Introduction
When compared to other commodity crops, cotton ranks as the third greatest user of pesticides in the USA (Swezey et al., Reference Swezey, Goldman, Jergens and Vargas1999; USDA, 2020a) and fourth greatest user of pesticides worldwide (Ferrigno et al., Reference Ferrigno, Guadagnini and Tyrell2017). According to the United States Department of Agriculture (2018), conventional cotton in the USA used $4.2 billion worth of pesticides in 2017, accounting for 6.35% by value of all the plant protection chemicals sold that year. In the USA alone, approximately 48 million pounds of pesticides were used on 12.6 million acres of cotton planted in nine states in 2017 (USDA, 2018), amounting to an approximate average of 3.8 pounds of pesticides per acre of cotton grown. Many of the insecticides, nematicides, fungicides, herbicides, desiccants and defoliants used in producing a conventional cotton crop have been associated with environmental and health problems (Maumbe and Swinton, Reference Maumbe and Swinton2003; Blackburn, Reference Blackburn2009; Settle et al., Reference Settle, Soumare, Sarr, Garba and Poisot2014; Agbohessi et al., Reference Agbohessi, Toko, Atchou, Tonato, Mandiki and Kestemont2015; Soil Association, 2019).
Decreased effectiveness of pesticides due to pesticide resistance has led to increased pesticide usage and higher costs of production in conventional cotton (Wossink and Denaux, Reference Wossink and Denaux2006; Benbrook, Reference Benbrook2012). For example, the United States Department of Agriculture (USDA) National Agricultural Statistics Service (NASS) Agricultural Chemical Use Program (2020a) shows that the pounds of glyphosate used nationally growing conventional cotton doubled between 2011 and 2019, and conventional cotton acres treated with pesticides increased by 38.2% (Fig. 1) (USDA, 2020b).
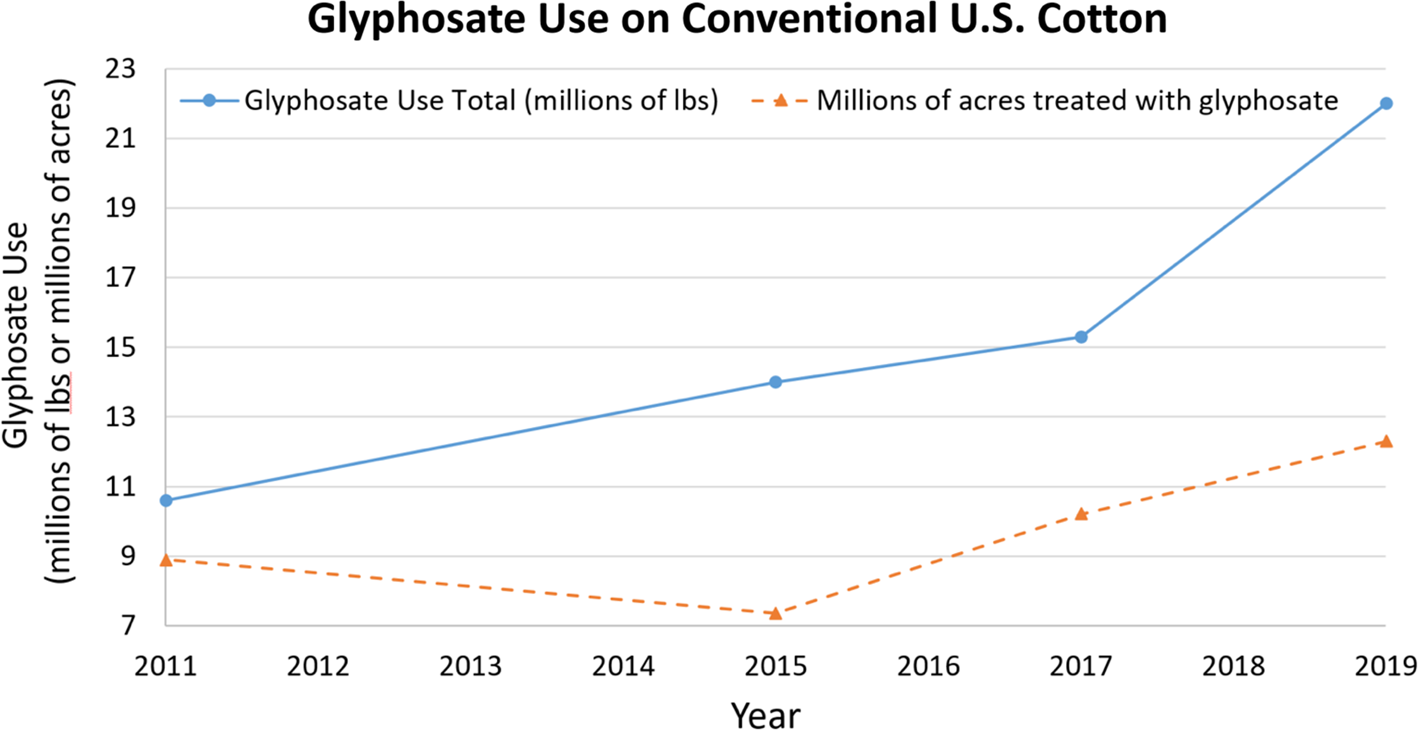
Fig. 1. Glyphosate use on conventional cotton grown in the USA. Both total glyphosate use and the number of acres treated with glyphosate have increased since 2011. Data from USDA-NASS, 2020: http://quickstats.nass.usda.gov.
