Vitamin D, known mainly for its role in Ca homeostasis, is now increasingly being recognized for its important function as a hormone with immunomodulatory effects on various physiological and pathological processes( Reference Nagpal, Na and Rathnachalam 1 , Reference Papandreou, Malindretos and Karabouta 2 ). Vitamin D deficiency can cause rickets in children and osteomalacia in adults. In addition, long-term vitamin D deficiency in youth can also increase the risk of common cancers, autoimmune disorders, hypertension and infectious diseases later in life( Reference Papandreou, Malindretos and Karabouta 2 , Reference Taylor, Lucas and Dear 3 ).
The concentration of 25-hydroxyvitamin D (25(OH)D) in the blood is regarded as the best indicator of vitamin D status, because it is quantitatively related to the supply of vitamin D over preceding weeks( Reference Hollis 4 ). Although there is as yet no consensus about the threshold level of 25(OH)D that indicates vitamin D deficiency in children, most experts now agree that vitamin D deficiency should be defined as a 25(OH)D concentration of <20 ng/ml. Vitamin D insufficiency is currently recognized as a 25(OH)D concentration of 21–29 ng/ml( Reference Bischoff-Ferrari, Glovannucci and Wilett 5 – Reference Zerwekh 7 ). Nowadays, vitamin D insufficiency and deficiency is an unrecognized epidemic and a common health problem worldwide, the prevalence of which has been reported to range from 15 to 80 %( Reference Holick 8 – Reference Weng, Shults and Leonard 11 ).
As with vitamin D deficiency, anaemia and elevated blood lead levels (BLL) are also remarkable nutritional problems for children in China. The role of vitamin D in erythropoiesis has been suggested by several clinical observations. In the haemodialysis population, vitamin D repletion has been associated with dose reductions in erythrocyte-stimulating agents and increased reticulocytosis( Reference Albitar, Genin and Fen-Chong 12 , Reference Saab, Young and Gincherman 13 ). Vitamin D has been demonstrated in bone marrow to affect marrow function( Reference Norman 14 ). Despite these intriguing observations, there is an overall paucity of clinical studies in children investigating whether adequacy of vitamin D affects blood Hb levels.
As a ubiquitous metal, Pb has been confirmed to have toxic effects on multiple tissues and organs and also to be neurotoxic upon exposure to a lower level (<10 μg/dl)( Reference Lanphear, Hornung and Khoury 15 , Reference Chandramouli, Steer and Ellis 16 ). However, studies on relationships between Pb and serum 25(OH)D are few; most were done more than two decades ago when BLL were much higher (≥29 μg/dl) and have yielded inconsistent results( Reference Box, Cherry and Waldron 17 – Reference Kemp, Neti and Howell 21 ). Meanwhile, some recent studies have found that the vitamin D receptor (VDR) involved in Ca metabolism interacts with Pb and that polymorphisms in VDR can affect 25(OH)D levels and Pb toxicokinetics( Reference Li, Shi and Yang 22 , Reference Rezende, Barbosa and Montenegro 23 ). Hence, with the improvement of the environment and prevention measures for Pb exposure, a new relationship between vitamin D and BLL that is lower than in the past should be established.
Therefore, in the present study we investigated concentrations of serum 25(OH)D, Hb and blood Pb in healthy children living in south-west China, in order to evaluate the prevalence of vitamin D deficiency and the relationship between vitamin D deficiency, anaemia and elevated BLL in children.
Materials and methods
Study design
The present study was performed from January to December in 2011. Each healthy child aged from 6 months to 14 years who was taken to a physical examination at West China Second University Hospital, Sichuan University was recruited. The hospital is the largest special hospital for women and children in south-west China, and is located in Chengdu, Sichuan (latitude 30·67°N). Children with chronic illness that could affect the level of vitamin D, such as gastrointestinal, liver or renal diseases, or who consumed vitamin D were excluded, as were any with haemoglobinopathies. Demographic data of each child, including age, race and home address, were obtained by medical record. All blood samples of children were collected by sophisticated paediatric phlebotomists.
This project was approved by the ethics committee of West China Second University Hospital, and all parents or guardians signed a consent form agreeing for their child to participate in the study.
Laboratory tests
All tests were conducted in a clinical laboratory that has been accredited by ISO15189 since 2006. Fasting venous blood samples of all children were obtained after a 30-min rest in a sitting position. Three blood samples, each containing 3 ml of blood, were collected from each child to measure 25(OH)D, Hb and Pb levels. The blood was collected in a vacuum tube containing separation gel for 25(OH)D, in a vacuum tube containing anticoagulant (EDTA tripotassium salt, EDTA-K3) for Hb and in a vacuum tube containing heparin for Pb.
