Introduction
The Livestock sector has generated a significant attention in recent years due to its considerable environmental impact (van der Linden et al., Reference van der Linden, de Olde, Mostert and de Boer2020). Currently, it is estimated to be responsible for 14.5% of global greenhouse gas (GHG) emissions (FAO, 2018a) and 12% of water pollution (Mehrabi et al., Reference Mehrabi, Gill, van Wijk, Herrero and Ramankutty2020). Beef, like any food, generates undesirable emissions during its production stage. These emissions can harm water, air, soil, or wildlife in local ecosystems (O'Brien et al., Reference O'Brien, Herron, Andurand, Caré, Martinez, Migliorati, Moro, Pirlo and Dollé2020).
Yet it is important to recognize that grazing-based beef production provides a series of benefits to the environment and society worldwide, which are often not considered, including providing ecosystem services such as grassland protection and soil carbon conservation. Although agriculture occupies only 4% of the total ice-free land surface on our planet, livestock covers 26%, with grasslands accounting for 22%. The remaining land, which is unsuitable for human use, constitutes most marginal areas (FAO, 2018a; Mehrabi et al., Reference Mehrabi, Gill, van Wijk, Herrero and Ramankutty2020). Livestock production also contributes to the global economy, generating employment and income for around 1.3 billion people worldwide and providing livelihoods for 600 million poor smallholder farmers in developing countries (FAO, 2018b). Their food products, such as beef, provide proteins of high biological value and essential micronutrients to the population (Mehrabi et al., Reference Mehrabi, Gill, van Wijk, Herrero and Ramankutty2020), such as B complex vitamins (B12, B2, B3, and B6) and micro minerals such as iron, selenium, zinc, copper, and manganese. Regarding its lipid content, beef fat provides essential dietary energy and key nutrients such as essential fatty acids and fat-soluble vitamins A, D, and E (Cabrera and Saadoun, Reference Cabrera and Saadoun2014; Mwangi et al., Reference Mwangi, Charmley, Gardiner, Malau-Aduli, Kinobe and Malau-Aduli2019).
Increasing competition for natural resources and increased global demand for beef (1.2% annual growth until 2050) in low- and middle-income countries, driven primarily by human population growth, higher incomes, urbanization, and lifestyle changes, put significant pressure on the sector to develop more sustainably (FAO 2018b). It is necessary to identify and adopt mitigation strategies to address the environmental burden it generates, addressing positive and negative interactions (Angerer et al., Reference Angerer, Sabia, König von Borstel and Gauly2021; González-Quintero et al., Reference González-Quintero, Bolívar-Vergara, Chirinda, Arango, Pantevez, Barahona-Rosales and Sánchez-Pinzón2021; Mehrabi et al., Reference Mehrabi, Gill, van Wijk, Herrero and Ramankutty2020; O' Brien et al., Reference O'Brien, Herron, Andurand, Caré, Martinez, Migliorati, Moro, Pirlo and Dollé2020). Added to this are consumers' growing concern about the quality, nutrient content, and safety of the beef they consume as well as animal welfare standards and the environmental impact of production systems. Where beef is produced under pastoral systems, it is perceived as healthier and more respectful to the environment and animal welfare (Herron et al., Reference Herron, Curran, Moloney, McGee, O'Riordan and O'Brien2021). Therefore, as ecological, economic, and human health concerns increase, as well as interest in soil, water, climate change, and animal welfare, demand for grassland-raised livestock will continue to increase in developing and developed countries. There are a series of challenges and opportunities for these production systems to improve livestock production as well as ecosystem services, and animal and human health (van Vliet Provenza and Kronberg, Reference van Vliet, Provenza and Kronberg2021).
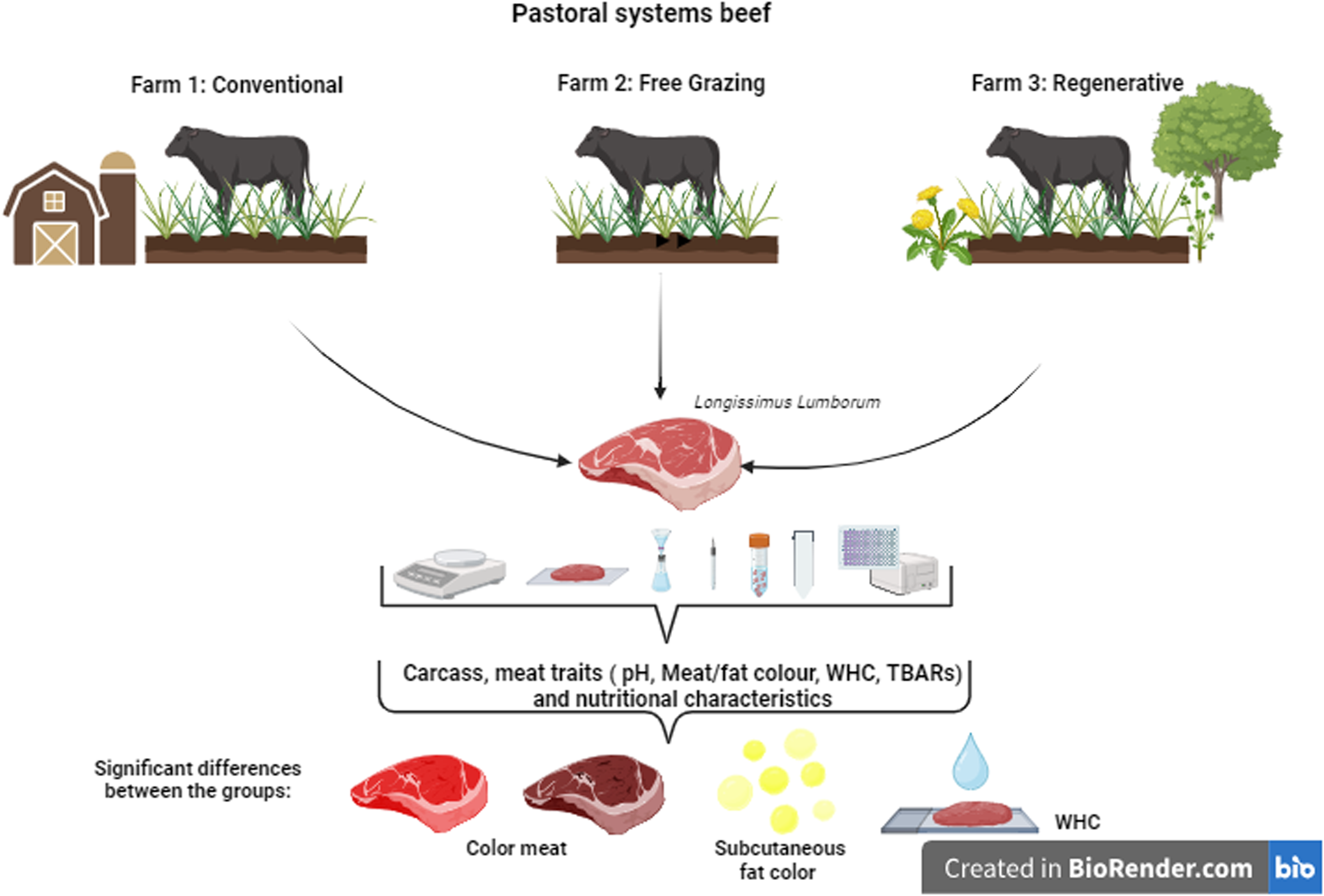
In this context, meat producers continually seek innovation and improvements in their systems to maximize profits and preserve the environment, increasing resource efficiency (Toro-Mujica, Reference Toro-Mujica2021), since conventional agricultural practices can cause soil degradation and a decrease in productivity (Khangura et al., Reference Khangura, Ferris, Wagg and Bowyer2023).
