INTRODUCTION
Threadworms, Strongyloides spp. within the Rhabditoidea superfamily are small (3–8 mm), slender nematodes of the small intestine (and caeca in some species). Only the female worms are parasitic and adult worms produce eggs by the process of parthenogenesis. Larvated eggs or first stage larvae (L1), in the case of Strongyloides stercoralis, are excreted in the hosts’ feces and undergo either rapid development through L1 and second stage larvae (L2) to infective third stage larvae (L3, homogonic cycle), or develop through the larval stages into free-living adult worms that may reproduce in the external environment to generate infective L3 (heterogonic cycle). Under certain conditions, some Strongyloides species may have further generations externally, but at present it is unknown whether this takes place under natural conditions (Streit, Reference Streit2008). The life cycle for Strongyloides ransomi of pigs, as representative of the species of veterinary importance, is summarized in Fig. 1. Percutaneous or oral (by penetration of oral mucosa) infection by L3, after the free-living phase, is followed by migration; for example via the lymphatic or haemato-tracheal routes (Mansfield et al. Reference Mansfield, Alavi, Wortman and Schad1995), after which adult female worms develop in the proximal small intestine. The pre-patent period varies with nematode species and route of transmission. Lactogenic, mainly colostral, transmission from the dam to offspring following reactivation of inhibited somatic larvae is common in almost all species (Moncol, Reference Moncol1975; Shoop et al. Reference Shoop, Michael, Eary and Haines2002).
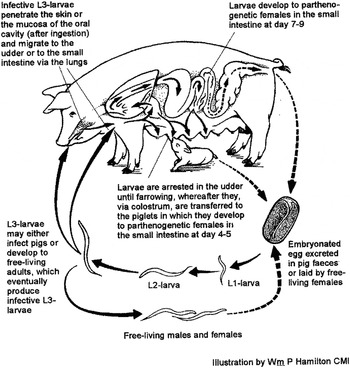
Fig. 1. The life cycle of Strongyloides ransomi in pigs. (Source: Food and Agriculture Organization of the United Nations, Roepstorff and Nansen (Reference Roepstorff and Nansen1998), Epidemiology, diagnosis and control of helminth parasites of swine. Reproduced with permission.)
Strongyloides spp. infect a range of domestic animal species worldwide. In general, there is host specificity for each Strongyloides spp. However, this has been based primarily on morphology and not genetic confirmation. In studies on the prevalence and impact of infection, it is generally assumed that the species present is the one that has been previously identified as belonging to that host. Despite being capable of infecting different age groups, clinical disease is most often encountered in the young animals. In herbivores, the small, oval, thin-shelled, larvated (atypical) eggs are easily recognized when fecal material is subject to flotation, a diagnostic procedure commonly performed for gastrointestinal nematodes. These infections are only sporadically associated with clinical signs in herbivores and in recent years clinical reports have been relatively few. However, the recent findings of Strongyloides stercoralis in dogs in some Nordic countries (Finland and Norway) (Dillard et al. Reference Dillard, Saari and Anttila2007; Hoegaasen et al. Reference Hoegaasen, Hamnes, Davidson and Lund2012) are interesting and may perhaps represent spreading of this potential zoonotic infection. This paper reviews the occurrence and impact of Strongyloides spp. in companion animals and large livestock (Table 1), although we are also aware of the importance of these infections in other species, e.g. rabbits and birds. The potential zoonotic implications of these parasites and future research requirements are discussed.
Table 1. FACTS BOX: different Strongyloides species in domestic animals

CANINE AND FELINE STRONGYLOIDOSIS
Occurrence
Strongyloides stercoralis occurs worldwide in dogs and infects people, non-human primates and wild canids (Fig. 2). Cats have been infected with S. stercoralis experimentally, but natural infections, until recently, have only been reported with Strongyloides tumefaciens (North America; India), Strongyloides planiceps (Japan; also infecting wild canids and weasels) and Strongyloides felis (India; Australia). Strongyloides also occurs in cats in Europe and Africa, but the species has not been specified and could potentially be S. stercoralis (Abu-Madi et al. Reference Abu-Madi, Al-Ahbabi, Al-Mashhadani, Al-Ibrahim, Pal and Lewis2007). The identification of the species in dogs and cats has been based on morphology and, in studies on prevalence and life cycles, morphology or assumptions regarding the species present in these hosts, are used.
