factor trial was modified to a split-plot design by sowing cover crops in subplots of 60 m2 (10 m × 6 m) established within each original plot. On August 24, 2011, the PLALF and PL systems were ploughed to a depth of 25 cm and treated with a rotary harrow, while the SC system was solely treated with the SC to a maximum depth of 10 cm. Cover crops were sown on August 30/31, 2011, with a row spacing of 18.75 cm. The whole area was rolled after sowing. The fourfold repetition was maintained. The following legume and non-legume cover crop species were cultivated in pure stands and one mixture of two species:
• L. perenne (40 kg ha−1), cv. Lemmos.
• P. tanacetifolia (12 kg ha−1), cv. Boratus.
• S. alba (20 kg ha−1), cv. Asta.
• S. alba and T. resupinatum (mixture: 10 kg ha−1 each), cvs. Asta; Marco Polo.
• T. resupinatum (20 kg ha−1), cv. Marco Polo.
• V. sativa (40 kg ha−1), cv. Ereica.
• Bare fallow ( = control).
On October 17, 2011, flame weeding on the bare fallow ( = control) plots took place.
The cover crops were followed by sowing the main crop oat on April 17, 2012 (Avena sativa, cv. Scorpion, 400 germinable grains m−2, row spacing 12 cm). Before that, the cover crops were mulched with a rotary mower on April 16, 2012. The PLALF and PL systems were treated with a cultivator to a depth of 7–10 cm, while the SC system was treated with the SC to a depth of 7–10 cm. On April 17, 2012, the whole area was treated with a rotary harrow directly before sowing the oat.
The harvest of oat took place on August 13, 2012. The PLALF and PL systems were ploughed to a depth of 25 cm on October 01, 2012, while the SC system was treated with the SC on October 01, 2012 to a depth of 8 cm and on October 29, 2012, to a depth of 10 cm. In the next year, on April 18, 2013, the whole area was treated with a spring tine cultivator to a depth of 8 cm. On April 22, 2013, the whole area was harrowed with a rotary harrow and the main crop field bean was sown (Vicia faba, cv. Bioro, 40 germinable grains m−2, double rows with 15 cm spacing, 45 cm apart). No fertilization was carried out, no inoculation of the legumes took place, and aside from the flame weeding on the bare fallow plots in October 2011, no weed control took place during the trial period until 2012. The whole area was hoed once on June 11, 2013 during the growth of main crop field bean.
Data collection
For weeds, the weed cover by species was determined at a late stage of the cover crops (March 30, 2012, 30 weeks after planting) and a late stage of the main crop oat (July 25, 2012, 14 weeks after planting) and a late stage of main crop field bean (July 19, 2013, 12 weeks after planting) on an area of one square meter per plot. Reference images were used to estimate the percentage of soil cover. For the total weed cover, the coverage levels of the different species were added together. The weed density by species was determined at an early stage of the main crop oat (May 30, 2012, 6 weeks after planting) and an early stage of the main crop field bean (May 23, 2013, four weeks after planting), ie, all weed plants per species in the assessment area were counted. The assessment area was one-tenth of a square meter ( = 1000 cm2, ie, a square with a side length of ~31.6 cm) and was randomly distributed four times across each plot. The total aboveground weed biomass was quantified at a late stage of the main crop oat at the same time as the second weed cover assessment and in the same area. To determine the weed biomass, all weeds were cut close to the ground. The samples were dried in a drying oven at 80°C. After complete drying, the dry matter weight was determined.
Except for L. perenne, all cover crop species tested in this trial froze during winter. The tillage in spring (either the chisel in the PLALF and PL systems or the SC in the SC system) was not sufficient to terminate the growth of L. perenne completely. L. perenne volunteers were counted as weeds in the weed assessments.
Weeds that could not be identified to species level are referred to by genus (eg, Matricaria sp. or spp.).
In the trial year 2011/2012, all the treatments were assessed or sampled ( = 84 plots). In the trial year 2012/2013, only selected plots were assessed or sampled in order to check whether the cover crop species still had an effect in the second year after cultivation. The following treatments were selected for this purpose: Bare fallow, S. alba and V. sativa ( = 36 plots).
For a schematic representation of the assessments and samplings, see Figure 2.

Fig. 2. Timeline of assessments and samplings in 2011–2012.
Data analysis
The statistical analyses were performed with SPSS-21. Mean values as well as standard errors were calculated to describe the distribution of weed emergence and weed diversity. Regression analysis was used for analysing the relation of cover crop biomass and weed emergence. Each data set was checked for normally distributed residuals (Kolmogorov–Smirnov test). If the distribution was not normal, the data were transformed for statistical evaluation. The type of transformation is indicated in the results. The representation of the data in the bar charts is based on the mean values of the original data. The main plot, subplot and block were examined for significant effects and interactions as fixed factors using a univariate analysis of variance. If the analysis of variance showed significant effects or interactions, a post hoc test (Tukey-B) was then carried out for the main effects or the simple effects of cover crops and tillage (α ≤ 0.05). Significant differences between treatments are indicated by different letters in the figures.
