- EI
energy intake
There is good evidence that dietary SFA increase the concentrations of serum LDL-cholesterol, an identified risk factor for CVD and particularly CHD(Reference Zock, Williams and Buttriss1). Whilst in general SFA raise total cholesterol and LDL-cholesterol, early studies have identified that individual fatty acids have markedly different effects. In particular, myristic (14:0) and palmitic (16:0) acids have been associated with elevated serum LDL-cholesterol concentrations in human subjects(Reference Katan, Zock and Mensink2, Reference Temme, Mensink and Hornstra3) whilst the other major SFA in foods, stearic acid (18:0), has been shown to be essentially neutral(Reference Bonanome and Grundy4). Some studies suggest that lauric acid (12:0) and 14:0 have more potent effects on serum LDL-cholesterol than 16:0 whilst others suggest that 14:0 and 16:0 are more potent than 12:0(Reference Gurr5). Much of the 12:0, 14:0 and 16:0 in the human diet is derived from milk fat(Reference Padley, Gunstone, Harwood, Gunstone, Harwood and Padley6), and therefore the consumption of milk and dairy foods would be expected to have adverse effects on serum LDL-cholesterol levels. A recent meta-analysis of sixty selected studies of human subjects has confirmed that when dietary carbohydrates are replaced with an isoenergetic amount of 12:0–16:0 SFA an increase in LDL-cholesterol occurs, but the study crucially notes that there is also an increase in the protective HDL-cholesterol(Reference Mensink, Zock, Kester and Katan7). It has been argued that the total cholesterol:HDL-cholesterol provides the most powerful predictor of the effect of dietary fatty acids on risk of CHD with low values being associated with reduced risk(Reference Mensink, Zock, Kester and Katan7). This interpretation indicates that the effects of 12:0 and 14:0 fatty acids may be somewhat beneficial as they both reduce the total cholesterol:HDL-cholesterol whilst the opposite is the case for 16:0. It has been concluded that overall the risk of CHD would be most effectively reduced by the replacement of dietary SFA with either cis-MUFA or PUFA(Reference Mensink, Zock, Kester and Katan7). The benefits in relation to cholesterol profile of the two replacement strategies are similar.
The replacement of SFA by both cis-MUFA and PUFA not only brings about favourable changes in plasma cholesterol pools but may have other beneficial outcomes. Whilst most attention has focused on the hypercholesterolaemic effect of SFA and associated increases in CVD risk, there is now some evidence that high intakes of SFA may also be related to reduced insulin sensitivity, which is a key factor in the development of the metabolic syndrome(Reference Nugent8). In epidemiological studies high intakes of SFA have been associated with a higher risk of impaired glucose tolerance and higher fasting plasma glucose and insulin concentrations(Reference Feskens and Kromhout9–Reference Feskens, Virtanen, Rasanen, Tuomilehto, Stengard, Pekkanen, Nissinen and Kromhout11). A 3-month intervention study involving 162 healthy subjects given diets rich in SFA (from butter and margarine) or cis-MUFA (from high-oleic sunflower oil) showed that the subjects on the SFA diet had significantly impaired insulin sensitivity (−10%) whilst those on the cis-MUFA diet showed no change(Reference Vessby, Uusitupa and Hermansen12) (Table 1). Also of note in this study is that additional dietary inclusion of n-3 fatty acids from fish oil had no effect on insulin sensitivity or insulin secretion and the favourable effects of the cis-MUFA diet were not seen in individuals with a high fat intake (>37% energy intake (EI)). Against these benefits there have been concerns that a high cis-MUFA intake is often accompanied by an increase in both factor VII activation and plasminogen activator inhibitor type-1 activity, which are risk factors for CHD(Reference Miller13). However, in addition to the effects on blood lipid profiles and other aspects of the CVD phenotype, there is emerging evidence that cis-MUFA may also have some anti-cancer benefits. For example, in vitro studies suggest that oleic acid may be involved in inhibiting the growth of breast cancer cells(Reference Menendez, Vellon, Colomer and Lupu14) and it is of note that in the Greek cohort of the European Prospective Investigation into Cancer and Nutrition Study the only single dietary characteristic of the Mediterranean diet shown to be significantly protective to a range of cancers was MUFA:SFA, with increased values being beneficial(Reference Benetou, Trichopoulou, Orfanos, Naska1, Lagiou1, Boffetta and Trichopoulos15).