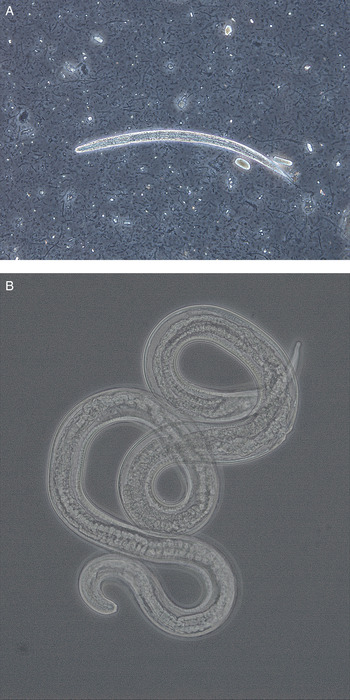
Fig. 2. (A) Larva (approximately 290 µm) and (B) adult female (1·5 mm) of Strongyloides stercoralis from dogs in Norway. The adult worm was recovered from a fecal smear of a puppy with severe diarrhoea. Photos kindly provided by Inger Sofie Hamnes, Norwegian Veterinary Institute.
Strongyloides stercoralis prevalence ranges from 0 to over 50% in dogs, with most studies indicating 0·2 to <5% with younger dogs more likely to be infected (Junior et al. Reference Junior, Goncalves-Pires, Silva, Goncalves and Costa-Cruz2006; Palmer et al. Reference Palmer, Thompson, Traub, Reese and Robertson2008; Gates and Nolan, Reference Gates and Nolan2009; Paulos et al. Reference Paulos, Addis, Fromsa and Mekibib2012; Riggio et al. Reference Riggio, Mannella, Ariti and Perrucci2013). Based on the few longitudinal studies available, S. stercoralis does not appear to be decreasing in prevalence (Asano et al. Reference Asano, Suzuki, Matsumoto, Sakai and Asano2004; Itoh et al. Reference Itoh, Kanai, Hori, Hoshi and Higuchi2009a , Reference Itoh, Kanai, Hori, Nakao, Hoshi and Higuchi b ).
Prevalence of Strongyloides in cats ranges from 0 to 4% (Mircean et al. Reference Mircean, Titilincu and Vasile2010; Mohd Zain et al. Reference Mohd Zain, Sahimin, Pal and Lewis2013; Takeuchi-Storm et al. Reference Takeuchi-Storm, Mejer, Al-Sabi, Olsen, Thamsborg and Enemark2015). However, in Qatar and Christmas Island, higher prevalence (up to 46%) has been seen (Abu-Madi et al. Reference Abu-Madi, Al-Ahbabi, Al-Mashhadani, Al-Ibrahim, Pal and Lewis2007; Adams et al. Reference Adams, Elliot, Algar and Brazell2008). In cases where dog and cat parasites were studied together, Strongyloides infections were less common in cats compared with dogs.
Prevalence of infection is influenced by the environment, age of the dog or cat and the housing situation. Prevalence, as well as the clinical significance of Strongyloides in neonatal dogs, is likely underestimated since standard fecal flotation methods have low sensitivity for recovery of S. stercoralis, S. tumefaciens and S. felis L1, which are more frequently present than larvated eggs (Bowman et al. Reference Bowman, Hendrix, Lindsay and Barr2002; Zajac and Saleh, Reference Zajac and Saleh2013). Non-reproductive female adults can live for several months also resulting in underestimating prevalence (Mansfield et al. Reference Mansfield, Niamatali, Bhopale, Volk, Smith, Lok, Genta and Schad1996).