For the assessment of the diversity of the weed community, the following indices were calculated:
• Species richness (S), ie, the total number of weed species per treatment
• Shannon–Wiener index H' = −∑ Pi × ln Pi (where Pi is the proportion of density or cover of species i out of the total density or cover of the weed community) (Spektrum, 2020)
• Evenness (E) = H' /ln S (where ‘S’ refers to species richness).
Results and discussion
Weed emergence
The assessments regarding weed emergence include an assessment of weed cover during the cover crops, and assessments of weed density, weed cover and weed biomass in the main crop oat and main crop field bean.
The emergence of C. arvense
The analysis of variance of the cover crop and tillage/crop rotation system for the emergence of C. arvense in 2012 showed a significant effect of the tillage/crop rotation system for all assessment dates (Table 2). There was no significant effect of the cover crops on C. arvense emergence (Table 2).
Table 2. Summary of the analysis of variance for the effects of the cover crop, tillage/crop rotation system and their interaction on weed cover and weed density, coherent species richness (S), evenness (E) and Shannon–Wiener Index (H'), weed biomass, and C. arvense emergence

On all three assessment dates in 2012, the PLALF system led to significantly lower C. arvense emergence than the other two systems, ie, on March 30, 2012 and July 25, 2012, the cover (Fig. 3a and c), and on May 30, 2012, the density (Fig. 3b) of C. arvense was significantly lower in the PLALF system than those in the other two systems. The emergence of C. arvense was similarly high in both the PL and SC systems (Fig. 3a, b and c). The emergence of C. arvense in the PL and SC systems sharply increased during the growth of main crop oat and accounted for the majority of the weed population (between 37 and 85% in the PL system and between 72 and 87% in the SC system).
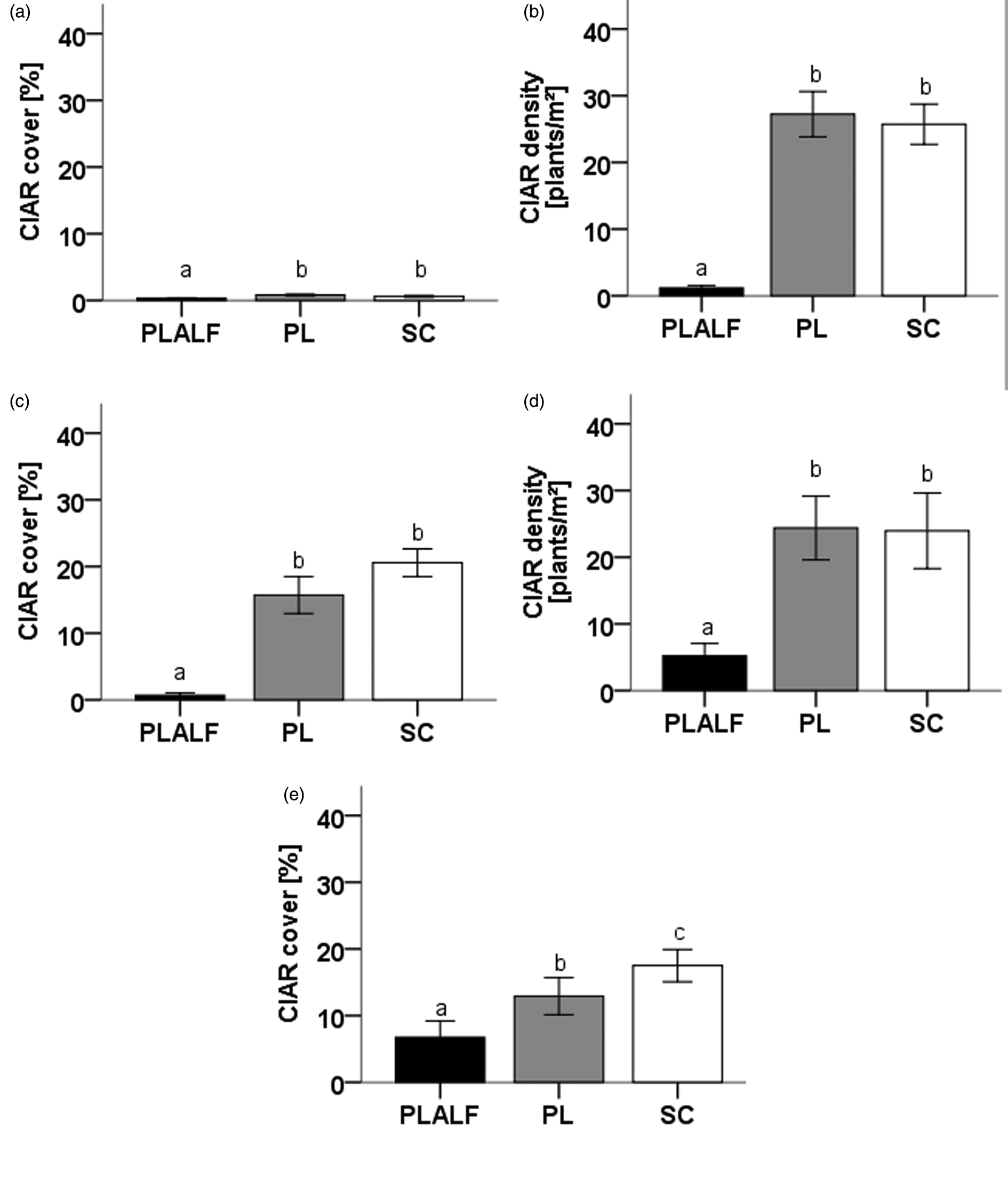
Fig. 3. (a–e) Emergence of C. arvense on three assessment dates in 2012 (a, b, c) and two assessment dates in 2013 (d, e) in different tillage/crop rotation systems. PLALF, conventional PL in a crop rotation with an alfalfa–grass ley; PL, plow in a cereal-based crop rotation; SC, stubble cleaner in a cereal-based crop rotation.
On both assessment dates in 2013 the tillage/crop rotation system had a significant effect on the emergence of C. arvense (Table 2). There was no significant effect of the cover crops in the second year after their cultivation and no significant interaction. On May 23, 2013 there was a significantly lower density of C. arvense in the PLALF system compared to the other two systems (Fig. 3d). On July 19, 2013, the PLALF system had a significantly lower cover of C. arvense than the SC system (Fig. 3e). The PL system had a medium cover of C. arvense.