Table 1. Effect of challenge with SFA or cis-MUFA on insulin variables, plasma glucose and serum lipids in healthy men and women (from Vessby et al. (Reference Vessby, Uusitupa and Hermansen12))
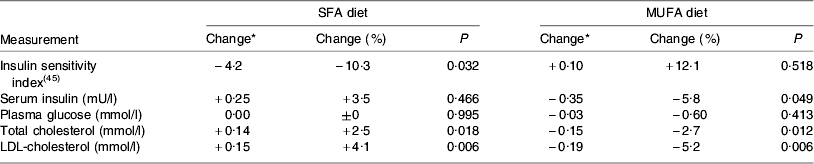
* Mean change during treatment expressed as least square mean.
Despite the recognised benefits of limiting SFA intake, it has been reported that in the UK men and women currently exceed the Department of Health mean population target for SFA intake (<11% total food energy(16)) by 22 and 20% respectively(Reference Henderson, Gregory, Irving and Swan17) and indeed few countries within the EU achieve such a target(Reference Hulshof, van Erp-Baart and Anttolainen18). The proportion of UK children exceeding the target is even greater(Reference Gregory, Lowe, Bates, Prentice, Jackson, Smithers, Wenlock and Farron19). Whilst on average UK adults do not achieve the population target for cis-MUFA intake (13% total food energy(16)), deviation from the target is much less than that for SFA, with cis-MUFA intakes of 12·1 and 11·5% EI for men and women respectively(Reference Henderson, Gregory, Irving and Swan17). There is, however, still considerable scope for improvement.
Milk and dairy products as sources of saturates
A comprehensive study across fourteen western European countries has shown that milk and dairy products are key sources of total fat (24%) and the single largest source of SFA (39%) in the UK diet(Reference Hulshof, van Erp-Baart and Anttolainen18) (Fig. 1). More recently, the UK National Diet and Nutrition Survey has confirmed the primary role of milk and dairy products as sources of dietary SFA(Reference Henderson, Gregory, Irving and Swan17). Thus, conceptually, a simple strategy to reduce intake of SFA would therefore be to advise that consumption of milk and dairy products should be substantially reduced. This approach, however, would ignore the fact that these foods also supply up to approximately 30% of dietary cis-MUFA in a number of EU countries(Reference Hulshof, van Erp-Baart and Anttolainen18) and are key sources of other key nutrients including vitamin B12 and Ca(Reference Givens, Kliem and Paquin20).
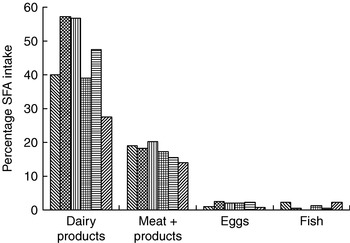
Fig. 1. Contribution of animal-derived foods to intake of SFA in selected European countries. (), Iceland; (
), Germany; (
), France; (
), UK; (
), Italy; (
), Greece. (Adapted from Hulshof et al. (Reference Hulshof, van Erp-Baart and Anttolainen18).)
There is also good epidemiological evidence that increased consumption of milk can provide cardiovascular protective benefits. Based on an analysis of ten cohort studies the reported risks in subjects with the highest intakes of milk, relative to those with the lowest consumption, were 0·87 (95% CI 0·74, 1·03) for CHD and 0·83 (95% CI 0·77, 0·90) for ischaemic stroke(Reference Elwood, Pickering, Hughes, Fehily and Ness21). Also, the high milk consumers had a reduced incidence of the metabolic syndrome(Reference Elwood, Pickering and Fehily22). Thus, simply reducing milk and dairy consumption in order to reduce SFA intake is not likely to produce benefits overall.