Biology and transmission
All of the Strongyloides species infecting dogs and cats have the typical lifecycle with adult female worms residing in the host reproducing via parthenogenesis and male and female adults in the environment reproducing sexually. The adult females live in the mucosal epithelium and lay eggs within the crypts of the small intestine, except for S. tumefaciens, which is located in tumors in the mucosa of the large intestine. While eggs with L1 can be found in fresh feces, hatched L1 are more frequently seen with S. stercoralis, S. tumefaciens and S. felis. The eggs are oval and range from 50 to 58 µm by 30–34 µm, with S. planiceps being slightly larger. The L1 of S. stercoralis has a prominent genital primodium, a rhabditiform oesophagus and straight tail. The host, the environment and the strain determine if the L1 enter the homogonic or heterogonic route, with the homogonic route favoured at higher environmental temperatures. With the heterogonic route, there typically is only one generation of free-living adults, although more might occur with S. planiceps.
The primary mode of infection for S. stercoralis is percutaneous, with oral exposure resulting in oral mucosal penetration (Galliard, Reference Galliard1950, Reference Galliard1951a , Reference Galliard b ). Arrested larvae do not appear to serve as a primary source of transmammary infection or reinfection (Mansfield and Schad, Reference Mansfield and Schad1995; Mansfield et al. Reference Mansfield, Niamatali, Bhopale, Volk, Smith, Lok, Genta and Schad1996). However, transmammary infection can occur if the bitch is infected late in gestation or during lactation (Shoop et al. Reference Shoop, Michael, Eary and Haines2002). The migration route of the larvae during initial infection appears to be related to the strain or an adaptation of the parasite to the host (Genta, Reference Genta1989). While pulmonary migration has been demonstrated with some strains, its role might be limited in dog specific strains (Faust and Kagy, Reference Faust and Kagy1933; Schad et al. Reference Schad, Aikens and Smith1989; Mansfield et al. Reference Mansfield, Alavi, Wortman and Schad1995).
The larvae reach the small intestine within 4–5 days with the prepatent period ranging from 5 to 21 days. Maximum egg output occurs approximately 20 days post infection and can continue at a lower level for up to 11 weeks. It is hypothesized that the immune system of the dog inhibits egg production prior to the death of the female worms. This has been supported by studies in which exposure to corticosteroids results in recrudescence of larval shedding (Genta et al. Reference Genta, Schad and Hellman1986; Mansfield et al. Reference Mansfield, Niamatali, Bhopale, Volk, Smith, Lok, Genta and Schad1996).
Autoinfection (the ability of L1 to molt to L2, then L3 and become an adult without leaving the host) does not appear to occur with the dog strain under normal conditions. If dogs are infected with a human strain, autoinfection can occur, but within a few generations, reverts to normal infections (requiring the L1 to leave the dog to continue development) (Faust and Kagy, Reference Faust and Kagy1933; Genta, Reference Genta1989). Autoinfection leading to hyperinfection (migrating L3 throughout the body) also can occur in neonatal dogs and when dogs are exposed to steroids. Dissemination of adult worms to the trachea, lungs and other extra-intestinal sites is rare, but has been observed in animals treated with high doses of prednisolone (Genta et al. Reference Genta, Schad and Hellman1986).
Less is known about the life cycles of S. tumefaciens, S. planiceps and S. felis. The mode of infection for these species is believed to be percutaneous and per os. Strongyloides tumefaciens females reside in nodules in the large intestine and L1 are found in feces. Other aspects of the S. tumefaciens life cycle are unknown. Strongyloides planiceps infects the host percutaneously and per os, has a prepatent period of 10–11 days, resides in the small intestine and produces partially embryonated eggs in the feces (Fukase et al. Reference Fukase, Chinone and Itagaki1985). Strongyloides felis infects largely older cats and enters the host percutaneously, followed by pulmonary migration. The prepatent period ranges from 9 to 14 days and results in L1 in the feces (Speare and Tinsley, Reference Speare and Tinsley1986, Reference Speare and Tinsley1987).