Over the past few years, regenerative livestock farming (RLF) has gained ground in response to the challenges posed by climate change and rising input costs. There are various descriptions of RLF, but there is still no agreed definition, which poses important challenges for all those who apply and understand the concepts of RLF, such as researchers, farmers, agricultural consultants, policymakers, and consumers (Khangura et al., Reference Khangura, Ferris, Wagg and Bowyer2023). However, according to Cusworth and Garnett (Reference Cusworth and Garnett2023), the definitions of RLF can be grouped into three (overlapping) types: 1. Those that emphasize a particular set of practices; 2. Those that focus on the desired or promised results; and 3. those who see regenerative agriculture as a new way of relating to each other and the natural world, as a way of thinking, going through a regenerative ‘journey’, which can be understood as the development from practices to results and attitudes.
RLF is a production system that aims to improve soil health and agricultural productivity and comply with the three pillars of sustainability: economic, environmental, and social. The RFL approach is grassland-based agriculture that reduces or eliminates the use of pesticides and herbicides; uses no-till agriculture; reduces or eliminates the use of fertilizers; uses AMP grazing (i.e., high intensity, short duration grazing, frequent paddock rotation, and long rest periods); and reduces or eliminates complementary feeding by destocking during the period of low primary vegetative productivity rather than operating at a fixed loading rate (Gosnell Charnley and Stanley, Reference Gosnell, Charnley and Stanley2020b). The net impact of these practices is expected to be an improvement in soil fertility, including increases in soil C, nitrogen, and phosphorus levels; improved system resilience to address climate change (including increased water infiltration, soil stability, and nutrient cycling); improvements in animal welfare; increases in biodiversity levels and an improvement in company profitability (Augustine et al., Reference Augustine, Derner, Fernández-Giménez, Porensky, Wilmer and Briske2020; Colley et al., Reference Colley, Olsen, Birkved and Hauschild2020; Gosnell Grimm and Goldstein, Reference Gosnell, Grimm and Goldstein2020a; Kleppel, Reference Kleppel2020).
In Chile, 72% of the bovine mass oriented to meat production is concentrated in the South where meat breeds are mainly composed of Angus and Hereford (Rojas-Cofre, Reference Rojas-Cofre2019). In these regions, the beef industry plays an important role in the economy, with over one million hectares of pastureland available for animal production. The country also possess a privilege sanitary status which make it possible to export meet products to North America, European Union and some Asian countries (Ibarra et al., Reference Ibarra, Rich, Adasme, Kamp, Singer, Atlagich and Mardones2018 and Morales et al., Reference Morales, Folch, Iraira, Teuber and Realini2012). The production systems are characterized by open-air grazing conditions, including in the diet preserved forage (Larraín and Vargas, Reference Larraín and Vargas2013). In southern Chile, the seasonality nature of grassland production and its fluctuating chemical composition throughout the year make it necessary the supplementation or transformation of feed through forage conservation or complement ingredients such as grains or industrial byproducts (González-Quintero et al., Reference González-Quintero, Bolívar-Vergara, Chirinda, Arango, Pantevez, Barahona-Rosales and Sánchez-Pinzón2021). In this way, a bovine meat production system prevalent in Southern Chile, referred to as ‘Conventional’ or ‘Traditional’, is characterized by free-range grazing for most of the animals’ lifecycle (from raising to rearing), with variations during the fattening stage, sometimes including supplementary feeding for the final phase. In recent years, new trends in grassland production systems and grazing management have emerged among producers and advisors in the country, including the adoption of RLF. However, the production protocols of RLF remain ambiguous. Further research is necessary to characterize and standardize the productive management practices establishing guidelines for uniform and sustainable productions. Additionally, this research will contribute to generating scientific information into the quality of Chilean beef, currently limited (Morales et al., Reference Morales, Folch, Iraira, Teuber and Realini2012).