Initiatives to reduce highly toxic synthetic chemical inputs used in conventional cotton production such as the Better Cotton Initiative (BCI) are attempts to address the negative environmental and health impacts of cotton production by developing standards for water stewardship, soil health, and biodiversity, among others (Shah et al., Reference Shah, Bansal, Singh, Benetto, Gericke and Guiton2018). These initiatives have gained traction with 360 licensed BCI farms in the 2017–18 cotton season accounting for approximately 5% of the US cotton production or about 1.1 million bales (BCI, 2019). However, some environmental standards, including BCI, still allow the use of glyphosate, which continues to foster the development of herbicide resistance across many weed species when used on genetically-modified (GM) crops such as herbicide-tolerant cotton (NAS, 2016). In addition, use of Bt (Bacillus thuringiensis) cotton, genetically modified to kill budworm/bollworm pests, and often cited as a ‘softer’ pest management approach, has resulted in a break-down of its insecticidal properties, evidenced by insect resistance identified in two of the three Bt toxin families (Cry1A and Cry2A) (Reisig, Reference Reisig2018).
In contrast, organic cotton production offers economically viable solutions to most of the environmental and health consequences associated with pesticide-intensive cotton production. Demand for organic cotton is growing and several studies have found that consumers are willing to pay price premiums for organic cotton (Hustvedt and Bernard, Reference Hustvedt and Bernard2008; Casadesus-Masanell et al., Reference Casadesus-Masanell, Crooke, Reinhardt and Vasishth2009; Ellis et al., Reference Ellis, McCracken and Skuza2012). Global production of organic cotton has increased in the last few years, with a 56% growth between 2016/17 and in 2017/18, reaching 831,193 bales (Textile Exchange, 2019). In 2019, the number of Global Organic Textile Standard (GOTS)-certified facilities grew globally by 35% across 70 countries (OTA, 2020). In the USA, organic production increased by 12% over the previous year's production in 2017, totaling about 23,341 bales of organic cotton fiber harvested over 26,302 acres (10,644 hectares) (Textile Exchange, 2019). Organic fiber is also the largest and fastest-growing sector in the organic non-food industry (including organic textiles, household products, personal care products, supplements, pet food and flowers) in the USA, with sales increasing 12.1% over 2019 to over $2 billion in 2019 (OTA, 2020). The consumer demand for domestic organic textile production has led to a rapid increase in GOTS-certified facilities in the USA. In 2019 alone, there was a 73% increase in US GOTS-certified facilities (OTA, 2020).