Clinical signs and diagnosis
Most Strongyloides infections in dogs and cats are asymptomatic and self-limiting. In cases of high worm burdens in dogs, diarrhoea (watery and with or without mucous) and bronchopneumonia can occur (Nolan, Reference Nolan and Bowman2001). More severe clinical signs including extensive skin lesions and bronchopneumonia, sometimes leading to death, have occurred when dogs were infected with human and non-human primate strains (Faust and Kagy, Reference Faust and Kagy1933). In neonatal dogs, S. stercoralis is a differential diagnosis for pulmonary disease and diarrhoea. Clinical signs of infection with S. planiceps have not been noted and are rare with S. felis, while S. tumefaciens infections result in tumour-like nodules in the large intestine and loose or diarrheic feces.
Specific methods for recovering L1, such as the Baermann method or Zinc sulphate flotation solution, are recommended for S. stercoralis and S. felis; however, fecal flotation is effective for S. planiceps (Nolan, Reference Nolan and Bowman2001; Zajac and Saleh, Reference Zajac and Saleh2013). Differentiation of Strongyloides L1 from those of Ancylostoma spp. is sometimes required. Also, larvated S. felis and Ancylostoma spp. eggs are similar, requiring culture to differentiate. At necropsy, the various species can be distinguished by location, size, appearance of the ovaries (straight or spiralled) and the shape of the tail. However, due to size, special attention is needed if sieving is applied.
Treatment and prevention
No products are registered for the treatment of Strongyloides infections in dogs and cats. Treatment doses can be higher than for other gastro-intestinal nematodes, due to the location of the female worms in the mucosa and crypts. In normal infections, ivermectin (200 µg kg−1 BW), albendazole (100 mg kg−1BW 2x days−1 for 3 days) and fenbendazole (50 mg kg−1 BW 1x day−1 for 3–5 days) are expected to be efficacious in the treatment of S. stercoralis dogs (Nolan, Reference Nolan and Bowman2001; Itoh et al. Reference Itoh, Kanai, Hori, Nakao, Hoshi and Higuchi2009b ). Treatments are less efficacious against migrating S. stercoralis L3, but unless the infected dog is very young or has been treated with steroids, the number of migrating L3 is low. In cases of hyperinfection, fenbendazole (50 mg kg−1 BW 1x day−1 for 7–14 days) and ivermectin (200 µg kg−1 BW 1x day−1 every 4 days for 3 or 4 doses) have been demonstrated to be effective (Nolan, Reference Nolan and Bowman2001). In cats, ivermectin (200 µg kg−1 BW) has been proposed as a treatment for all species (Bowman et al. Reference Bowman, Hendrix, Lindsay and Barr2002).
Due to the wide environmental dissemination of S. stercoralis, prevention is challenging. Proper hygiene and sanitation in the human population and common methods such as removing dog and cat faeces from the environment and preventing free-roaming dogs could decrease infections. In kennels and breeding facilities, good hygiene and management can prevent heavy infections (Dillard et al. Reference Dillard, Saari and Anttila2007).
Zoonotic aspects
A primary concern with S. stercoralis infections in dogs is the zoonotic potential. Dogs have been infected with S. stercoralis obtained from people in experimental studies and human infections have been related to dog exposure, although genetic typing has not occurred in these cases. More recently, molecular techniques have shown that there are clear differences between the strains and the existence of subspecies has been proposed (Ramachandran et al. Reference Ramachandran, Gam and Neva1997; Schär et al. Reference Schär, Guo, Streit, Khieu, Muth, Marti and Odermatt2014). The zoonotic potential of dog S. stercoralis is now being challenged (Takano et al. Reference Takano, Minakami, Kodama, Matsuo and Satozono2009; Schär et al. Reference Schär, Guo, Streit, Khieu, Muth, Marti and Odermatt2014). However, as the studies by Faust and Kagy (Reference Faust and Kagy1933) demonstrated, S. stercoralis can adapt from a human to dog host, changing its virulence (autoinfection and hyperinfection capabilities) and migratory pathways. If the converse is true, with dog strains adapting to humans and increasing in virulence, then while zoonosis might be less common than previously proposed, dog infections could still pose a significant health risk to people. The zoonotic potential of cat Strongyloides has not been studied, but with its spread into Nordic countries and potential reports of S. stercoralis infections, requires research.