In the context of generating a premium product of exceptional nutritional value, an extensive body of research has meticulously explored the inherent qualities of grass-based beef systems which harbor indispensable nutrients essential for promoting a healthy diet. Multiple studies conducted across Argentina, Brazil, Chile, and Uruguay have unequivocally demonstrated that beef derived from livestock raised within pasture-centric systems exhibits a discernibly lower proportion of intramuscular fat content (ranging from 1.6% to 3.6%) contrasted to meat from feedlot systems where concentrates constitute the principal dietary component (with fat content ranging from 3.18% to 7.65%) (Cabrera and Saadoun, Reference Cabrera and Saadoun2014). This nutritional discrepancy has been further underscored by the augmented presence of polyunsaturated fatty acids, including but not limited to linoleic acid (C18:2), linolenic acid (C18:3), eicosapentaenoic acid (C20:5, EPA), and docosapentaenoic acid (C22:5, DPA), imbuing the meat with appreciably reduced n-6 and n-3 fatty acid ratios in contrast to their counterparts from grain-finishing systems. This nutritional attribute is particularly salient considering the heightened scrutiny accorded to diets characterized by elevated n-6: n-3 ratios, which have been implicated as pivotal risk factors in the development of specific malignancies and coronary ailments (Cabrera and Saadoun, Reference Cabrera and Saadoun2014; Pordomingo et al., Reference Pordomingo, Grigioni, Carduza and Volpi Lagreca2012).
Furthermore, empirical evidence has substantiated the phenomenon that cattle nurtured on grass-based regimens can yield meat endowed with double the proportion of conjugated linolenic acid (CLA) compared to their concentrate-fed counterparts, registering levels of 0.53% to 0.25%, respectively (Realini et al., Reference Realini, Duckett, Brito, Dalla Rizza and De Mattos2004). Intriguingly, this trend extends to southern Chile, where steers reared on pasturage manifested CLA levels of 0.88% compared to the 0.7% exhibited by those subjected to concentrate-based diets (Morales et al., Reference Morales, Folch, Iraira, Teuber and Realini2012). Emerging research posits that meat from diverse botanical sources is poised to deliver elevated nutritional superiority, affording potential health advantages compared to beef sourced from intensive feedlot production systems (Butler et al., Reference Butler, Ali, Oladokun, Wang and Davis2021).
Quality attributes of beef must be thoroughly examined in the context of bovine meat production, including the coloration of beef and its tenderness. Some research suggests that extensive beef production systems may result in meat that exhibits a slightly darker complexion and marginally less tenderness when compared to beef from intensive production systems. However, it is crucial to acknowledge that certain studies have not provided consistent evidence to support these observed differences (Santos et al., Reference Santos, Monteiro, Voss, Komora, Teixeira and Pintado2021). Therefore, more research is needed on beef quality and nutritional value produced in different pastoral systems and its potential relationship with generating a higher-quality product.
According to this background, it is observed that grassland-based systems can generate a healthier meat product. In recent years, several studies have focused on showing the differences in meat quality from pastoral systems vs those animals fed with concentrates under controlled environments. However, there are no studies in Chile for cattle that evaluate meat quality from different pastoral systems, which consider real farm production data and products from livestock companies. Based on the background presented, this study aimed to explore some factors that influence the quality of beef from three pastoral livestock systems in southern Chile, such as carcass characteristics, instrumental parameters (pH, meat color and subcutaneous fat, capacity of water retention, lipid oxidation), and nutritional value, exposing attributes of each one for different traits.
Materials and methods
The study was carried out in three full-cycle commercial beef cattle farms that used the Angus breed, located in the Regions of La Araucanía and Los Ríos (Farm 1: Conventional, Farm 2: Free Grazing, and Farm 3: Regenerative) (Fig. 1), and data were collected between 2022 and 2023. All three farms breed Aberdeen Angus cattle within a holistic production system. These are commercial farms with different production strategies, so this study is completely empirical.
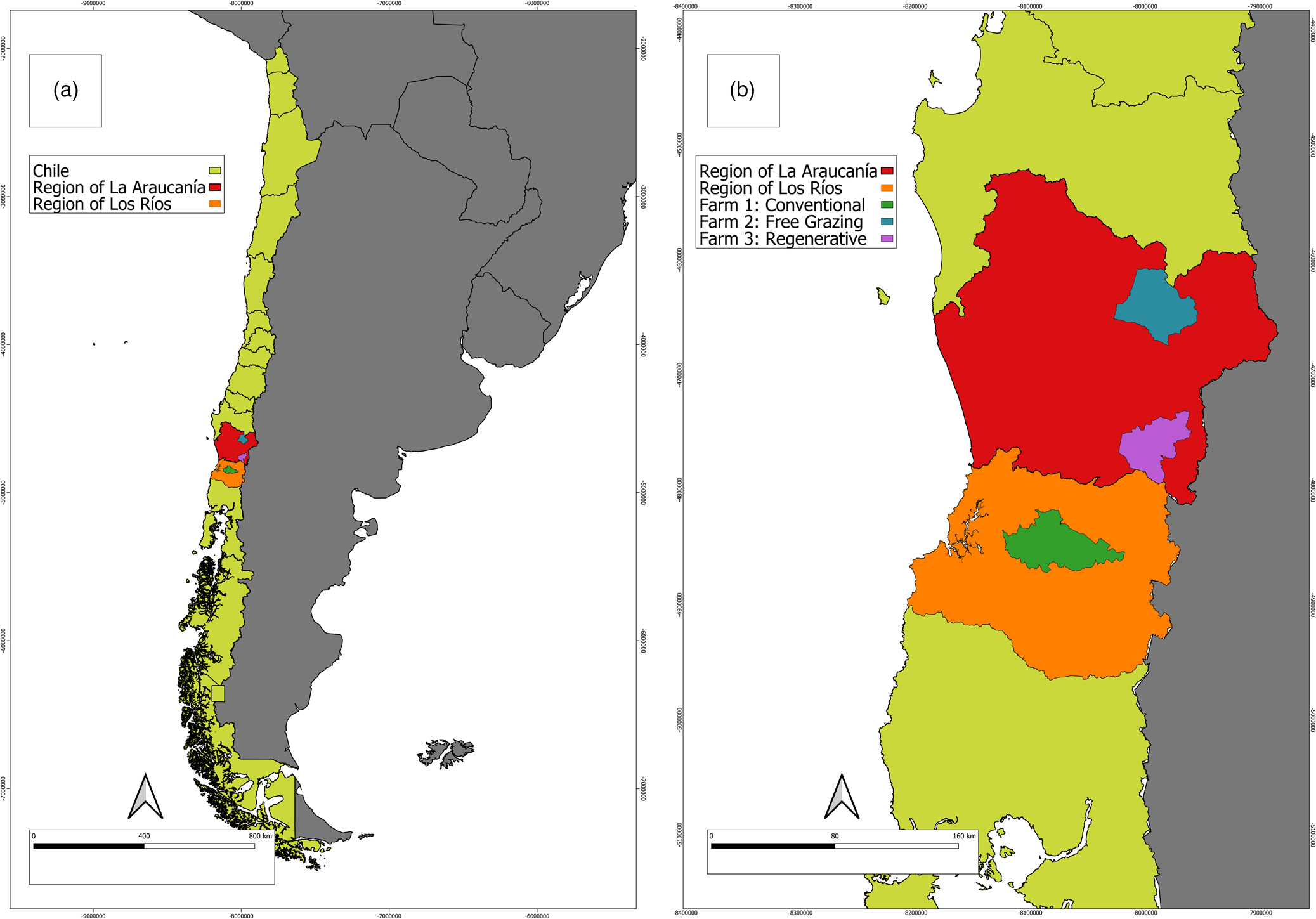
Figure 1. Geographic location on the map of Chile of the livestock systems under study.