Despite this growth, organic adoption in cotton remains low in the USA. In 2011, for example, adoption of organic in cotton systems was lower than organic transition for any other domestic crop (USDA, 2013). Farmers considering transitioning to organic cite challenges to transition including cost, complex recordkeeping, on-farm production problems, lack of infrastructure and difficulties accessing to profitable markets (Stephenson et al., Reference Stephenson, Gwin, Schreiner and Brown2017). However, data on the agronomic challenges that organic cotton producers, in specific, are faced with is scant. While a study by Hanson et al. in 2004 found that cotton growers were especially concerned about risk from yield loss and price variability when considering transitioning to organic, a better understanding of current pre- and post-farm gate challenges for those in organic cotton production is needed (Hanson et al., Reference Hanson, Dismukes, Chambers, Greene and Kremen2004).
Because organic systems do not allow the use of genetic modification, synthetic fertilizers and most synthetic pesticides, organic cotton can be a more sustainable alternative to conventional cotton production. However, little research has examined the specific practices used by US organic cotton growers and the environmental aspects of those practices. Additionally, to more effectively encourage farmers to transition to organic, data is needed on current organic cotton production challenges. This study surveyed organic cotton producers and processors to better understand the specific approaches and techniques used in organic cotton production and processing and the environmental impacts of those techniques. We also highlight the challenges posed by organic cotton production and look at the future of how organic sustainable cotton can best be supported.
Methods
In spring 2019, a survey assessing the state of organic cotton production and handling in the USA was developed by researchers at Iowa State University (ISU) and mailed with a stamped, self-addressed return envelope to 113 organic cotton producers and handlers such as cotton gins. Those contacted were listed in the USDA-National Organic Program (NOP) Integrity Database (USDA-AMS-NOP, 2019) (Table 1). The USDA list included 119 organic cotton producers from ten states, with Texas predominating with 105 growers and 28 handlers. Organic cotton handlers were listed in six states, though the majority (21) were in Texas. Textile Exchange reported that the Texas Organic Cotton Marketing Coop (TOCMC) has approximately 35 producer members who plant approximately18–20,000 acres of organic cotton. The TOMC was established in 1993 and serves as an organic cotton production hub in the favorable cotton growing zone around Lubbock, Texas (TOMC, Reference [TOCMC]2020). While the USDA database lists 105 organic cotton producers in Texas, it does not provide acreage totals. Textile Exchange also lists Procot Cooperative, managed by Allenberg Cotton Company with additional farmer members (Textile Exchange, 2019).
Table 1. Number of US certified organic cotton producers and cotton handlers by state (USDA-AMS-NOP, 2019)
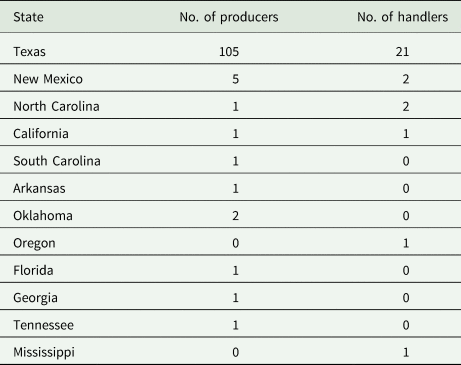
Organic cotton producers and handlers were asked about the growth of organic and conventional cotton and their respective acreage, rank pest issues they encounter and the prevalence of GMO contamination. Finally, they were asked about production techniques and other challenges to growing and/or marketing organic cotton.
Due to time limitations during the 2019 field season, only one survey was sent to each individual. Twelve producer and five processor surveys were returned, representing 15%, a return rate experienced with a previous ISU survey on organic production (Delate et al., Reference Delate, Canali, Turnbull, Tan and Colombo2016). Survey responses were followed up with telephone conversations to further explore grower and handler experiences.
Results
Survey results showed a strong recognition of production practices by farmers that attest to the knowledge of the environmental benefits of organic cotton production, as described in Table 2. The results also identified key pest management concerns as well as concerns with GM contamination, pesticide drift, weather, organic seed sourcing among other concerns.