EQUINE STRONGYLOIDOSIS
Occurrence and impact
Strongyloides westeri is relatively common worldwide. Clinical disease is invariably only observed in young foals (i.e. animals of 2–7 weeks-old; Hiepe et al. Reference Hiepe, Nickel and Siebeke1975) and only when high levels of infection are present (Netherwood et al. Reference Netherwood, Wood, Townsend, Mumford and Chanter1996). Prevalence has been measured as high at stud farm level, but at low levels in individual horses; for example, the parasite was detected in <10% of all horses sampled in a number of studies (Mfitilodze and Hutchinson, Reference Mfitilodze and Hutchinson1989; Lyons et al. Reference Lyons, Tolliver, Drudge, Granstrom and Collins1993; Gawor, Reference Gawor1995; Teixeira et al. Reference Teixeira, Felippelli, Cruz, Maciel, Fávero, Gomes, Buzzulini, Prando, Bichuette, Lopes, Oliveira and Costa2014). In populations where there is a history of high use of prophylactic anthelmintic treatments such as ivermectin, prevalence at stud farm level is lower (Relf et al. Reference Relf, Morgan, Hodgkinson and Matthews2013). In one study, performed where the prevalence of S. westeri within the same population was compared over time, prevalence was found to be greater (i.e. 30% of foals) in 2014 than in previous reports from the same area sampled during the late 1990s and early 2000s (Lyons and Tolliver, Reference Lyons and Tolliver2014). The authors of this study proposed that the relative increase may have been due to a reduced usage of ivermectin in foals over time due to concerns about resistance to this compound in the other important foal parasitic nematode, Parascaris equorum (Lyons and Tolliver, Reference Lyons and Tolliver2014). This theory remains to be substantiated by further evidence.
Biology and transmission
Like other species of Strongyloides, S. westeri can undergo parasitic cycles and free-living reproductive cycles in the environment, with females only in the host cycle and free-living males and females sexually reproducing outside the host. Survival of S. westeri worms in the environment is favoured by warm and humid conditions, which can lead to high levels of infection and clinical signs. Thus, disease is more likely to be seen in foals reared where bedding accumulates and management is poor. An important source of infection to foals is transmission of larvae within days after birth from the mare's milk. Mares are thought to harbour inhibited larvae from early life infections. These larvae reactivate around the time of parturition and travel to the mammary gland to be transmitted in the milk. There is little known about how long S. westeri larvae persist in the milk. In one study, in a single pony mare, larvae were found to be present in the milk on days 10, 24 and 32 days post-partum (Mirck, Reference Mirck1977). However, in other mares under study, larvae were not detected in milk despite the fact that their foals had patent infections between 13 and 16 days post-partum. The experimental conditions in this study precluded environmental transmission, so the infections were likely to be lactogenic and the lack of larvae detected in the mares’ milk was probably due to the relatively low sensitivity of the test applied (Mirck, Reference Mirck1977). In the same study, 10 foals were reared artificially; these did not develop S. westeri infections, indicating that vertical transmission via the placenta does not occur. In foals, the prepatent period has been measured as 10–14 days, with the length of patency ranging from 43 to 83 days (Mirck and Franken, Reference Mirck and Franken1978). By the time foals are 4–6 months old, egg shedding ceases (Hiepe et al. Reference Hiepe, Nickel and Siebeke1975).