Characterization Of the production systems
Farm 1: conventional (G1)
This is a highly technical system of traditional agronomic management. The feed base for cattle is prairie under rotational grazing. The fattening stage occurs under permanent confinement in sheds, obtaining animals for slaughter between 18 and 20 months. According to soil analysis results and consultant recommendations, chemical fertilizers are used on pastures and supplementary crops. In addition, manure from the fattening is used as organic fertilizer. No-tillage is used in the cultivated pastures. Regarding reproductive management, it uses artificial insemination, review bulls, and heat synchronization, and to diagnose pregnancy, rectal palpation and ultrasonography are used. The main animal losses are due to neonatal deaths due to attacks by predators (vultures and fearl dogs). The farm is officially free of tuberculosis, brucellosis, and enzootic bovine leukosis. The main business is the sale of fat animals.
Farm 2: free grazing (G2)
This is a technical system of traditional agronomic management. The feed base for cattle is prairie under rotational grazing. The fattening stage takes place under free grazing but with supplementation (meadow silos and concentrate). The production cycle is slightly shorter, and animals are slaughtered at 18 months. Chemical fertilizers are used on pastures and supplementary crops (oats, triticale). Tillage is used in grasslands. This system stands out for the genetic quality of its herd. It performs genetic improvement activities such as phenotypic selection of males and females, artificial insemination (AI), and embryo transfer (ET). Regarding reproductive management, it uses AI, review bulls, heat synchronization, TE, and ultrasonography to diagnose pregnancy. The farm is not officially free of tuberculosis, brucellosis, and enzootic bovine leukosis. The main business is the sale of fat animals through livestock marketing fairs. The direct sale of black Angus breeding bulls also stands out.
Farm 3: regenerative (G3)
This production system uses agronomic management based on holistic principles, with a high level of technology in the irrigation system of its meadows. The feed base for the cattle is the naturalized grassland under planned holistic grazing, which involves the rotation of livestock between multiple paddocks to distribute the pressure across the landscape of the farm, incorporating short grazing intervals with relatively high animal population densities and more extended recovery periods (Park et al., Reference Park, Ale, Teague and Jeong2017). The complete production cycle is carried out under direct grazing and in the open air. Fat animals are available for slaughter between 18 and 20 months. No agrochemicals are used in its meadows, and zero tillage is practiced. Regarding reproductive management, natural mating and artificial insemination at a fixed time are used only in heifers; ultrasonography is used to diagnose pregnancy. The main animal losses are due to abortions and neonatal deaths due to stress, mainly caused by the daily movement of the herd. The farm is officially free of tuberculosis, brucellosis, and enzootic bovine leukosis. The main businesses are the sale of fat animals and specialized brand meat.
Table 1 below shows a summary of the main characteristics of the production systems.
Table 1. Main characteristics of the livestock systems under study according to their property management and marketing systems

Each farm rears calves, fattens, and finishes steers, as shown in Table 2 (Calf-to-finished steer nutritional progression chart for the conventional, free grazing, and regenerative systems).
Table 2. Calf-to-finished steer nutritional progression chart for conventional, free grazing and regenerative systems
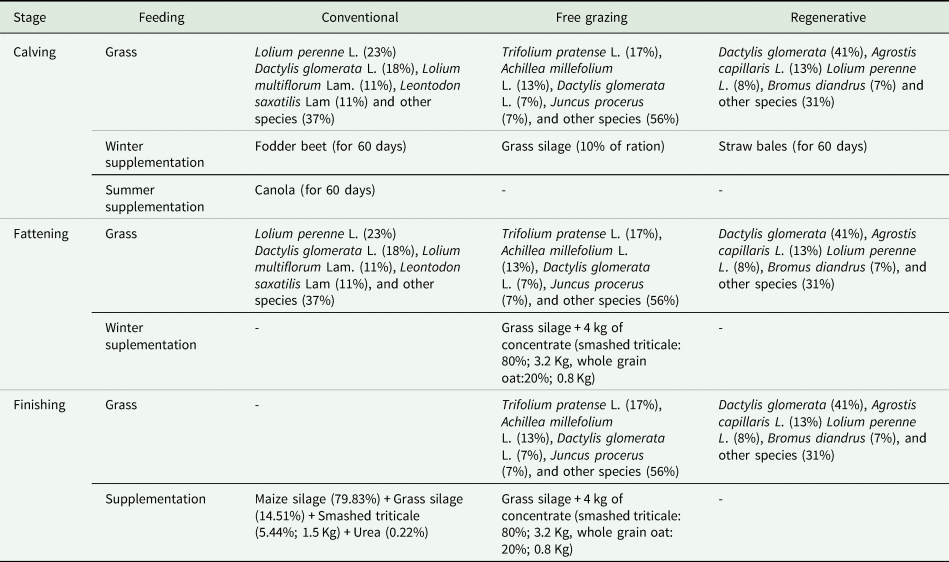
Meat Quality
The steers were fed on pastures throughout their lives in G2 (n:108), G3 (n:240), and G1 (n:280) and were confined in sheds for the last 100 days, with differences in feeding during fattening. The botanical composition was dominated by grasses in G1, mainly Lolium perenne L (23%), Dactylis glomerata L. (18%) and Lolium multiflorum Lam (11%), in G2 legumes such as Trifolium pratense L. predominated (17%), and other herbs such as Achillea millefolium L (13%), G3 presented a polyphyletic meadow, highlighting species Dactylis glomerata (41%), Agrostis capillaris L. (13%) Lolium perenne L. (8%), Bromus diandrus (7%) and Trifolium pratense L. (5%).