Serum 25(OH)D concentration was detected by chemiluminescent immunoassay using the Liaison total assay (DiaSorin Inc., Stillwater, MN, USA), and control material (Bio-Rad, Hercules, CA, USA) was used for quality control to ensure the accuracy of measurement of 25(OH)D concentrations every day. Hb was detected using the Sysmex XE-2100 haematology analyser (Sysmex Corporation, Kobe, Japan) with the homologous calibrator, reagent kits and quality control processes provided by the manufacturer. Blood Pb was tested by flame atomic absorption spectroscopy using a BH2100-type, tungsten boat, atomic absorption spectrometer (Beijing Bohui Innovation Technology Co. Ltd, Beijing, China). Daily internal quality control was done using Seronorm™ Trace Elements whole blood quality control (LOTMR9607; Sero AS, Billingstad, Norway).
Definitions
Serum 25(OH)D concentration <20 ng/ml was defined as vitamin D deficiency, while <30 ng/ml was defined as insufficiency according to the criteria most experts recommend( Reference Bischoff-Ferrari, Glovannucci and Wilett 5 – Reference Zerwekh 7 ). Anaemia was defined using WHO criteria as Hb < 11·0 g/dl for ages 0·5–4·99 years, <11·5 g/dl for ages 5–11·99 years and <12·0 g/dl for ages 12–14 years( 24 ). Elevated BLL was defined as Pb ≥10 μg/dl, conforming to current WHO guidelines( 25 ). However, some studies have provided evidence of Pb neurotoxicity even at levels <10 μg/dl( Reference Lanphear, Hornung and Khoury 15 , Reference Chandramouli, Steer and Ellis 16 ), and the US Centers for Disease Control and Prevention has recently revised its level to 5 μg/dl for monitoring children. So we created a category that we called ‘mildly elevated BLL’, defined as Pb level ≥5 μg/dl but <10 μg/dl, as a more sensitive measure of elevated risk.
Statistical analysis
All data were analysed using the statistical software package IBM SPSS Statistics version 17·0 and included descriptive statistics, univariate linear regression, Pearson's product–moment correlation and binary logistic regression. The χ 2 test, t test and one-way ANOVA were also performed to investigate the differences between children with vitamin D sufficiency, insufficiency and deficiency status, respectively. In the different models, BLL and Hb were used as a continuous variable and as a categorical outcome. For binary logistic regression, the data set was split into vitamin D sufficiency and non-sufficiency parts to analyse the association between 25(OH)D deficiency and risk factors, including child demographics, Hb and BLL. For all of the analyses, P values were two-tailed with α < 0·05 considered statistically significant.
Results
A total of 1218 children aged from 6 months to 14 years were recruited in our study, including 721 boys (59·2 %) and 497 girls (40·8 %). Mean level was 33·18 (sd 16·48) ng/ml for 25(OH)D, 5·63 (sd 1·9) μg/dl for Pb and 12·35 (sd 3·61) g/dl for Hb. The prevalences of 25(OH)D insufficiency and deficiency were 27·4 % and 21·6 %, respectively, which were higher than those for anaemia at 8·5 % and elevated BLL at 1·8 %, but lower than that for mildly elevated BLL at 56·9 %. Serum 25(OH)D decreased and blood Pb and Hb levels increased with age (Table 1). There was no significant difference in each parameter (25(OH)D, Hb, Pb) between boys and girls (all P > 0·05). After univariate linear regression analysis, 25(OH)D, Hb and Pb concentrations each showed a significant association with age.
Table 1 Serum 25(OH)D, blood lead and Hb levels according to age group and gender among 1218 children aged from 6 months to 14 years, south-west China, 2011
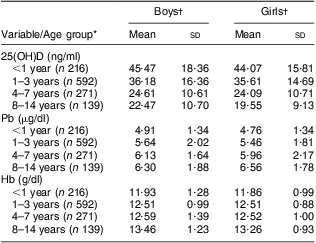
25(OH) D, 25-hydroxyvitamin D.
*Univariate linear regression between (i) age and vitamin D: r 2 = 0·194, t = −17·116, P < 0·001; (ii) age and Pb: r 2 = 0·052, t = 8·182, P < 0·001; (iii) age and Hb: r 2 = 0·005, t = 2·618, P = 0·009.
†Difference in mean value between boys and girls (t test): vitamin D, P = 0·972; Pb, P = 0·113; Hb, P = 0·24.