Clinical signs and diagnosis
As indicated above, S. westeri only causes clinical signs in young foals when present in high numbers. Signs in foals up to a few weeks-old include acute diarrhoea, weakness and weight loss as well as respiratory signs. One study demonstrated that the association between S. westeri and clinical signs of diarrhoea was dependent on the numbers of eggs shed, with a positive association only observed when more than 2000 eggs per gram (EPG) of feces were detected (Netherwood et al. Reference Netherwood, Wood, Townsend, Mumford and Chanter1996). Infection with large numbers of larvae leads to inflammation, oedema and erosion of the small intestinal epithelium (Brown et al. Reference Brown, MacKay, Chandra, Davenport and Lyons1997). This can affect absorption and digestion and result in diarrhoea. It is important to appreciate that S. westeri is one of several pathogens that are associated with diarrhoea in foals. Other pathogens include bacteria such as Salmonella and Clostridium spp., and viruses such as rotavirus, and, indeed, these are more likely causes of diarrhoea in young foals than S. westeri infections (Netherwood et al. Reference Netherwood, Wood, Townsend, Mumford and Chanter1996). When foals are infected via the environment, the larvae penetrate the skin or are ingested, and the larvae then undergo a migratory life cycle, via the circulatory system and lungs. As larvae migrate through the lungs, they cause inflammation and haemorrhage, which can lead to respiratory signs in heavy infections. Clinical signs are much less common in older foals. Older foals are more likely to display clinical signs of infection with the intestinal ascarid nematode, P. equorum.
Diagnosis is made on the basis of the detection of large numbers of the characteristic larvated eggs or hatched larvae in faeces; however, it should be noted that high numbers of eggs and larvae can be found in the faeces of foals exhibiting no clinical signs of strongyloidosis.
Treatment and prevention
Control of this parasite on stud farms where there has been a clinical problem requires both chemical and environmental interventions. Treatment of infected foals with anthelmintics will lower environmental contamination, but it is also important to remove fecal-contaminated bedding and to provide dry stabling to limit amplification of free-living generations. The parasites are susceptible to macrocyclic lactones, modern benzimidazoles and pyrantel (Ludwig et al. Reference Ludwig, Craig, Bowen, Ansari and Ley1983; Felippelli et al. Reference Felippelli, Cruz, Gomes, Lopes, Teixeira, Maciel, Buzzulini, Bichuette, Campos, Soares, Bergamasco, Oliveira and Costa2015). As mares can act as a reservoir of infection, periparturient anthelmintic treatments can be administered in an attempt to reduce transmammary transmission. Transmission of larvae to foals via milk may be prevented by the treatment of mares with ivermectin within 24 h of foaling (Di Pietro, Reference Di Pietro1989). Anthelmintic resistance has not been reported in S. westeri thus far, but it is important to balance chemotherapeutic administration of anthelmintics to control these worms against the risk of increasing selection pressure in other equine parasitic nematode species for which drug resistance is an issue.
RUMINANT STRONGYLOIDOSIS
Occurrence
Strongyloides papillosus, a universally common intestinal nematode of cattle and small ruminants, generally parasitizes the small intestine as female adult stages and can cause diarrhoea and malnutrition, especially in young animals. The prevalence of S. papillosus has been reported to vary between age groups, management systems, areas and climates; for example, the parasite was detected in 58% of lambs in northern Tunisia during spring (Akkari et al. Reference Akkari, Gharbi and Darghouth2012), in 18% of sheep on pasture in southern Poland during summer (Balicka-Ramisz et al. Reference Balicka-Ramisz, Ramisz and Zychlinska-Buczek2013) and in only 3% of sheep in winter season in northern Egypt, while nil were infected in the other seasons in the same area (Khalafalla et al. Reference Khalafalla, Elseify and Elbahy2011). In calves, S. papillosus was detected in 10–53% of 1-month old of beef calves during housing in central Germany, however, during the grazing season the prevalence decreased to 6% (Jäger et al. Reference Jäger, Gauly, Bauer, Failing, Erhardt and Zahner2005). In Costa Rica, 4–20% of 4 month-old dairy calves were found infected by coproscopy (Jiménez et al. Reference Jiménez, Fernandez, Alfaro, Dolz, Vargas, Epe and Schnieder2010). High levels of infections in calves have been seen in the wet/humid summer season in southern Japan (Taira and Ura, Reference Taira and Ura1991).