The steers were slaughtered in commercial slaughterhouses between 18 and 20 months of age. Twelve meat samples were obtained from each Longissimus lumborum system (~300 g) at the level of the 12th rib between February and April 2023 and transported to the laboratory under controlled temperature conditions. The physical-chemical analyses were conducted 7 days after slaughter in the Meat Technology and Innovation Center laboratory of the Universidad de La Frontera. An ISFET IQ puncture pH meter (Hanna Meat pH meter, model: HI98163) was used to measure pH (6 replicates) for meat samples from each steer. To measure meat color in the eye area of the loin and subcutaneous fat, a portable CR-10 colorimeter was used through the CIE L*a*b* system (luminosity (L*), redness (a*) and yellowness (b*)), respectively, in six random locations (Konica Minolta Color Rader, model: CR-10). The Water Retention Capacity (CRA) was analyzed through the compression method (percentage of juice expelled per sample). Lipid oxidation was determined by the process of thiobarbituric acid reactive substances (TBARs) described by Vyncke (Reference Vyncke1975), recording the absorbances at 532 nm and 600 nm using a BioTek Epoch 2 Microplate Spectrophotometer. Data on carcass characteristics, such as hot rod weight (PVC) and yield (RC), were obtained from slaughter reports.
Statistical analysis was performed with a simple Analysis of Variance test (ANOVA) with Tukey's post hoc test (p < 0.05), which was conducted to highlight the differences in traits both within and across the three different systems. This analysis was done using IBM SPSS Statistics 23 software (IBM Corporation, 225 Somerset, New York). Results were expressed as mean ± standard deviation. Finally, representative samples of Longissimus lumborum from each system were sent to a certified food quality laboratory (DICTUC, Chile). The proximate chemical composition of the meat was determined according to standard methods of the Association of Official Analytical Chemists (AOAC, Reference A.O.A.C1990). The energy content is calculated using the Atwater calculation (Mayxard, Reference Mayxard1944). Total carbohydrates will be determined by calculation using the difference. The moisture content of the meat samples was analyzed according to the Chilean standard 841.of78 (INN 2018). The mineral compositions of meat samples were determined using AOAC methods. The zinc value was determined through the procedures established by AOAC 968.08, iron based on AOAC 999.11, and selenium content based on AOAC 986.15. Total lipids were determined according to the Folch Lees and Stanley (Reference Folch, Lees and Stanley1957) technique in the CTI Carne laboratory of the University of La Frontera.
Results
Carcass Traits:
According to the data obtained during the slaughter of Angus steers, the fat cover of the carcasses was similar between the three production systems (~1) and without significant differences (P > 0.05). For hot carcass weight and carcass yield, significant differences were detected between the three groups (P < 0.05), obtaining higher values for the free grazing system (313.58 kg and 58. 69%, respectively) (Table 3).
Table 3. Summary table of the weights and gains during the fattening of the steers, carcass measurements, and characteristics of the Longissimus lumborun beef from the three pastoral systems under study (Conventional, Free grazing, and Regenerative)

a*, redness; b*, yellowness; C*, chroma; L*, lightness; h*, tone.
P value with an asterisk (*) indicates significant differences between types of pastoral systems (P < 0.05).
Meat Quality attributes:
Longissimus lumborum pH:
There was no significant difference in pH between the three farming systems (P > 0.05). The pH of the meat samples averaged 5.5 for the three production systems (Table 3). However, slight variations were observed in their measurement ranges: 5.30–5.79 for the conventional, 5.25–6.08 for the free grazing, and 5.38–5.66 for the RLF system.
Color:
Significant color differences (P < 0.05) were observed between the meat samples from the three production systems, as obtained by colorimetry using the CIELab system. The redness (a*) and yellowness (b*) were higher for the Conventional System (18.46 and 14.87, respectively). The luminosity (L*) was lower for the regenerative system (35.22). Regarding the color of the subcutaneous fat, significant differences (P < 0.05) were obtained for all color parameters between the production systems. Yellowness (b*) was greater for the free grazing system at 21.55, followed by the regenerative system at 19.57 (Table 3).
Water holding capacity:
Water retention capacity was significantly different between the three production systems (P < 0.05, table 3), with the highest value reported for the conventional system (32.53%).
Lipid oxidation (TBARs):
The results of this study did not show significant differences between the means of the three production systems for the thiobarbituric acid reaction (TBARs). However, the free grazing system presented a greater standard deviation between its measurements (Table 3).
Nutritional characteristics of Longissimus lumborum
The results of the nutritional composition of the longissimus lumborum cut from each production system are presented in Table 4. The energy, fat, and protein content were higher for the conventional system sample (254.60 Kcal/100 g, 3.29 g/100 g, and 22.00 g/100 g, respectively), and the sodium level was also higher for this system (75. 00 mg /100 g). Regarding the meat sample from the Regenerative System, a higher content of ash and selenium stands out (0.86 g/100 g and 0.28 mg/100 g). Higher values are presented in the conventional system (1.97 and 3.97 mg/100 g) for iron and zinc.