Manipulating the fatty acid composition of dairy foods to replace some SFA with cis-MUFA may therefore prove a valuable means of reducing SFA intake whilst retaining the cardioprotective benefits of milk. This hypothesis is supported by two intervention studies with such modified milk and milk products(Reference Noakes, Nestel and Clifton23, Reference Seidel, Deufel and Jahreis24). A summary of the main findings from these two studies is shown in Table 2. In the first of the studies, which used an 8-week randomised cross-over design with thirty-three men and women, diets containing normal dairy products with 65 and 23% fatty acids as SFA and cis-MUFA respectively were compared with diets in which the fat in the dairy products had been modified to contain 50% SFA and 35% cis-MUFA(Reference Noakes, Nestel and Clifton23). Broadly similar percentages of SFA and cis-MUFA were used in the second study, which involved thirty-one men and women with three sequential intervention periods each of 18 d(Reference Seidel, Deufel and Jahreis24). Whilst the latter study used both subjects who were normocholesterolaemic and hypercholesterolaemic(Reference Seidel, Deufel and Jahreis24), it is of note that the control values for total cholesterol and LDL-cholesterol for the subjects in the first of the studies(Reference Noakes, Nestel and Clifton23) were somewhat higher than those for the hypercholesterolaemic group of the second study(Reference Seidel, Deufel and Jahreis24). Despite this difference, overall responses were greater in the second study(Reference Seidel, Deufel and Jahreis24), which also interestingly reports a more significant (P>0·01) increase in HDL-cholesterol (+22%) in the normocholesterolaemic group than in the hypercholesterolaemic group (+6%; P>0·05). This finding suggests benefits of the modification in subjects generally regarded as normocholesterolaemic. Serum concentrations of LDL-cholesterol were significantly decreased in both studies whilst HDL-cholesterol was increased only in the second study(Reference Seidel, Deufel and Jahreis24), leading to a decreased LDL-cholesterol:HDL-cholesterol. In the second study the dietary manipulation resulted in reduced concentrations of lipoprotein (a), leading the authors to conclude that modifying the milk fat composition has positive effects on both LDL-cholesterol:HDL-cholesterol and lipoprotein (a) concentrations, both of which have been established as risk factors for CHD(Reference Seidel, Deufel and Jahreis24). Based on the observed reduction (−4·3%) in total cholesterol, it was proposed that this outcome could lead to a 9% reduction in death from CHD(Reference Noakes, Nestel and Clifton23).
Table 2. Effect of consuming dairy products with reduced SFA and increased cis-MUFA on serum cholesterol (chol), TAG and lipoprotein (a) in all participating subjects in two studies(Reference Noakes, Nestel and Clifton23, Reference Seidel, Deufel and Jahreis24) (mmol/l except where stated)
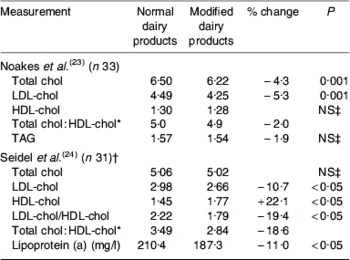
* Not presented by authors.
† Subjects who were normo-and hypercholesterolaemic together.
‡ P>0·05.
Whilst these two studies do provide support for the concept of modifying milk fatty acid composition in the way described, it is noteworthy that both studies involved small numbers of participants, were of short duration and had only a limited number of outcomes measured with no estimates of insulin sensitivity or vascular function included(Reference Noakes, Nestel and Clifton23, Reference Seidel, Deufel and Jahreis24). More detailed and longer-term studies are urgently needed.
Modifying milk fatty acid composition
Decreasing the SFA content of milk
The main technique used to decrease the SFA content of milk fat is to include supplements of plant oils or oilseeds rich in unsaturated C18 fatty acids in the diet of the dairy cows (for review, see Givens & Shingfield(Reference Givens, Shingfield, Williams and Buttriss25)). Specifically, this approach can be used to reduce the proportion of SCFA and medium-chain fatty acids (6:0–16:0) and increase the concentrations of long-chain fatty acids in milk(Reference Grummer26, Reference Doreau, Chillard, Rulquin, Demeyer, Garnsworthy and Wiseman27). These changes are believed to occur as a result of long-chain fatty acids (C≥16) inhibiting the de novo fatty acid synthesis of short- and medium-chain SFA in the mammary gland, and because lipid supplements increase the amount of long-chain unsaturated fatty acids in plasma available for incorporation into milk fat. Normally, including plant oils (other than palm oil rich in 16:0) in the cows' diet has little effect on milk fat content of 4:0 but consistently increases 18:0 concentrations at the expense of 16:0(Reference Palmquist, Beaulieu and Barbano28, Reference Chillard, Ferlay, Mansbridge and Doreau29). In all cases, inclusion of plant oils and oilseeds in the diet of the cow leads to an unavoidable increase in milk trans-18:1 content as a result of extensive lipolysis and biohydrogenation of C18 PUFA in the rumen.