Some differences were noted when child demographics, season of sampling, Hb and BLL were compared between the three groups (deficiency, insufficiency, sufficiency) divided by serum 25(OH)D concentration (Table 2). Gender and season of blood sampling showed no significant difference between the three groups, while age was strongly associated with 25(OH)D status. The percentage of 25(OH)D insufficiency and deficiency in children aged 4–7 years and 8–14 years was as high as 76·8 % (208/271) and 81·3 % (113/139), respectively, being approximately two to four times higher than that in children aged <1 year and 1–3 years. There was a statistically significant relationship between 25(OH)D status and BLL. The proportion of children with lower BLL (<5 μg/dl) in the 25(OH)D sufficiency group was more than that in the other two groups (61·4 % v. 24·4 % or 14·1 %, P < 0·001). Furthermore, mean Pb level in the group with 25(OH)D sufficiency was significantly lower than in the other groups (P = 0·013 and P < 0·001). There was no statistically significant association between 25(OH)D status and Hb concentration in the present study.
Table 2 Demographic characteristics, season of sampling and laboratory variables according to vitamin D status among 1218 children aged from 6 months to 14 years, south-west China, 2011

25(OH)D, 25-hydroxyvitamin D.
*Spring = March to May; summer = June to August; autumn = September to November; winter = December to February.
†Anaemia was defined as Hb < 11·0 g/dl for ages 0·5–4·99 years, < 11·5 g/dl for ages 5–11·99 years and <12·0 g/dl for ages 12–14 years.
‡Data are presented as mean and standard deviation.
§The χ 2 test was performed to test the differences between variables; P < 0·05 was considered statistically significant.
||ANOVA was performed to test the differences between variables; P < 0·05 was considered statistically significant.
The correlations between 25(OH)D and Hb, and between 25(OH)D and BLL, are demonstrated in Fig. 1. There was no statistically significant relationship between 25(OH)D and Hb (r = −0·012, P = 0·669). Furthermore, the vast majority of children with 25(OH)D deficiency and insufficiency had a normal Hb level (546/597, 91·5 %). In contrast, a significant negative correlation was determined between 25(OH)D and BLL (r = −0·216, P < 0·001). Pearson's product–moment correlation showed that there was no significant correlation between BLL and Hb (r = −0·023, P = 0·437).

Fig. 1 The correlations between vitamin D status, measured as serum 25-hydroxyvitamin D (25(OH)D) level, and Hb and blood lead levels (BLL) among 1218 children aged from 6 months to 14 years, south-west China, 2011. (a) Two-way scatterplot of vitamin D status and Hb level shows there is no correlation (r = −0·012, P = 0·669); (b) two-way scatterplot of vitamin D status and BLL shows a negative correlation (r = −0·216, P < 0·001)
To further confirm the relationship between 25(OH)D concentration and Hb or BLL, multivariate logistic regression was performed controlling for age, gender and season of blood sampling (Table 3). After adjustment, those children with 25(OH)D deficiency and insufficiency had a higher odds of being 8–14 years of age (OR =18·89; 95 % CI 10·14, 32·99; P < 0·001) and having elevated BLL (OR = 1·01; 95 % CI 1·00, 1·02, P = 0·045). However, it was seemed there was still no certain association of Hb level with 25(OH)D deficiency and insufficiency (OR = 0·52; 95 % CI 0·97, 0·99; P = 0·062).
Table 3 Multivariate logistic regression model for vitamin D deficiency and insufficiency (25(OH)D < 30 ng/ml) in 1218 children aged from 6 months to 14 years, south-west China, 2011

25(OH)D, 25-hydroxyvitamin D; Ref., reference category.
*Spring = March to May; summer = June to August; autumn = September to November; winter=December to February.
Discussion
The present study provided data on the prevalence of 25(OH)D deficiency and the correlations of 25(OH)D with anaemia and BLL in a large, regionally representative sample of children in the target age range of 6 months to 14 years old.
Our study confirmed a higher prevalence of 25(OH)D deficiency (21·6 %) and insufficiency (27·4 %) than of either anaemia or elevated BLL among south-west Chinese children. The prevalence of vitamin D deficiency was reported to be 3·1 % to 57·8 % in Chinese adolescents, 0·1 % in Japanese children aged 1–4 years, 29·8 % in Korean children aged less than 2 years and 24 % in Mongolian children younger than 1 year old( Reference Fraser 26 , Reference Yoon, Park and Seo 27 ). These data for Asian children as study population are comparable to our outcomes. Our findings show that there was no significant difference in 25(OH)D concentration between the genders of children, but there was an increasing risk of vitamin deficiency and insufficiency in older age groups, which is consistent with the previous studies( Reference Kumar, Muntner and Kaskel 28 , Reference Absoud, Cummins and Lim 29 ). That may be because of lower vitamin D intake by the older children, who were undergoing a rapid growth period while not receiving an adequate diet, unlike the infants and toddlers being breast-fed or receiving powdered formulas with balanced nutrient content. With increased understanding of the profound role of vitamin D in growth, development and other physiological progress( Reference DeLuca 30 , Reference Dusso, Brown and Slatopolsky 31 ) such as cellular proliferation and differentiation, immune system modulation and so on, the recommendations have been revised to include all infants, those who are exclusively breast-fed and older children and adolescents, in order to ensure their adequate vitamin D levels( Reference Wagner and Greer 32 ). Nowadays, a new guideline of the American Academy of Pediatrics is that all infants, children and adolescents have a minimum daily intake of 10 μg (400 IU) of vitamin D beginning soon after birth and this replaces the previous recommendation of a minimum daily intake of 5 μg (200 IU) of vitamin D supplementation beginning in the first 2 months after birth and continuing through adolescence( Reference Wagner and Greer 32 ).