Table 4. Nutritional characteristics of Longissimus lumborum sample from Conventional, Free grazing, and Regenerative pastoral systems
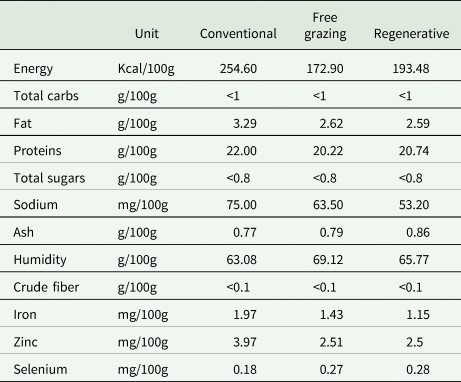
Discussion
The Results related to carcass characteristics indicated some differences among the three production systems (P < 0.05) for hot carcass weight (HCW) and carcass yield (CY), with higher values obtained for the free grazing system (313.58 kg PVC and 58.69% RC respectively). These differences might have been influenced by multiple factors, such as the use of anabolic agents during rearing and fattening in the free grazing system, where studies reveal that HCW was higher in steers receiving implants compared to non-implanted steers (Webb et al., Reference Webb, Block, Harty, Salverson, Daly, Jaeger and Blair2020). Another influential factor could be the destination marketing system, where animals passing through a market remained fasting for longer, leading to higher CY due to ruminal emptying effects. Additionally, the genetic quality of Angus cattle in this system is noteworthy, attributed to the increased utilization of assisted reproductive technologies (artificial insemination, embryo transfer), which enhances cattle's quality and production efficiency (Lamb et al., Reference Lamb, Mercadante, Henry and DiLorenzo2016).
In controlling the technological quality of meat, pH plays a very important role as it largely determines shelf life, processability, and water retention capacity. Post mortem, glycolysis decreases the pH of meat from 7 to approximately 5.5. This occurs due to the cessation of oxidative metabolism in cells, leading to anaerobic consumption of glycogen and the accumulation of lactic acid. Of course, this process only occurs if the animal's muscle glycogen reserves were normal and sufficient at the time of death (Clinquart et al., Reference Clinquart, Ellies-Oury, Hocquette, Guillier, Santé-Lhoutellier and Prache2022). In this study, the mediation of Ph in the meat samples showed an average of 5.5 for the three production systems without significant differences (P > 0.05). However, greater variations were observed in the pH measurement ranges for the free grazing System (5.25–6.08). This could be attributed to the increased stress experienced during handling before slaughter, which impacts meat quality through muscle glycogen depletion (Hultgren et al., Reference Hultgren, Segerkvist, Berg, Karlsson, Öhgren and Algers2022).
The color of meat is one of the most influential visual appearance traits in consumer purchasing decisions (Tomasevic et al., Reference Tomasevic, Djekic, Font-i-Furnols, Terjung and Lorenzo2021). The bright cherry-red color of fresh beef is commonly associated with freshness and wholesomeness, and any deviation from this appearance results in consumer rejection and economic losses. The color of fresh meat is influenced by many intrinsic factors (muscle source, muscle fiber type, and breed) and extrinsic factors (animal management and diet). Concerning extrinsic factors, diet is a critical pre-slaughter factor that influences the susceptibility of meat to oxidative deterioration (Salim et al., Reference Salim, Ferrari, Monteiro and Mano2022a). The present study observed significant color differences among the meat samples from the three production systems. The redness (a*) was higher in the conventional system (18.46), which is consistent with other studies, such as the meta-analysis conducted by (Lanari et al., Reference Lanari, Brewster, Yang and Tume2002). They investigated the impact of feed during the final fattening phase, comparing grass-fed steers to grain-fed steers in feedlot conditions, focusing on color parameters of the longissimus lumborum muscle. The study documented those muscles from grain-fed animals exhibited greater redness (a*) compared to their grass-fed counterparts. The yellowness (b*) was also higher for the conventional system (14.87), attributable to its final fattening diet. Studies indicate that grain-fed animals' longissimus muscles show a slight tendency towards greater yellowness than grass-fed animals. These differences in b* values observed in the longissimus muscles of grain-fed animals vs grass-fed ones could be partially attributed to a higher intramuscular fat content typically observed in grain-fed animals (Luciano et al., Reference Luciano, Moloney, Priolo and Monahan2011; Patino et al., Reference Patino, Medeiros, Pereira, Swanson and McManus2015; Salim et al., Reference Salim, Ferrari, Monteiro and Mano2022). Regarding the luminosity of the color of the meat, the results indicated a lower luminosity (L*) for the Regenerative System (35.22), as reflected in the study conducted by Salim et al. (Reference Salim, Ferrari, Monteiro and Mano2022), where it is indicated that meat luminosity (L*) is influenced by diet, as meat from grain-fed animals exhibited higher L* values (4.01%) than their grass-fed counterparts. These observed differences in luminosity could be attributed to changes in muscle metabolism due to enzymes in grass-fed animals. Jurie et al. (Reference Jurie, Ortigues-Marty, Picard, Micol and Hocquette2006) evaluated the influence of feeding (grazing pasture vs. maize silage) on Charolais steer muscles. This author observed that the increase in oxidative enzymes (e.g., ß-hydroxyacyl-CoA dehydrogenase and isocitrate dehydrogenase) in the meat of grass-fed cattle could alter the glycolytic pattern of muscles, making glycolytic muscles like the longissimus more oxidative, resulting in a lower L* value.
The color of subcutaneous bovine adipose tissue (carcass fat) depends on various factors (age, sex, and breed). However, diet is the most important extrinsic factor, but its influence depends on the duration of each type of feeding. Cattle produced under extensive pasture-based systems generally have carcass fat that is yellower than their intensively raised and concentrate-fed counterparts, and this is caused by carotenoids from green forage (fresh grass and preserved forage) (Dunne et al., Reference Dunne, Monahan, O'Mara and Moloney2009). The main responsible pigment is β-carotene and, to a lesser extent, lutein (Strachan Yang and Dillon, Reference Strachan, Yang and Dillon1993). This was evidenced in the results, where the two pastoral systems ending in grassland obtained the highest values of yellowness (b*), 21.55 for free grazing and 19.57 for the regenerative system.