From the beginning of the trial, an increase in the number of thistle patches could be observed in both the PL system and the SC system (Haase and Heß, Reference Haase, Heß, Leithold, Becker, Brock, Fischinger, Spiegel, Spory, Wilbois and Williges2011). Therefore, the SC did not meet the expectations placed upon it in this trial. However, this result must take into account that—as is often the case in practice as well (Schmidt, Reference Schmidt2010)—there was less experience with the use of the reduced tillage method compared to the mouldboard PL. This may have resulted in the SC not being used optimally, especially with regard to the timing of the tillage, which is especially important for the successful use of the SC.
Many studies describe an increase in perennial dicotyl weeds and grasses under reduced tillage (Shrestha et al., Reference Shrestha, Knezevic, Roy, Ball-Coelho and Swanton2002; Peigné et al., Reference Peigné, Ball, Roger-Estrade and David2007; Mäder and Berner, Reference Mäder and Berner2012; Armengot et al., Reference Armengot, Blanco-Moreno, Bàrberi, Bocci, Carlesi, Aendekerk, Berner, Celette, Grosse, Huiting, Kranzler, Luik, Mäder, Peigné, Stoll, Delfosse, Sukkel, Surböck, Westaway and Sans2016). Here, C. arvense levels increased in the PL and SC systems, ie, both tillage systems in the cereal-based crop rotation. In this study, the type of tillage had nearly no effect on the occurrence of C. arvense besides the weed community existed mainly in annual dicotyledons.
In a study by Brandsæter et al. (Reference Brandsæter, Bakken, Mangerud, Riley, Eltun and Fykse2011), deep ploughing (25 cm) resulted in up to a 90% lower incidence of C. arvense compared to shallow ploughing (15 cm). In the trial presented here, deep ploughing alone did not lead to satisfactory control of C. arvense. Only ploughing in the crop rotation with PLALF proved to be successful in regulating C. arvense. Studies by Lukashyk (Reference Lukashyk2005) and Lukashyk et al. (Reference Lukashyk, Berg and Köpke2008) show that repeated mowing is necessary for effective control of C. arvense by forage production. In our study, C. arvense was successfully controlled by three years of forage production and cutting two to three times.
A combination of crop rotation with perennial forage production and reduced tillage, such as the use of the SC, could combine the advantages of reduced tillage with the effective management of C. arvense. However, in studies by Gruber and Claupein (Reference Gruber and Claupein2008) and Gruber and Claupein (Reference Gruber and Claupein2009), shallow ploughing (15 cm) or cultivating (15 cm) soon led to the spread of C. arvense in winter wheat following alfalfa–grass mixture, while alfalfa–grass in combination with deep ploughing (25 cm) proved effective for the management of C. arvense.