Table 2. Organic practices cited in survey results and their benefits, compared with typical conventional cotton practices
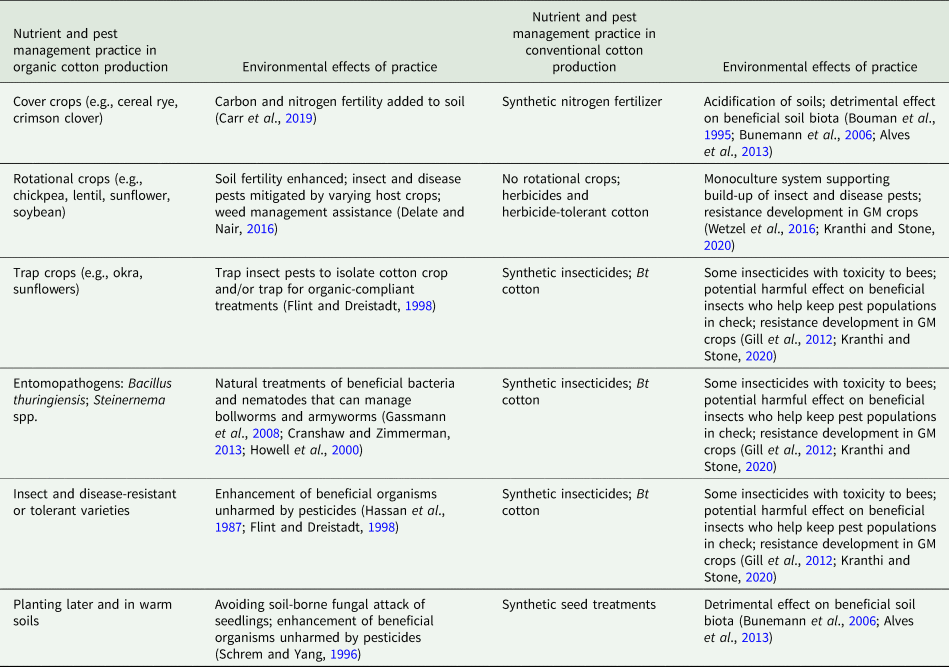
The range of organic practices cited in survey results included the use of cover crops, rotational crops, trap crops, entomopathogens, insect and disease-resistant or tolerant varieties and planting later to take advantage of warmer soils.
Sixty percent of the respondents noted weeds as the most critical pest management issue and 90% cited weed management within the three highest-ranked constraints. Among the weeds cited within organic cotton fields were (in order of abundance): bindweed, pigweed, lakeweed, Johnson grass, morning glory, nut grass and crabgrass.
While insect pests were not cited as challenging as weed management in organic cotton, the results showed the importance of bollworm, aphid and thrips management (Table 3).
Table 3. List of insect pests cited in 2019 survey of organic cotton producers
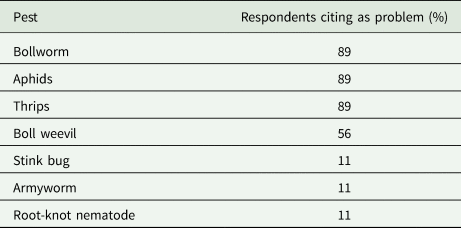
Twenty percent of producer respondents reported issues with GM contamination in their organic cotton crop, with levels between 2 and 10%. Eighty percent of organic cotton producers reported that they were able to source organic seed stock. Other challenges to organic production cited respondents included climatic conditions such as extreme weather events, inadvertent pesticide contamination and market access.
Discussion
Environmental impacts of organic management techniques
Unlike conventional cotton, which relies heavily on GM Bt-cotton for managing the main budworm/bollworm complex, organic management of insect pests uses a multi-pronged approach, including crop rotations, use of resistant or tolerant varieties, releases of beneficial insects and microorganisms targeting specific pests.
Since adopting organic practices, 40% of respondents reported increases in beneficial organisms on their farms, including lacewings, lady beetles and ‘microbes’ (presumably relating to their perception of greater soil quality). This trend corresponds with observations from other cropping systems where toxic pesticides have been eliminated or reduced, and specifically, in cotton, where organic fields hosted more generalist insect predators than conventional fields (Swezey et al., Reference Swezey, Goldman, Bryer and Nieto2007).