Increasing the cis-MUFA content of milk
As result of extensive metabolism of dietary unsaturated fatty acids, 18:0 is the most abundant long-chain fatty acid available for incorporation into milk fat. However, the secretion of cis-9 18:1 in milk is in excess of mammary 18:1 uptake as a result of the activity of stearoyl-CoA (Δ9) desaturase activity in mammary secretory cells. Desaturation of 18:0 to cis-9 18:1 is the predominant precursor–product of the Δ9 desaturase, converting approximately 40% of 18:0 uptake by the mammary gland(Reference Chillard, Ferlay, Mansbridge and Doreau29). It is therefore feasible to exploit the endogenous desaturation in the mammary gland to enhance the cis-MUFA (18:1 in particular) content of milk fat by supplementing diets with lipids rich in 18:0, although this approach does not normally alter the cis-9 18:1/18:0(Reference Chillard, Ferlay, Mansbridge and Doreau29). However, feeding plant oils or oilseeds rich in cis-9 18:1 can be used to enhance milk fat cis-9 18:1 content. Care is needed to ensure that as far as possible such material is protected from rumen microbial biohydrogenation, thus providing maximum enhancement of cis-MUFA in milk fat whilst minimising the concentrations of trans-18:1 in milk(Reference Givens, Shingfield, Williams and Buttriss25). Supplements of cis-9 18:1 acyl amides(Reference Jenkins30, Reference Loor, Herbein and Jenkins31) or high levels of whole cracked rapeseeds in the diet(Reference Givens, Allison and Blake32) have been shown to dramatically increase the cis-9 18:1 content of milk fat, although this approach can be associated with some reduction in voluntary diet intake and hence milk yield. With a rapeseed diet 18:1 in milk fat increased from 20·1% total fatty acids to 41·3% total fatty acids, although the rather extreme treatment diets used were associated with reduced milk production(Reference Givens, Allison and Blake32). This approach would therefore need to be balanced by a premium paid for milk of altered fatty acid composition.
Modelling the impact of changes in milk composition on the risk of CVD at EU population level
As part of the Lipgene project(33) a series of studies have been undertaken to produce milk with reduced SFA (typically from 70% total fatty acids to 55% total fatty acids) and increased cis-MUFA (typically from 20% total fatty acids to 32% total fatty acids). The background to this modification has been discussed(Reference Givens34) but essentially it has been achieved by the use of oleic acid-rich rapeseeds in the diet of the dairy cow. A number of models based mainly on changes in the concentration of serum cholesterol pools has been proposed to assess the impact of changes in the amount and composition of dietary lipids on the risk of CHD and CVD in general. Within the Lipgene project(33) these models have been used to develop five approaches to assess the impact at EU population level of reducing SFA and increasing cis-MUFA content of milk fat. Lack of data currently prevents such modelling of the effects on insulin sensitivity or the metabolic syndrome as a whole.
Baseline data employed
The intakes of SFA and cis-MUFA by males and females in eleven EU member states as reported in the TRANSFAIR study(Reference Hulshof, van Erp-Baart and Anttolainen18) were used to represent current baseline intakes. Similarly, data for the percentage of total intake of these fatty acids derived from milk fat were also taken from the same study. These baseline data are summarised in Table 3. The effect on intake of these fatty acids in each of the eleven EU member states was calculated assuming that all milk and dairy products consumed had SFA reduced from 70% total fatty acids to 55% total fatty acids and cis-MUFA increased from 20% total fatty acids to 32% total fatty acids. It was assumed that the total fatty acid content of these foods remained the same as in unmodified products.
Table 3. Baseline values for SFA, cis-MUFA intakes, the percentage supplied by dairy products and total serum cholesterol in eleven EU member states (adapted from Hulshof et al. (Reference Hulshof, van Erp-Baart and Anttolainen18) and Allender et al. (Reference Allender, Scarborough, Peto, Raynor, Leal, Luengo-Fernández and Gray35))
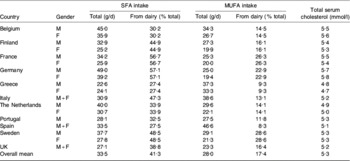
M, males; F, females.