Another important quality attribute in meat products is the water retention capacity, which influences the product's performance, has economic implications, and is important in terms of food quality (BertramKarlsson and Andersen, Reference Bertram, Karlsson and Andersen2003; Rosenvold and Andersen, Reference Rosenvold and Andersen2003). Several pre- and post-mortem factors influence the water-holding capacity (WHC) of the meat. The genotype and diet of meat animals during growth and development are important due to their direct influence on muscle characteristics. In the pre-slaughter period, stresses on the animal, such as fasting and different stunning methods, are likely to influence the WHC of the meat. Post-slaughter, cooling, aging, and injection of non-meat ingredients influence WHC (Cheng and Sun, Reference Cheng and Sun2008). According to our results, the WHC was lower for the free grazing system (0.25 grams), and it could be explained because these animals went through a higher degree of stress before slaughter, as they went through a marketing fair before slaughter; this factor is especially critical for their final meat quality (Bertram et al., Reference Bertram, Schäfer, Rosenvold and Andersen2004). Pre-slaughter stress produces lactic acid, reducing muscle pH even when cattle are still alive (Henckel et al., Reference Henckel, Karlsson, Oksbjerg and Petersen2000). For decades, it has been recognized that the pH within the first hour's postmortem is important for the WHC of meat due to protein denaturation (Bertram et al., Reference Bertram, Stødkilde-Jørgensen, Karlsson and Andersen2002). Despite the absence of discernible differences in the pH between the groups, it is worth mentioning that the free grazing system exhibited individual ranges of pH values comparatively higher than the rest of the groups evaluated.
The perception of redness in beef is an important factor in consumer preferences and acceptance of beef because of a perception of freshness (Altmann, Trinks and Mörlein, Reference Altmann, Trinks and Mörlein2023). According to a study, meat stored for longer periods was initially perceived by consumers as less red and then also declined more rapidly during display. The redness of beef is due to a protein called myoglobin, which transports oxygen in the cells. As the temperature of the meat increases, the myoglobin darkens, and at well-done levels, myoglobin becomes metmyoglobin, giving the meat a gray color (Corlett et al., Reference Corlett, Pethick, Kelman, Jacob and Gardner2021). Myoglobin, the principal pigment responsible for the color of meat, is water-soluble. Consequently, excessive water loss leads to meat discoloration (Apple and Yancey, Reference Apple and Yancey2013). This phenomenon is evident in the findings of the present study, where the group conventional with greater WHC also exhibits higher values for redness and lightness.
The main non-microbial factor responsible for the deterioration of the quality of meat foods is oxidative damage, which generates quality losses during storage and display in retail markets (Faustman et al., Reference Faustman, Sun, Mancini and Suman2010; Hur Park and Joo, Reference Hur, Park and Joo2007). In meat, the oxidative stability of lipids depends on the balance between antioxidant and prooxidant components of the muscle. Muscle antioxidants include endogenous antioxidant systems and molecules of dietary origin, such as tocopherols and carotenoids (Descalzo and Sancho, Reference Descalzo and Sancho2008). On the contrary, polyunsaturated fatty acids are highly oxidizable substrates and can act as pro-oxidants (Morrissey et al., Reference Morrissey, Sheehy, Galvin, Kerry and Buckley1998). The inherent susceptibility of meat lipids to oxidative deterioration can be significantly affected by the diet that animals receive by modifying the antioxidant and pro-oxidant components of the muscle (Pouzo et al., Reference Pouzo, Descalzo, Zaritzky, Rossetti and Pavan2016). This study found no significant differences in TBAR values among the three groups. However, it should be noted that farms that mainly depend on grazing exhibited lower values (0.27 and 0.28 mg MDA/kg for the free grazing and regenerative systems, respectively). This observation could be attributed to the animals' access to more antioxidants than a conventional system. Grass forages provide vitamin E, other natural antioxidants, and a higher proportion of long unsaturated fatty acids, which tend to oxidize. In addition, the concentration of antioxidants from pastoral systems (Free-Range and Regenerative) could be good enough to also compensate for the peroxidation that high PUFA levels could generate in the muscle (Pouzo et al., Reference Pouzo, Descalzo, Zaritzky, Rossetti and Pavan2016).
Regarding the nutritional quality attributes of beef, it is known to provide a wide range of essential macro and micronutrients, in particular proteins of high biological value, heme iron, zinc, and vitamins B3, B6, and B12. However, the concentrations of some nutrients vary between animals and cuts, about the metabolic type of the muscle (heme iron, vitamin B12), or the composition of the muscle (lipids, zinc) (BauchartChantelot and Gandemer, Reference Bauchart, Chantelot and Gandemer2008; Clinquart et al., Reference Clinquart, Ellies-Oury, Hocquette, Guillier, Santé-Lhoutellier and Prache2022).
Several studies demonstrate that feeding or finishing grass/forage significantly reduces total carcass fat content compared to grain-finished cattle (Van Elswyk and McNeill, Reference Van Elswyk and McNeill2014), as systems that rely on grain finishing maximize availability of net energy and glucose for fat synthesis as muscle growth declines in older animals, thus contributing to higher fat content than can be achieved by feeding or grass finishing (Scollan et al., Reference Scollan, Hocquette, Nuernberg, Dannenberger, Richardson and Moloney2006), which is demonstrated in our study, where the conventional system obtained higher values of total fat (3.29 g/100 g) vs the systems finished in grassland. This higher fat content in the conventional system would also explain the higher energy content of the samples, as meat fat is a calorie-dense macronutrient with a key role in providing essential nutrition and maintaining a healthy body weight (Vannice and Rasmussen, Reference Vannice and Rasmussen2014). In general, the fat content in the Longissimus lumborum samples for the three systems under study (3.29, 2.62, and 2.59 g/100 g, conventional, free grazing, and regenerative, respectively) were similar to those obtained by Morales et al., Reference Morales, Folch, Iraira, Teuber and Realini2012 and Larraín and Vargas, Reference Larraín and Vargas2013, which demonstrate that these production systems produced it is low-fat meat and that it could be classified as extra lean according to the Food Health Regulations (Larraín and Vargas, Reference Larraín and Vargas2013).