Regrowth of alfalfa–grass or grass–clover as volunteers is a common problem when ploughing it under with reduced tillage. In their study, Gruber and Claupein (Reference Gruber and Claupein2008) also at least partially attribute the high occurrence of C. arvense after alfalfa–grass in a system using shallow ploughing or chiseling to the regrowth of alfalfa–grass. Other implements may be more effective for incorporating grass–clover or alfalfa–grass, such as a rotating tine roller following a rotary cultivator, which promotes the desiccating process of the grass–clover stubble, compared to only using the rotary cultivator (Reiter et al., Reference Reiter, Suntinger, Friedel, Häring, Hörning, Hoffmann-Bahnsen, Luley, Luthard, Pape and Trei2015). Krauss et al. (Reference Krauss, Berner, Burger, Wiemken, Niggli and Mäder2010) report successful ploughing under of clover in September with an SC and subsequent chiseling followed by sowing cover crops. Another promising tillage method in crop rotations with perennial forage production that may be applicable to the control of C. arvense is a two-layer PL system (Gruber and Claupein, Reference Gruber and Claupein2008; Gruber and Claupein, Reference Gruber and Claupein2009).
Research is currently underway into the possibilities of using grass–clover or alfalfa–grass at farms without livestock and incorporating the vegetation using reduced tillage. One possible application method for stockless farms is the so-called cut-and-carry green manure, ie, the use of cut grass–clover or alfalfa–grass mixture as fertilizer in other areas (Burgt et al., Reference Burgt, Eekeren, Scholberg and Koopmans2013).
Late stage of cover crop—weed cover
The data for weed cover assessed on March 30, 2012 were not normally distributed. Square root-transformed data were used for the statistical analysis. The analysis of variance of the cover crop and tillage/crop rotation system showed a significant cover crop by tillage/crop rotation system interaction (Table 2).
In the PLALF system, the cover crop treatments L. perenne, P. tanacetifolia and S. alba had a significantly lower weed cover than the bare fallow treatment, despite the flame weeding. In the SC system, L. perenne had a significantly lower weed cover than the bare fallow and T. resupinatum treatments. In the PL system there were no statistical differences due to cover crops (Fig. 4).

Fig. 4. Weed cover in the late stage of cover crops (March 30, 2012) sown in different tillage/crop rotation systems. PLALF, conventional PL in a crop rotation with an alfalfa–grass ley; PL, plow in a cereal-based crop rotation; SC, stubble cleaner in a cereal-based crop rotation. BR, bare fallow; LP, L. perenne; PT, P. tanacetifolia; SA, S. alba; TR, T. resupinatum; SATR, mixture of S. alba and T. resupinatum; VS, V. sativa.
The cover crop treatments reduced weed cover compared to the bare fallow in the late stage of the cover crops by 75% (PLALF system), 72% (PL system) and 61% (SC system). In a study by Dorn et al. (Reference Dorn, Jossi, Heijden, Leithold, Becker, Brock, Fischinger, Spiegel, Spory, Wilbois and Williges2011) and in cover crop trials in Saxony (Schmidt and Gläser, Reference Schmidt and Gläser2013), very high rates of weed emergence were also detected in the control plots without cover crops, whereas all the cover crop treatments demonstrated weed-suppressing ability. However, weed suppression through cover crops worked better in the PLALF and PL systems than in the SC system. This may be due to the lower weed pressure in these systems than in the SC system. In a study by Reimer et al. (Reference Reimer, Ringselle, Bergkvist, Westaway, Wittwer, Baresel, Van Der Heijden, Mangerud, Finckh and Brandsæter2019), the weed-suppressing effect of cover crops decreased with increasing weed pressure.
There is often a negative correlation between aboveground cover crop biomass and weed emergence (Bàrberi and Mazzoncini, Reference Bàrberi and Mazzoncini2001; Teasdale et al., Reference Teasdale, Brandsæter, Calegari, Skora Neto, Upadhyaya and Blackshaw2007; Döring et al., Reference Döring, Storkey, Baddeley, Collins, Crowley, Howlett, Jones, Mccalman, Measures, Pearce, Roderick, Watson and Wolfe2017; Grosse and Heß, Reference Grosse and Heß2018). Above ground cover crop biomass yield from the same trial published in Grosse et al. (Reference Grosse, Haase and Heß2019) showed that the non-legume cover crop species in the SC system had a lower biomass production compared to those in the ploughed systems. This may have caused the cover crops in the SC system to have a weaker suppression effect than the cover crops in the ploughed systems.