Baseline values for the mean total serum cholesterol concentrations in the population of the same eleven member states were taken from a WHO study carried out in 2002(Reference Allender, Scarborough, Peto, Raynor, Leal, Luengo-Fernández and Gray35) (Table 3) whilst the latest records on annual deaths from CHD, stroke and other CVD-related causes in the selected member states and for the total EU27 (Austria, Belgium, Bulgaria, Cyprus, Czech Republic, Denmark, Estonia, Finland, France, Germany, Greece, Hungary, Republic of Ireland, Italy, Latvia, Lithuania, Luxembourg, Malta, The Netherlands, Poland, Portugal, Romania, Slovak Republic, Slovenia, Spain, Sweden, UK) were obtained from Allender et al. (Reference Allender, Scarborough, Peto, Raynor, Leal, Luengo-Fernández and Gray35).
Approaches used
Model 1
The reduction in EI from SFA (as % total EI) that would result from the change in milk fat composition was calculated and this value was used in the model of Keys et al. (Reference Keys, Anderson and Grande36) to predict the absolute reduction in serum total cholesterol (mmol/l) that would result:

where ΔS is change in % EI from SFA, ΔP is change in % EI from PUFA and ΔC is change in dietary cholesterol intake. It was assumed that ΔP and ΔC were zero.
Using the calculated reduction in total cholesterol, the risk of an incidence of CHD was calculated from a risk analysis(Reference Law, Thompson and Wald37), which predicts the maximum risk reduction to be 30% per 0·6 mmol/l reduction in serum total cholesterol.
Model 2
The reduction in EI from SFA (as % total EI) was calculated from the model of Keys et al. (Reference Keys, Anderson and Grande36) and together with the serum cholesterol values reported by Allender et al. (Reference Allender, Scarborough, Peto, Raynor, Leal, Luengo-Fernández and Gray35), the percentage reduction in serum cholesterol was predicted. To calculate the likely percentage reduction in CHD events the percentage reduction serum cholesterol was then used in the model of Lipids Research Clinics Program(38), which proposes that a 4% reduction in cholesterol will result in a 9% reduction in risk of developing CHD.
Model 3
The percentage reduction in serum cholesterol was calculated as for model 2 and this value was used in the model of Sacks & Katan(Reference Sacks and Katan39) to predict the percentage reduction in risk of a cardiac event. The latter model proposes that the risk of a coronary event is reduced by 2% for each 1% reduction in total cholesterol.
Model 4
The absolute reduction in serum total cholesterol (mmol/l) was estimated from the model of Keys et al. (Reference Keys, Anderson and Grande36) followed by an estimate of the percentage reduction in death from CHD derived from the data of Lloyd-Williams et al. (Reference Lloyd-Williams, O'Flaherty, Mwatsama, Birt, Ireland and Capewell40). The data derived indicate that for men and women a reduction in serum cholesterol of 0·063 mmol/l would result in a 1·4 and 0·89% reduction in CHD deaths respectively. These estimates were used with the EU death rate data(Reference Allender, Scarborough, Peto, Raynor, Leal, Luengo-Fernández and Gray35) to predict the absolute yearly reduction in deaths from CHD. The absolute yearly reduction in deaths from stroke and other CVD-related causes was estimated using the same reduction in risk as for CHD and the estimates for the whole EU 27 was based on the mean reduction in risk of the eleven selected member states.
Model 5
The model of Mensink et al. (Reference Mensink, Zock, Kester and Katan7), which predicts changes in cholesterol pools from isoenergetic replacement of carbohydrate with SFA, MUFA and PUFA was rearranged to predict the effect on serum total cholesterol:HDL-cholesterol of isoenergetic replacement of SFA with cis-MUFA. Minor balancing with PUFA was included to maintain equal lipid exchanges:

where ΔS is reduction in % EI from SFA, ΔM is increase % EI from cis-MUFA and ΔP is balancing change in % EI from PUFA.