Additionally, frequently cited practices of organic cotton farmers responding to our survey are associated with multiple environmental benefits (Table 2). For example, organic cotton farmers, as for all organic farmers, are required by USDA-NOP rules to practice crop rotations to help maintain soil quality on their farms (USDA-AMS-NOP, 2019). There was a keen awareness among organic cotton producers relating to the need to rotate crops to help augment soil fertility, increase soil quality and assist with insect pest mitigation. For example, using cover crops and crop rotations helps build soil quality (Fageria et al., Reference Fageria, Baligar and Bailey2005; DuPont et al., Reference DuPont, Ferris and Van Horn2009; Haruna and Nkongolo, Reference Haruna and Nkongolo2015; Tully and McAskill, Reference Tully and McAskill2019).
There were 35 other crops listed on organic cotton farms in the USDA-NOP database, which can be assumed to be planted in rotation with cotton, since organic regulations prohibit growing the same crop on the same land each year (Table 4). Our survey found that cover crops such as cereal rye and crimson clover were the primary rotational cover crops used by organic cotton growers in the USA. Additionally, there is extensive research demonstrating the benefits of cover crops and rotating crops. In contrast, cotton monocropping may lead to a build-up of disease and depletion of soil fertility which, in turn, can lead to greater use of pesticides and fertilizers to compensate (Kurtz et al., Reference Kurtz, Boone, Peck and Hoeft1984; Bullock, Reference Bullock1992; Helmers et al., Reference Helmers, Yamoah and Varvel2001; Peters et al., Reference Peters, Sturz, Carter and Sanderson2003; Smith et al., Reference Smith, Gross and Robertson2008). By using organic principles and practices, organic farmers' methods have led to research showing organic soils tend to have higher levels of soil health metrics than conventional soils. For example, organic soils tend to have more aggregate stability, have higher water holding capacity and are more porous than conventionally managed soils (Lotter et al., Reference Lotter, Seidel and Liebhardt2003; Gomiero et al., Reference Gomiero, Pimentel and Paoletti2011). Organic practices can also lead to greater soil organic carbon sequestration (Mondelaers et al., Reference Mondelaers, Aertsens and Van Huylenbroeck2009; Gomiero et al., Reference Gomiero, Pimentel and Paoletti2011; Gattinger et al., Reference Gattinger, Muller and Haeni2012; Tuomisto et al., Reference Tuomisto, Hodge, Riordan and Macdonald2012; Ghabbour et al., Reference Ghabbour, Davies, Misiewicz, Alami, Askounis, Cuozzo, Filice, Haskell, Moy, Roach and Shade2017), which may aid in mitigating climate change.
Table 4. Other crops grown on organic cotton farms in the USA, USDA-NOP Integrity Database, 2019
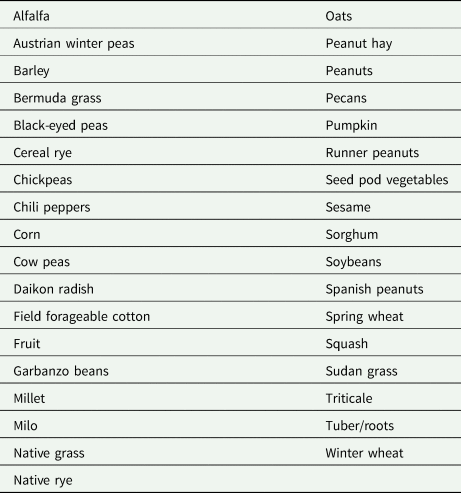
Enhancing water quality and quantity through moisture-conserving practices
According to the Textile Exchange (2014), cotton production accounts for 69% of textile fiber's water footprint. Organic cotton production practices can reduce water consumption by as much as 91% (Textile Exchange, 2017). Water management is a critical component of organic cotton production and processing. Boll and fiber properties, such as lint to seed ratio, and length, strength and micronaire (fineness of lint), are primarily determined by the cotton variety, as well as, to a lesser extent, irrigation and fertilization practices. An adequate water amount is necessary for vigorous growth, good budding and fruiting, and the formation of healthy bolls during cotton production, and ranges from 700 to 1300 mm, depending on climate and length of the total growing period (UN-FAO, 2019).
A delicate water balance is required for optimum production, with excess water early in the growing period restricting root and crop development and lack of sufficient water during bud formation depressing yields. Excessive vegetative growth can also result if too much water is present during flower opening and boll formation, leading to decreased yields. A moderate water deficit after peak flowering to restrict vegetative growth will lead to good boll-set and higher yields, despite a reduction in the number of flowers. Consistency in water supply will help ensure adequate growth and avoid yield depressing flower and boll shedding.