Among the cover crop species tested in this trial, L. perenne, P. tanacetifolia and S. alba suppressed weeds more effectively in the late stage of the cover crops than the mixture of S. alba and T. resupinatum, T. resupinatum and to some extent V. sativa. In the SC system, T. resupinatum did not suppress weeds at all compared to bare fallow. T. resupinatum is classified by Kolbe et al. (Reference Kolbe, Schuster, Hänsel, Grünbeck, Schließer, Köhler, Karalus, Krellig, Pommer and Arp2004) as unsatisfactory for weed control, which is attributed to its slow initial development.
In this experiment, the regression between cover crop biomass and weed cover in the late stage of cover crops was significant at the 0.000 probability level. In total, 43% of the variation in weed cover in the late stage of cover crops could be explained by cover crop biomass (y = 4.8594×10−0.048x; R 2 = 0.4256, bare fallow included with ‘0’ for ‘no cover crop biomass yield’). The data for T. resupinatum are not included, as it was not possible to determine its yield due to poor field emergence (Grosse et al., Reference Grosse, Haase and Heß2019).
Early stage of the main crop oat—weed density
The analysis of variance of the cover crop and tillage/crop rotation system for weed density assessed on May 30, 2012 showed a significant effect of the tillage/crop rotation system (Table 2). There was no significant effect of cover crops.
The SC system had a significantly higher weed density than the other two systems (Fig. 5). The PLALF system had a 76% and the PL system a 62% lower weed density than the SC system.

Fig. 5. Weed density in the early stage of main crop oat (May 30, 2012) in seven cover crop treatments sown in different tillage/crop rotation systems. PLALF, conventional PL in a crop rotation with an alfalfa–grass ley; PL, plow in a cereal-based crop rotation; SC, stubble cleaner in a cereal-based crop rotation. BR, bare fallow; LP, L. perenne; PT, P. tanacetifolia; SA, S. alba; TR, T. resupinatum; SATR, mixture of S. alba and T. resupinatum; VS, V. sativa.
Late stage of the main crop oat—weed cover and biomass
The data for weed cover assessed on July 25, 2012 were not normally distributed. Square root-transformed data were used for the statistical analysis.
The analysis of variance of the cover crop and tillage/crop rotation system for weed cover in the late stage of the main crop oat showed a significant cover crop by tillage/crop rotation system interaction (Table 2). There was no difference due to cover crop treatment within each system (Fig. 6). Compared to the other system treatments the PLALF × P. tanacetifolia treatment had the significantly lowest weed cover; while the SC × P. tanacetifolia,PL × bare fallow, PL × L. perenne, SC × P. tanacetifolia and SC × V. sativa treatments had the significantly highest weed cover.

Fig. 6. Weed cover in the late stage of main crop oat (July 25, 2012) in seven cover crop treatments sown in different tillage/crop rotation systems. PLALF, conventional PL in a crop rotation with an alfalfa–grass ley; PL, plow in a cereal-based crop rotation; SC, stubble cleaner in a cereal-based crop rotation. BR, bare fallow; LP, L. perenne; PT, P. tanacetifolia; SA, S. alba; TR, T. resupinatum; SATR, mixture of S. alba and T. resupinatum; VS, V. sativa.
The analysis of variance of the cover crop and tillage/crop rotation system for weed biomass showed a significant effect of the tillage/crop rotation system (Table 2). The PLALF system had significantly lower weed biomass than the other two systems (Fig. 7). Therefore, the alfalfa–grass ley resulted in better weed suppression than both the PL and SC tillage methods even two years after its termination.

Fig. 7. Weed biomass in the late stage of main crop oat (July 25, 2012) in seven cover crop treatments sown in different tillage/crop rotation systems. PLALF, conventional PL in a crop rotation with an alfalfa–grass ley; PL, plow in a cereal-based crop rotation; SC, stubble cleaner in a cereal-based crop rotation. BR, bare fallow; LP, L. perenne; PT, P. tanacetifolia; SA, S. alba; TR, T. resupinatum; SATR, mixture of S. alba and T. resupinatum; VS, V. sativa.
On both weed assessment dates that occurred during the oat crop, the influence of cover crops diminished compared to that on the first assessment date during the cover crops. This was also the case in a study by Reimer et al. (Reference Reimer, Ringselle, Bergkvist, Westaway, Wittwer, Baresel, Van Der Heijden, Mangerud, Finckh and Brandsæter2019), where cover crops reduced weeds throughout the cover crop period but only slightly during the main crop period. The weed-suppressing effect of the cover crops was overshadowed by the effects of the tillage/crop rotation systems, and the cover crops did not lead to a significant reduction in weed emergence beyond the cover crop period. However, if cover crops were used for several consecutive years, there would possibly be some identifiable long-term effects on weed reduction compared to that under bare fallow, as was the case in the study by Brust et al. (Reference Brust, Gerhards, Karanisa, Ruff and Kipp2011).