It has been proposed that changes in serum total cholesterol:HDL-cholesterol have greater prediction power for CHD events than changes in either total cholesterol or LDL-cholesterol(Reference Mensink, Zock, Kester and Katan7). The estimated reduction in this ratio was then used to predict the percentage reduction in CHD events on the basis that a one unit change in total cholesterol:HDL-cholesterol is associated with a 53% change in the risk of mycocardial infarction(Reference Stampfer, Sacks, Salvini, Willet and Hennekens41).
Results and discussion
Despite being derived from two different studies there was a positive relationship between baseline data for total SFA intake and total cholesterol concentrations (R 2 0·51) and a positive relationship of similar strength between intake of SFA from dairy products and total cholesterol (R 2 0·53; Fig. 2), suggesting that the total cholesterol values did to some extent reflect the previously recorded habitual intake of SFA at the population level. The predicted reductions in total cholesterol arising from the change in milk fatty acid composition ranged from 0·038 mmol/l (0·8%) in Greek men to 0·141 mmol/l in German women (2·43%) with a mean reduction of 0·078 mmol/l (1·46%) across the eleven member states. These changes were the result of essentially replacing 1·3% EI from SFA with cis-MUFA. In accordance with this premise, the predicted mean reduction in total cholesterol is slightly larger than the value of 0·063 mmol/l that was estimated during a similar exercise but based on replacing 1% dietary SFA by 0·5% each of cis-MUFA and PUFA(Reference Lloyd-Williams, O'Flaherty, Mwatsama, Birt, Ireland and Capewell40). However, the predicted percentage reductions in total cholesterol concentrations are smaller and greater than seen in the two previously described intervention studies(Reference Noakes, Nestel and Clifton23, Reference Seidel, Deufel and Jahreis24) respectively, although it is of note that the values in the control groups of these two studies are considerably higher and somewhat lower respectively than the baseline values used in the present study. The reductions in the total cholesterol:HDL-cholesterol predicted from model 5 ranged from 0·072 units (German women) to 0·016 units (Greek men) with an overall mean of 0·037 units. These reductions are all smaller than those calculated from the results of the two intervention studies(Reference Noakes, Nestel and Clifton23, Reference Seidel, Deufel and Jahreis24). Taken together, these data do point to the need for more intervention studies to explore a wide range of phenotypes and background diets over longer periods.
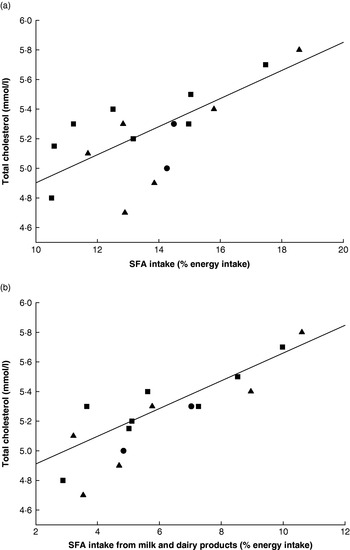
Fig. 2. Relationships between (a) total SFA and (b) SFA from milk and dairy products and total serum cholesterol in eleven EU member states. (■), Males; (▲), females; (•), males and females. (a) R 2 0·51; (b) R 2 0·53. (Adapted from Hulshof et al. (Reference Hulshof, van Erp-Baart and Anttolainen18) and Allender et al. (Reference Allender, Scarborough, Peto, Raynor, Leal, Luengo-Fernández and Gray35).)
Predicted reductions in risk of a CHD event provided by models 1, 2, 3 and 5 are given in Table 4. There was considerable variability in risk reduction both between member states and between models, although all models produced a similar ranking. Germany followed by France had the greatest predicted reduction in risk whilst Greece consistently had the lowest, all of which was consistent with the baseline SFA intakes. Model 5 was the only one to use changes in both SFA and cis-MUFA and to base its assessment of change in risk on changes in total cholesterol:HDL-cholesterol rather than total cholesterol. It has been argued that since some of the SFA in milk and dairy products (notably 12:0 and 14:0) increase the cardioprotective HDL-cholesterol as well as LDL-cholesterol, predictions that account for both changes have greater power(Reference Mensink, Zock, Kester and Katan7). Thus, it seems that the smaller risk reductions provided by model 5 may be more realistic than those from the other models, although it has been commented that epidemiological observations often suggest a much greater effect of dietary fat composition change than the cholesterol-based model(Reference Mensink, Zock, Kester and Katan7).