While the majority of cotton producers in the survey respondents rely on furrow or sprinkler irrigation, many are using or examining the potential for drip irrigation to reduce water quantities and energy costs. Dry land production, where cotton is grown without the aid of irrigation, has also been gaining popularity (Mauget et al., Reference Mauget, Marek, Adhikari, Leiker, Mahan, Payton and Ale2020). Biologically-based soil-building practices, such as crop rotation, cover crops and compost applications, have also been shown to increase the water-holding capacity of soils (Cambardella et al., Reference Cambardella, Delate and Jaynes2015) and can lead to lessen applications of water over the growing season. Additionally, cover crops help conserve water in cotton production in the southeastern USA (Vann et al., Reference Vann, Reberg-Horton, Edmisten and York2018).
In lieu of toxic synthetic defoliants used in conventional cotton, organic cotton boll maturation and defoliation is accomplished by water and nutrient management and defoliants permitted in organic production. Depending on climate and depth of stored soil water, irrigation can be terminated 4–5 weeks before final picking to facilitate defoliation. The absence of green leaf material is imperative at harvest since it increases moisture content, leaf trash and other impurities, and lowers fiber grade (Hutmacher et al., Reference Hutmacher, Vargas, Wright and Roberts2003).
Any discussion of the environmental footprint of organic cotton must include the extensive use of water in cotton processing and contamination of water resulting from the toxic chemicals used in cotton processing (Choudhury, Reference Choudhury and Muthu2017). The use of regulated processing aids in organic cotton processing, in accordance with the GOTS, helps protect water supplies from harsh chemical pollutants (GOTS, 2019).
Challenges to organic production: weeds, insects and diseases
Weeds were the top concern for most organic cotton growers. In addressing this concern, organic cotton growers use a variety of methods to manage weeds, including crop rotations (described below), tillage, hand-weeding and some organic herbicides, if not cost-prohibitive. These results mirror what Swezey et al. (Reference Swezey, Goldman, Bryer and Nieto2007) found that costs of production averaged 37% higher for organic when compared to conventional cotton production, primarily due to costs associated with hand-weeding.
Insect pests listed as a concern for organic cotton growers included Western Lygus plant bugs, spider mites, whiteflies, aphids, stink bugs and moths, though it was assumed ‘moths’ referred to lepidopteran larvae, which is usually controlled with Bt sprays.
Diseases were generally not cited as a problem in most cotton-growing regions likely due to resistant or tolerant varieties and crop rotations mitigating pathogen inoculum carry-over. Only one survey respondent in Texas reported problems with Fusarium and Rhizoctonia, which are common soilborne fungi that can attack cotton seedlings during cool, damp weather. Delaying planting until warmer soil conditions can mitigate these diseases, and they are not commonly reported on older plants, since cotton plants demonstrate more resistance to them with age (UC-ANR, 2019). Rotating to other crops, particularly non-hosts, is important to prevent Verticillium wilt, bacterial blight, damping off diseases and root-knot nematode management, and is a required organic practice.
Challenges to organic production: GM contamination and GM-free seed availability
In the USA, there has been a proliferation of ‘stacked’ GM traits conferring both herbicide tolerance (HT) and insect resistance from the soil bacterium, Bt. Since the introduction of GM cotton in 1996, the percent of cotton acreage grown with both HT-only and stacked-gene varieties increased from 2 to 91% by 2017 (USDA ERS, 2018).
Although organic cotton producers source and plant seed grown organically without the use of GM, episodes of GM contamination continue across the Cotton Belt (Donaldson, Reference Donaldson2015). Some of the causes of GM contamination have been reported to include the following: accidental use of GM seed; cross-pollination from GM crops; contamination from farm equipment; and accidental mixing during storage, transport or ginning (Textile Exchange, 2019).
This study found great concern around GM contamination of their organic cotton crop. Although none of the producers had been involved in any litigation over contamination, it is an on-going concern and is particularly noted as a risk during seed, ginning, hauling and the delinting process. One respondent mentioned cross-pollination with GM cotton as a concern. Although cotton has been reported to have a low level of cross-pollination (Reisig, Reference Reisig2018), cross-pollination with GM cotton can occur.