Main crop field bean—weed density, cover and biomass
On May 23, 2013, the analysis of variance of the cover crop and tillage/crop rotation system for weed density showed a significant effect of the tillage/crop rotation system (Table 2). The SC system had a significantly higher weed density than the other two systems (Fig. 8). There was no significant effect of cover crops in the second year after their cultivation and no significant interaction.

Fig. 8. Weed density in the early stage of main field bean (May 23, 2013) in different tillage/crop rotation systems. PLALF, conventional PL in a crop rotation with an alfalfa–grass ley; PL, plow in a cereal-based crop rotation; SC, stubble cleaner in a cereal-based crop rotation.
Neither the tillage/crop rotation systems nor the cover crop treatments had a significant effect on the weed cover and the weed biomass, which had been assessed on July 19, 2013 (Table 2). The hoeing process had led to an assimilation of the different systems.
Weed diversity
Species richness
There was a significant effect of cover crop treatment on species richness in the late stage of cover crops on March 30, 2012 (Table 2). V. sativa led to the lowest species richness among the cover crop treatments, ie, four to five species compared to four to seven (data not shown). Overall, 16 weed species or genera were identified. Capsella bursa-pastoris, C. arvense, Galium aparine, Lamium spp., Matricaria spp., Myosotis arvensis, Stellaria media, Trifolium spp., triticale (as a volunteer), and Viola spp. comprised the largest shares of weed cover.
Neither cover crop treatment nor tillage/crop rotation system had any effect on species richness during the oat crop (Table 2). On May 30, 2012, Brassica napus, Capsella bursa-pastoris, Chenopodium spp., C. arvense, Galeopsis tetrahit, Matricaria spp., Polygonum spp., Sinapis spp. and S. media were the most commonly occurring weeds (data not shown). On July 25, 2012, Apera spica-venti, Chenopodium spp., C. arvense, Galium aparine, L. perenne, Matricaria spp., Polygonum spp., Sinapis spp. and Sonchus spp. made up the majority of the weed cover.
There were no significant effects on species richness on both assessment dates in 2013 (Table 2).
Evenness
On March 30, 2012, the analysis of variance of the cover crop and tillage/crop rotation system showed a significant cover crop by tillage/crop rotation system interaction on evenness (Table 2). The SC × S. alba treatment had the significantly lowest evenness (0.37), while the PLALF × S. alba treatment had the highest evenness (0.96), and L. perenne had the highest evenness in the PLALF and PL systems (0.96 and 0.97, respectively) (Fig. 9).

Fig. 9. Evenness on March 30, 2012. The means and standard errors per treatment are given. Different letters indicate significant differences between tillage/crop rotation systems. PLALF, conventional PL in a crop rotation with an alfalfa–grass ley; PL, plow in a cereal-based crop rotation; SC, stubble cleaner in a cereal-based crop rotation. BR, bare fallow; LP, L. perenne; PT, P. tanacetifolia; SA, S. alba; TR, T. resupinatum, SATR, mixture of S. alba and T. resupinatum; VS, V. sativa.
On May 30, 2012, the tillage/crop rotation system resulted in significant differences in evenness; whereas cover crop treatment had no significant effect on evenness (Table 2). The SC system had significantly lower evenness than the other two systems (SC system: 0.41, compared to PL system: 0.51 and PLALF system: 0.59) (Fig. 10).