Table 4. Predicted reductions in risk of a CHD event in eleven EU member states estimated by four modelsFootnote *
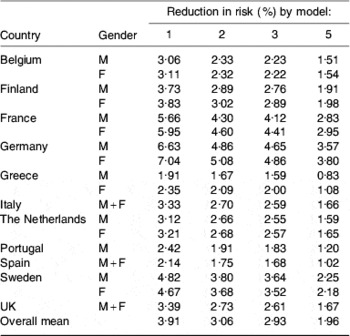
M, males; F, females.
* For details of models, see text.
Table 5 gives the predicted reduction in deaths estimated by model 4. This model suggests a reduction of about 7900 and 2700 deaths from CHD and stroke per year respectively in the eleven selected member states, which compares favourably with predicted respective reductions of 7000 and 2000 for fifteen member states(Reference Lloyd-Williams, O'Flaherty, Mwatsama, Birt, Ireland and Capewell40); the authors of the latter study have proposed a slightly more conservative dietary change(Reference Lloyd-Williams, O'Flaherty, Mwatsama, Birt, Ireland and Capewell40). Table 5 predicts reductions of approximately 10 500 and 3900 deaths from CHD and stroke per year respectively in the EU27. Of note is the fact that the predicted reduction in deaths from CHD in the UK is broadly in line with the reduction that has been predicted for England and Wales if value added tax was to be extended to dietary SFA(Reference Marshall42), although interestingly in a commentary on the value added tax idea it was suggested that substituting a modified food for a traditional food may represent a more-consumer-friendly intervention(Reference Kennedy and Offutt43).
Table 5. Reduction in deaths from CHD and stroke in eleven EU member states and the EU27 estimated by model 4Footnote *
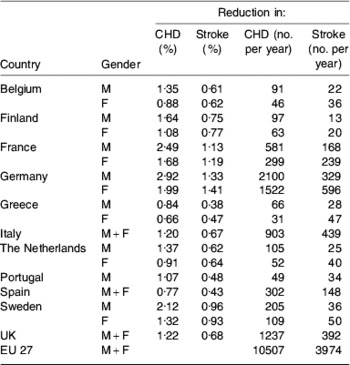
EU27, Austria, Belgium, Bulgaria, Cyprus, Czech Republic, Denmark, Estonia, Finland, France, Germany, Greece, Hungary, Republic of Ireland, Italy, Latvia, Lithuania, Luxembourg, Malta, The Netherlands, Poland, Portugal, Romania, Slovak Republic, Slovenia, Spain, Sweden, UK; M, males; F, females.
* For details, see text.
Ultimately, the direction of any population-directed diet modification will depend on cost–benefit analysis and political will. However, since CVD is currently estimated to cost the EU in the order of €169×109/year(Reference Leal, Luengo-Fernández, Gray, Petersen and Rayner44) and €192×109/year(Reference Allender, Scarborough, Peto, Raynor, Leal, Luengo-Fernández and Gray35) and likely to rise because of an ageing population, the economic scope for some dietary change would appear to be large.
Conclusions
Despite the recognised benefits of reducing SFA intake, the UK target that intake should not on average exceed 11% total food energy(16) is met by few countries within the EU. Whilst milk and dairy products are the single largest source of SFA in most diets, epidemiological evidence suggests cardioprotective and other benefits of milk such that reducing consumption to meet SFA targets may be counterproductive. There is evidence to suggest that modifying the fatty acid content of milk fat by reducing SFA and increasing cis-MUFA will reduce risk factors for CHD, and such changes can now be achieved through alteration of the diet of the dairy cow. Modelling of the effects of such changes at an EU population level points to the potential for substantial reductions in coronary events and deaths, although implementation of such a widespread policy will require major changes in the agro-food industry at both economic and political levels. Given the current and projected costs of health care such a process requires urgent consideration.
Acknowledgements
This work was supported by LIPGENE, an EU Sixth Framework Programme Integrated Project (2004–2009) (http://www.ucd.ie/lipgene/). I also wish thank my colleague Dr Anne Marie Minihane for much valuable discussion and criticism during the preparation of this paper. The author declares no conflict of interest.