Twenty percent of producers and one processor noted awareness of resistance to Bt developing in Bt-cotton conventional fields. Bt resistance closely associated with the proliferation of Bt GM corn is especially concerning to organic farmers, because many organic farmers rely on naturally derived Bt as a form of pest control.
The concern over GM contamination is linked with pesticide contamination (see Challenges to organic production: climate, chemical drift and markets section below), as the increased prevalence of GM crops has been associated with an uptick in pesticide use on conventional crops, especially glyphosate, which can then drift to an organic field crop (Benbrook, Reference Benbrook2012).
There was a discrepancy between GM testing and GM presence, as reported by processors. Only one processor reported testing for GM presence in the organic cotton they processed, while the remainder rely on the veracity of the organic certificate or certification process to verify the GM-free status of the cotton. However, two out of five processors reported GM contamination in cotton they processed, ranging from 10 to 12% contamination. It was unclear if another entity determined contamination. This differs from organic food grain crops, which are routinely subjected to GM testing before purchasing and processing, and processors record the level of contamination for each load.
Contamination of organic cotton with any GM material is costly to organic growers. If GOTS-certified, a GM-positive test result would cause growers and other organic stakeholders to invest time and money to identify the cause of contamination, conduct additional tests and engage insurance companies and certifiers in order to remain compliant. In some cases, the product may be rejected and/or the organic land would need to undergo a 3-yr conversion period if the certifier determines an excessive contamination level, or revert to conventional production.
Challenges to organic production: sourcing seed
The majority (80%) of organic cotton producers reported that they were able to source organic seed stock. The 20% that had issues securing non-GM, organic-compliant seed did not specify the extent of their seed search, which may relate to their answers. Multiplication issues (e.g., easier access to organic seed) seem to be a worldwide concern (Textile Exchange, 2015). Oftentimes, organic producers must reach out to a number of companies before finding the specific organic variety they wish to plant. One producer reported the need for higher-yielding organic cotton varieties, citing lower organic yields compared to conventional counterparts. While research has shown similar yields for organic and conventional cotton (Swezey et al., Reference Swezey, Goldman, Bryer and Nieto2007), but, as is the case in other organic crops, weather can dramatically impact organic yields (Delate et al., Reference Delate, Cambardella, Chase and Turnbull2015). For example, if weed management does not proceed on in a timely fashion, due to rain and wet soils, yields can be decreased up to 20% of that harvested in normal years. More recent studies in India by Forster et al. (Reference Forster, Andres, Verma, Zundel, Messmer and Mäder2013) reported 14% lower yields in organic vs conventional cotton, but stability of organic yields in the face of detrimental weather, which may become more critical with worldwide climate change.
In addition, public plant breeding for true organic varieties, i.e., bred under organic conditions, is lacking, with only one plant breeder in Texas working on developing cotton varieties for organic producers. Efforts by Dr Jane Dever (Texas A & M University) include developing a cotton variety with elongated seed packets to help prevent physical mixing and contamination with conventional and GM-cotton.
Worldwide, there appears to be an interest in participatory plant breeding, where growers are involved with the cultivar selection process on their farms. The Seed, Integrity and Community Investment (SICI) program is investing in non-GM cotton seed in participatory breeding, multiplication programs and product traceability solutions (Textile Exchange, 2019). Participatory breeding may, or may not, speed the planting of higher-yielding varieties, as this has not yet occurred with participatory organic corn breeding programs, for example. Rather, success in developing varieties with specific quality traits has occurred in participatory breeding programs.
Challenges to organic production: climate, chemical drift and markets
Other challenges reported by survey respondents included the changing climate, citing lack of sufficient rainfall, hail, excessive rains and early season winds, and snow on the cotton crop before harvest. Efforts to deal with climate change, particularly through carbon-sequestering practices that build soil organic matter and retain soil moisture levels, can assist with drought mitigation (Franzluebbers et al., Reference Franzluebbers, Hubbs and Norfleet2012).