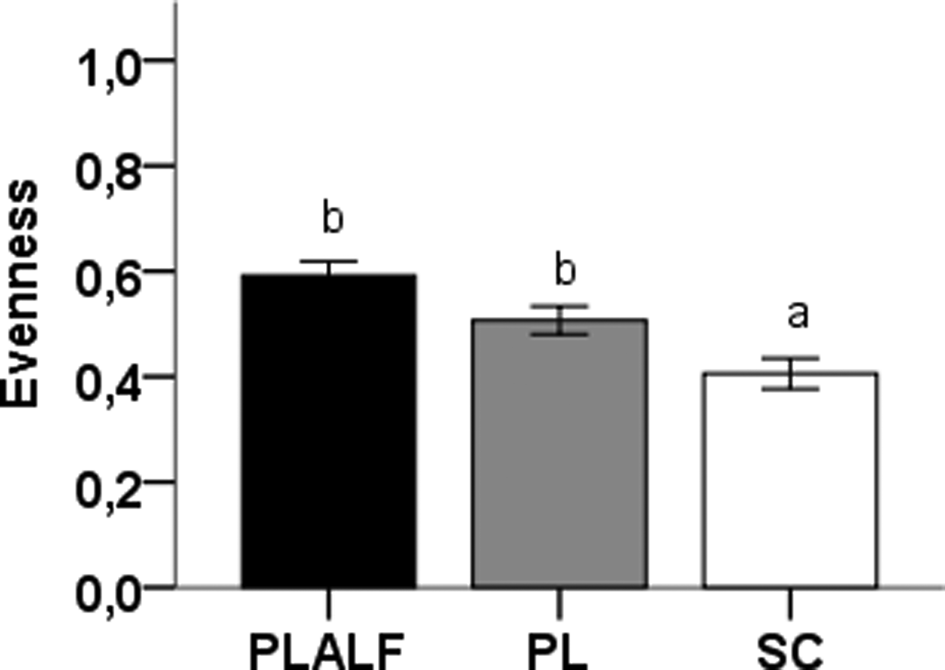
Fig. 10. Evenness on May 30, 2012. The means and standard errors per treatment are given. Different letters indicate significant differences between tillage/crop rotation systems. PLALF, conventional PL in a crop rotation with an alfalfa–grass ley; PL, plow in a cereal-based crop rotation; SC, stubble cleaner in a cereal-based crop rotation.
On July 25, 2012, the tillage/crop rotation system again resulted in significant differences but no difference due to cover crop (Table 2). The SC system had the significantly lowest evenness (0.63), the PL system had an intermediate evenness (0.74), and the PLALF system had the highest evenness (0.88) (Fig. 11).

Fig. 11. Evenness on July 25, 2012. The means and standard errors per treatment are given. Different letters indicate significant differences between tillage/crop rotation systems. PLALF, conventional PL in a crop rotation with an alfalfa–grass ley; PL, plow in a cereal-based crop rotation; SC, stubble cleaner in a cereal-based crop rotation.
The tillage/crop rotation system had a significant effect on evenness assessed on May 23, 2013 (Table 2). The cover crop treatments in the second year after sowing had no significant effect on evenness. The PL system had a significantly higher evenness than the PLALF and SC systems (Fig. 12). On July 19, 2013, there were no significant effects on evenness (Table 2).

Fig. 12. Evenness on May 23, 2013. The means and standard errors per treatment are given. Different letters indicate significant differences between tillage/crop rotation systems. PLALF, conventional PL in a crop rotation with an alfalfa–grass ley; PL, plow in a cereal-based crop rotation; SC, stubble cleaner in a cereal-based crop rotation.
Shannon–Wiener index
The analysis of variance of the cover crop and tillage/crop rotation system showed a significant cover crop by tillage/crop rotation system interaction on the Shannon–Wiener index on March 30, 2012 (Table 2). The SC × S. alba treatment and the SC × P. tanacetifolia treatment had the significantly lowest Shannon–Wiener index, whereas the PLALF × P. tanacetifolia, PLALF × the mixture of S. alba and T. resupinatum treatment, the PL × P. tanacetifolia, the PL × the mixture of S. alba and T. resupinatum treatment and the PL × T. resupinatum treatment had the significantly highest Shannon–Wiener index (Fig. 13).

Fig. 13. Shannon–Wiener index on March 30, 2012. The means and standard errors per treatment are given. Different letters indicate significant differences between tillage/crop rotation systems. PLALF, conventional PL in a crop rotation with an alfalfa–grass ley; PL, plow in a cereal-based crop rotation; SC, stubble cleaner in a cereal-based crop rotation. BR, bare fallow; LP, L. perenne; PT, P. tanacetifolia; SA, S. alba, TR, T. resupinatum; SATR, mixture of S. alba and T. resupinatum; VS, V. sativa.
On May 30, 2012, there was a significant effect of cover crop and tillage/crop rotation system on the Shannon–Wiener index (Table 2). Bare fallow had a significantly lower Shannon–Wiener index than S. alba and V. sativa (Fig. 14). The SC system again had a significantly lower Shannon–Wiener index than the other two systems. The Shannon–Wiener index was 1.26 for the SC system, 1.54 for the PL system, and 1.47 for the PLALF system.

Fig. 14. Shannon–Wiener index on May 30, 2012. The means and standard errors per treatment are given. Different letters indicate significant differences between tillage/crop rotation systems. PLALF, conventional PL in a crop rotation with an alfalfa–grass ley; PL, plow in a cereal-based crop rotation; SC, stubble cleaner in a cereal-based crop rotation. BR, bare fallow; LP, L. perenne, PT, P. tanacetifolia; SA, S. alba; TR, T. resupinatum; SATR, mixture of S. alba and T. resupinatum; VS, V. sativa.