Diet is a factor that modifies the nutritional profile of beef (Pighin et al., Reference Pighin, Pazos, Chamorro, Paschetta, Cunzolo, Godoy and Grigioni2016). Feeding cattle with grass or grain diet can alter the micronutrient content of beef (Scollan et al., Reference Scollan, Dannenberger, Nuernberg, Richardson, MacKintosh, Hocquette and Moloney2014; García et al., Reference García, Pensel, Sancho, Latimori, Kloster, Amigone and Casal2008; Krusinski et al., Reference Krusinski, Maciel, Sergin, Goeden, Schweihofer, Singh and Fenton2022). The highest levels of selenium are notable in both 100% pastoral systems (Free Grazing and Regenerative, 0.27 and 0.28 mg/100 g, respectively), consistent with prior research indicating elevated selenium content in beef sourced from pastoral systems in South America (Cabrera et al., Reference Cabrera, Ramos, Saadoun and Brito2010; Ramos Cabrera and Saadoun, Reference Ramos, Cabrera and Saadoun2012; Cabrera, Ramos and Saadoun, Reference Cabrera, Ramos and Saadoun2013). In a comparative investigation conducted in Uruguay, meat from grass-fed Aberdeen Angus steers contained more selenium than steers fed only a concentrate-based diet (Cabrera, Pereiro, and Saadoun, Reference Cabrera, Pereiro and Saadoun2013), as also presented in this study. The differences in selenium concentrations in meat likely result from a combination of different production practices, a concentrated diet, and the availability of traces of mineralized salt in the final stage.
Furthermore, the selenium content and species of both plant and animal foods depend on environmental conditions, particularly the amount and species of selenium to which the animal/plant is exposed (Cabrera and Saadoun, Reference Cabrera and Saadoun2014). Other factors such as botanical species, selenium species, soil chemical and physical characteristics such as pH, soil texture, organic matter content, and the presence of ions such as SO2− 4 and PO3−4 also influence the absorption of Se by plants (Mikkelsen, Page and Bingham, Reference Mikkelsen, Page and Bingham1989). Although grass species could affect the level of selenium in beef, few reports reveal more information about the natural sources of selenium in grassland-based production systems (Cabrera and Saadoun, Reference Cabrera and Saadoun2014). Concerning zing values, it is recognized that beef is one of the richest sources of highly bioavailable zinc, which could guarantee adequate levels of the recommended daily dose (Cabrera and Saadoun, Reference Cabrera and Saadoun2014). Studies in Uruguay show that meat from pasture-raised cattle contains a slightly lower level of zinc (2.3–3.7 mg/100 g of fresh meat) than other meats previously published in reports (Cabrera et al., Reference Cabrera, Ramos, Saadoun and Brito2010). The low level of zinc observed in the samples from the three systems under study could be explained by the reduced level of micronutrients in the grasslands of southern Chile (Campillo et al., Reference Campillo, Urquiaga, Undurraga, Pino and Boddey2005).
Finally, beef from pasture-raised steers exhibits distinctive organoleptic properties compared to meat produced in grain-based confined systems. When the primary diet consists predominantly of high-quality forage from temperate pastures, the fat content of the meat can be reduced, and the composition of its fatty acids can be improved. In pastoral diets, the chemical components present in the lipid fraction may influence the taste and color of the meat, with particular relevance to those associated with human health. However, meat quality is not solely defined by organoleptic characteristics but also by the composition of meat and fat, emphasizing their significance in aspects related to human health (Rearte Reference Rearte2022). All these outcomes are influenced by numerous factors inherent to the uniqueness of farms that make them almost incomparable to each other. This impacts the final product and underscores the imperative to establish standardized protocols for finishing animals to achieve a consistent end product with consumer acceptability and sustainability.
Conclusions
The Intricate interplay of various factors within diverse pastoral systems impacts the carcass and meat quality. A holistic approach encompassing all these influences is imperative to formulate a comprehensive model to achieve consistent, uniform products characterized by stability across all attributes over time. This is particularly pertinent in southern Chile, where a plethora of distinct criteria governs the finalization of steers. Consequently, this diversity of factors contributes to a discernible heterogeneity among the resultant products available in the market. This heterogeneity manifests in the nuanced attributes of the meat, including but not limited to color, redness, water-holding capacity, and other sensory characteristics, thereby exerting a tangible impact on consumers' perceptual experience. Consuming meat from animals primarily fed high-quality forage from pastures reduces fat content. The chemical components present in the lipid fraction of pastoral diets play a crucial role in influencing the taste and color of the meat, with notable implications for human health. While organoleptic characteristics contribute significantly to meat quality, it is essential to recognize the interplay between the composition of both meat and fat. This underscores the importance of considering not only sensory attributes but also the nutritional aspects in assessing the overall quality of beef, thereby emphasizing the potential health benefits associated with pasture-raised systems. Addressing and managing these complexities is paramount for enhancing the marketability and consumer satisfaction associated with beef products from the region. Therefore, to improve and complement future research, it is necessary to include the evaluation of other quality attributes of beef, such as the consumer's perspective regarding the factors most valued and used in their purchasing decision to predict quality, such as price, designation of origin/brands/certification, appearance/color of the meat, presentation, and visible fat. Without a holistic view of the production and marketing of livestock products, the generation of healthy products is incomplete.
Institutional review board statement
The animal study protocol was approved by the Universidad de La Frontera's Institutional Ethics Committee (protocol code N°154_01; November 4th, 2021).
Acknowledgements
C.V. would like to thank the Dirección de Postgrado, Programa de Doctorado en Ciencias Agroalimentarias y Medioambiente, Dirección de Investigación, Universidad de La Frontera and National Doctoral Grant ANID N°21200347.
The authors are members of the Healthy Meat network, funded by CYTED (ref.119RT0568)
Funding
This research was supported by the Agencia Nacional de Investigación y Desarrollo (ANID) under Grant National Doctoral N°21200347 and funded (partially) by the University of La Frontera, Doctoral Thesis Support Program.
Disclaimer/Publisher's note
The statements, opinions, and data contained in all publications are solely those of the individual author(s) and contributor(s) and not of MDPI and/or the editor(s). MDPI and/or the editor(s) disclaim responsibility for any injury to people or property resulting from any ideas, methods, instructions, or products referred to in the content.