Other concerns facing organic cotton growers who responded to the survey included chemical drift from neighboring conventional fields and uncertain markets. Strict adherence to federal rules governing drift, and litigation in the case of non-compliance, could level the playing field with conventional producers. Unfortunately, current rules governing drift place the burden of minimizing contamination on organic producers. While some research has been conducted investigating methods for reducing GM contamination, including isolation, border rows and shifted planting dates, these can be costly for organic producers to implement and are not always effective, and 71% of organic farmers believe that federal regulations overseeing GM crop approvals are not adequate for protecting organic farm products (Hubbard, Reference Hubbard2019). For example, while the USDA has ample authority under the Plant Protection Act (USDA APHIS, 2000) to address genetic contamination of organic crops, the most recent USDA proposed rule on the ‘Movement of Certain Genetically Engineered Organisms’ gives a narrow interpretation of mandated authority, leaving regulatory decisions in the hands of agricultural biotechnology companies (USDA-AMS-NOP, 2019).
Market demand for cotton, in general, has been increasing, with world consumption in 2018/19 reaching 123.6 million bales, growing 0.9% from the previous year, to the highest level since 2007/08 (Dohlman et al., Reference Dohlman, Johnson, MacDonald, Meyer and Soley2019). Organic cotton production specifically has been growing, with a 56% increase in 2017–2018 to 831,193 bales, exceeding the previous year's growth rate of 10% (Textile Exchange, 2019). Organic cotton usage should increase, particularly if concerted efforts advertise the outstanding environmental, social and economic benefits of organic cotton for producers, farmworkers and consumers.
Future scenarios
Organic cotton will continue to raise the environmental bar for all cotton production. Many industry groups are aligned with this effort, under the auspices of the Organic Trade Association, Textile Exchange and GOTS. In December 2018, GOTS aligned with the Fashion Industry Charter for Climate Action, supporting the goals of the Paris Agreement, with the organic cotton industry aiming for achieving net zero emissions by 2050. Among the issues that will be addressed are a more carbon-neutral (or carbon-accumulating, if soil is involved) production phase, ‘selection of climate-friendly and sustainable materials, low-carbon transport, improved consumer dialogue and awareness, working with the financing community and policymakers to catalyze scalable solutions, and exploring circular business models’ (UNFCCC, 2018). If producers are supported for carbon-sequestering practices, such as crop rotations and cover crops, there is apt to be more conversion to organic production, as demonstrated for other crops (Singerman et al., Reference Singerman, Delate, Chase and Greene2011). It is incumbent upon universities, NGOs and industry groups to work together toward the goal of creating an organic cotton sector steeped in the principles of ecology, health, fairness and care (Rahmann et al., Reference Rahmann, Ardakani, Bàrberi, Boehm, Canali, Chander, David, Dengel, Willem Erisman, Galvis-Martinez, Hamm, Kahl, Köpke, Kühne, Lee, Løes, Moos, Neuhof, Nuutila, Olowe, Oppermann, Rembiałkowska, Riddle, Rasmussen, Shade, Sohn, Tadesse, Tashi, Thatcher, Uddin, von Fragstein und Niemsdorff, Wibe, Wivstad, Wenliang and Zanoli2016). A forward-looking research agenda could include participatory breeding efforts toward higher-yielding organic cotton varieties with specific characteristics that differentiate organic from conventional cotton. In addition, improved methods of organic weed management, such as flame or electric weeding that maintain soil quality, will also contribute to a more robust organic cotton industry in the USA. Finally, an in-depth analysis of current organic cotton marketing structures is needed, including the economic and social benefits of collective vs individual marketing, and methods to increase the number of cotton co-operative members. As members of the 27-yr-old Texas Organic Cotton Marketing Co-op note, cooperative efforts, including pooling production to level yield variation across sites, help mitigate risks and ensure a quality product for consumers (Hanson et al., Reference Hanson, Dismukes, Chambers, Greene and Kremen2004).
Acknowledgements
The authors would like to thank the many farmers and processors who participated in the survey and/or spoke with us directly about their experiences. We would also like to thank the Organic Trade Association Fiber Council for helping us collect information and connecting us with organic cotton producers and processors.
Financial support
This work was supported by a grant from The Organic Center.