On July 25, 2012, no significant effects of cover crop or tillage/crop rotation system on the Shannon–Wiener index were observed (Table 2).
On May 23, 2013, there was a significant effect of tillage/crop rotation system on the Shannon–Wiener index (Table 2). The cover crop treatments in the second year after sowing had no significant effect on the Shannon–Wiener index. The PL system had a significantly higher Shannon–Wiener index than the PLALF system (Fig. 15).

Fig. 15. Shannon–Wiener index on May 23, 2013. The means and standard errors per treatment are given. Different letters indicate significant differences between tillage/crop rotation systems. PLALF, conventional PL in a crop rotation with an alfalfa–grass ley; PL, plow in a cereal-based crop rotation; SC, stubble cleaner in a cereal-based crop rotation.
On July 19, 2013, there were no significant effects on the Shannon–Wiener index (Table 2).
An increase in cropping system diversity by the diversification of crop rotation and tillage guards against the development of a specialized weed community (Bàrberi, Reference Bàrberi2002). The predominance of one or a few weed species can therefore be a sign of uniform management lacking in diversity in the past.
The tillage/crop rotation system in the sixth year of the experiment had no significant effects on species richness. However, the tillage/crop rotation system had significant effects on evenness and the Shannon–Wiener index. Regarding evenness, the SC system had significantly lower values than the other two systems on two of three assessment dates. Regarding the Shannon–Wiener index, the SC system had significantly lower values than the other two systems on the second assessment date.
Other studies report increased weed diversity with reduced tillage (Bàrberi and Mazzoncini, Reference Bàrberi and Mazzoncini2001; Bàrberi et al., Reference Bàrberi, Aenderkerk, Antichi, Armengot, Berner, Bigongiali, Blanco-Moreno, Carlesi, Celette, Chamorro, Crowley, Döring, Grosse, Haase, Heß, Huiting, José-Maria, Klaedtke, Kranzler, Luik, Peigné, Sukkel, Surböck, Talgre and Sans2014; Armengot et al., Reference Armengot, Blanco-Moreno, Bàrberi, Bocci, Carlesi, Aendekerk, Berner, Celette, Grosse, Huiting, Kranzler, Luik, Mäder, Peigné, Stoll, Delfosse, Sukkel, Surböck, Westaway and Sans2016) or similar weed diversity compared to that under conventional tillage (Hampl, Reference Hampl, Heß and Rahmann2005; Sans et al., Reference Sans, Berner, Armengot and Mäder2011). In a study by Armengot et al. (Reference Armengot, Blanco-Moreno, Bàrberi, Bocci, Carlesi, Aendekerk, Berner, Celette, Grosse, Huiting, Kranzler, Luik, Mäder, Peigné, Stoll, Delfosse, Sukkel, Surböck, Westaway and Sans2016), the effect of tillage on weed diversity was dependent on the particular experiment and the cultivated crop. In their comparison of seven field experiments in different growing regions of Europe, the cultivated crop was even more important than the kind of tillage. However, in this experiment, the dependence on a single type of tillage—continuous tillage with the SC in the SC system compared to alternating inversion and non-inversion tillage in the two other systems—strongly influenced weed diversity. Additionally, the crop rotation differed in only one component, although it lasted for three years. Since alfalfa–grass mixture is grown for its ability to suppress weeds, it is not expected to increase weed diversity.
Conclusions
Alfalfa–grass mixture in crop rotation and ploughing (PLALF system) was found to be the best means of controlling C. arvense and other weeds compared to cereal-based crop rotation and ploughing (PL system) and cereal-based crop rotation with the SC (SC system).
For the weed community, the varying tillage (ploughing alternating with chiseling) resulted in higher weed diversity and evenness than the continuous use of the SC. Therefore, a recommendation for practice would be to alternate tillage strategies in reduced-tillage systems to prevent the development of detrimental weed communities with a few dominant species. For weed control, however, perennial forage legumes are still recommended, preferably with short-term cover crops in the rotation. More research is necessary to attempt to achieve similar benefits from a crop rotation that includes short-term cover crops but does not include perennial forage legumes.
Acknowledgements
This work was part of the FP7 ERA-Net (CORE Organic II)—project ‘Reduced tillage and green manures for sustainable organic cropping systems’ (TILMAN ORG, www.tilman-org.net). It was funded by grants from the Federal Program for Organic and Sustainable Farming supported by the German Federal Ministry of Food and Agriculture. The publication has been supported by the German Federal Ministry of Education and Research (BMBF) in the framework of the funding measure ‘Soil as a Sustainable Resource for the Bioeconomy—BonaRes (Module B): BonaRes Centre for Soil Research, subproject B’ (grant no. 031B0511B).
Conflict of interest
The authors declare that they have no conflict of interest.