In our study we did not find a relationship between 25(OH)D and anaemia, which is similar to results by Abdul-Razzak et al. ( Reference Abdul-Razzak, Khoursheed and Altawalbeh 33 ) and Kersey et al. ( Reference Kersey, Chi and Cutts 34 ) However, an association between vitamin D deficiency and a greater risk of anaemia and lower Hb was demonstrated by Sim et al.( Reference Sim, Lac and Liu 35 ). The study subjects of Sim et al. were adults and 65 % of them had chronic kidney disease, which is different from our study. In addition, elevated BLL, as an important factor to influence anaemia, might suppress Hb production. For that reason we also tested this possible interaction in our sample. However, we found that BLL had no correlation with Hb in children, which may due to the low Pb levels in our sample because, according to the US Centers for Disease Control and Prevention, Pb does not begin to suppress Hb until it reaches 25–40 μg/dl( 36 ).
Different from anaemia, a negative correlation between blood Pb and 25(OH)D was found in our study. Previous studies had found elevated BLL would cause anorexia( Reference Papanikolaou, Hatzidaki and Belivanis 37 ), which reduced dietary intakes of Ca and vitamin D. Meanwhile, experimental studies in rats have demonstrated that Pb can decrease serum 1,25-dihydroxyvitamin D levels due to the toxic effects of Pb on the kidney( Reference Smith, DeLuca and Tanaka 38 , Reference Daggett, Oberley and Nelson 39 ). Thus, it is possible that elevated BLL is a cause of the reduction in serum 25(OH)D. However, the correlation coefficient of 25(OH)D and blood Pb was not great, which may be attributed to the following reasons. First, genetic polymorphism of VDR has been associated with susceptibility to Pb and vitamin D deficiency in some studies; e.g. the VDR FokI (rs2228570) f genotype was associated with high 25(OH)D level and low blood Pb( Reference Li, Shi and Yang 22 , Reference Rezende, Barbosa and Montenegro 23 , Reference Schwartz, Stewart and Kelsey 40 – Reference Santos, Mascarenhas and Satler 42 ), which indicates that genetic features are not to be ignored. Second, some studies in animals have demonstrated that relationships among Pb, dietary Ca and vitamin D are complex and in some cases counterintuitive, and therefore are not predictable based solely on theoretical considerations( Reference Bogden, Gertner and Christakos 43 – Reference Fullmer 45 ). Thus, the significant negative association we found between blood Pb and serum 25(OH)D in the present study may not represent a causal relationship and the underlying mechanisms should be researched in the future.
There are some limitations in our study. First, the dietary intake and status of essential nutrients, such as supplement Ca, Fe and vitamin D, were not investigated in the children in our study, which may have influenced the correlation between 25(OH)D and other parameters. In addition, blood samples in different seasons were collected from different children rather than paired from the same one, which may weaken the effect of season on Pb and vitamin D levels. Another potential limitation is that the Han population accounts for 95·7 % (1166/1218) of our study subjects. However, race is a key factor that affects blood Pb and vitamin D nutrition, as well as their interactions( Reference Kemp, Neti and Howell 21 ). Hence, our findings may not be generalizable to other populations outside Han children.
Conclusion
We found the presence of 25(OH)D insufficiency and deficiency to be more common than either anaemia or elevated BLL in children in south-west China, with age appearing to be a key factor influencing BLL and vitamin D nutrition. In addition, 25(OH)D deficiency and insufficiency in children was associated with elevated BLL while lacking a significant relationship with anaemia. Therefore, our study showed that there is some relationship between vitamin D status and age-related accumulation of Pb in children; if this finding could be confirmed by further study, it could have important public health implications.
Acknowledgements
Source of funding: This research received no specific grant from any funding agency in the public, commercial or not-for-profit sectors. Conflicts of interest: There is no conflict of interest. Authors’ contributions: L.C. and X.L. contributed equally to this paper and should be considered as joint first authors. L.C. and X.L. did data collection, statistical analysis and paper writing; H.S., W.D. and H.W. conducted the laboratory tests of 25(OH)D, Hb and blood Pb; Y.J. was the designer and manager of the study.