Clinical impact
Infections in small ruminants are commonly associated with maceration of pedal skin in young animals, and more than 50 000 EPG of feces have been observed in perinatal lambs grazing wet (waterlogged) areas without clinical signs. The penetration of the interdigital and/or coronary area may predispose to foot-rot (Deplazes et al. Reference Deplazes, Eckert, von Samson-Himmelstjerna and Zahner2013). An experimental infection with S. papillosus in goats showed clinical signs, including transient diarrhoea, elongated fecal pellets terminally, dehydration, anorexia and anaemia (Pienaar et al. Reference Pienaar, Basson, du Plessis, Collins, Naude, Boyazoglu, Boomker, Reyers and Pienaar1999). The pre-patent period is similar to that in cattle infections: 8–14 days. Heavy infection causes serious strongyloidosis (Vegors, Reference Vegors1954; Davis et al. Reference Davis, Herlich and Bowman1960) and, in the 1980s, more than 150 calves died suddenly in southern Japan in association with S. papillosus infection (Taira and Ura, Reference Taira and Ura1991). These calves died within a few minutes of collapsing, without any significant preceding clinical signs. The animals had a large number of S. papillosus eggs and numerous adult worms in the intestine at post mortem. The deaths were attributed to hyperinfection by L3 due to rearing of similar-aged calves on sawdust bedding at high stocking density (Taira and Ura, Reference Taira and Ura1991). Sudden death of calves was subsequently confirmed by experimental infection with a large amount of S. papillosus L3 (Taira et al. Reference Taira, Nakamura, Tsuji, Kubo and Ura1992). Heavily infected calves showed accelerated respiration as the first clinical sign shortly before death, but body temperature remained almost normal and no consistent hematological, biochemical or histological changes were identified (Ura et al. Reference Ura, Nakamura, Tsuji and Taira1992; Nakanishi et al. Reference Nakanishi, Nakamura, Ura, Tsuji, Taira, Tanimura and Kubo1993). Vocal noises and general spasms were observed several times during the course of death. All animals died within 3–4 minutes from the first respiratory signs (Ura et al. Reference Ura, Nakamura, Tsuji and Taira1992). When electrocardiographic and pneumographic examinations were employed on infected calves, various patterns of tachy- and brady-arrhythmia were observed 1–2 days before death while abnormal pneumograms were not observed (Tsuji et al. Reference Tsuji, Itabisashi, Nakamura, Taira, Kubo, Ura and Genno1992; Ura et al. Reference Ura, Taira, Nakamura, Tsuji and Hirose1993). Although Taira et al. intensively investigated sudden death in cattle due to S. papillosus, the mechanisms underlying cardiac sudden death have yet to be definitively confirmed. The only way to predict an occurrence of sudden death in calves is by performing fecal egg counts. Calves with high counts (EPG > 10 000) should be treated with effective anthelmintics to avoid disease and build-up of infections in the group.
Pathogenesis
Rabbits are also susceptible to S. papillosus, but experimental rodents are not (Buzubik Reference Buzubik1965; Neilson and Ngheim Reference Neilson and Ngheim1974; Nwaorgu and Connan, Reference Nwaorgu and Connan1980; Taira et al. Reference Taira, Minami and Smitanon1991). Heavily infected rabbits often die due to significant wasting, characterized by anaemia, severe anorexia and weight loss, but no cardiac abnormalities have been seen (Nakamura et al. Reference Nakamura, Tsuji and Taira1994). In addition, gastrointestinal motor disturbances were identified as underlying the manifestations and death in infected animals (Kobayashi and Horii, Reference Kobayashi and Horii2008). However, the mechanisms for death resulting from S. papillosus infection have yet to be identified in either ruminants or rabbits. Despite the importance of these symptoms, little is known about the mechanisms causing the gastrointestinal manifestations of this infection. Enteric parasitic infections are known to evoke changes in intestinal motility (Gregory et al. Reference Gregory, Wenham, Poppi, Coop, MacRae and Miller1985; Crosthwaite et al. Reference Crosthwaite, Huizinga and Fox1990; Marzio et al. Reference Marzio, Blennerhassett, Chiverton, Vermillion, Langer and Collins1990; Palmer et al. Reference Palmer, Wong-Riley and Sharkey1998). Although various reports have described the effects of parasitic infection on intestinal transit, the mechanisms inducing gastrointestinal motor disturbance are poorly understood. In a study by Kobayashi et al. (Reference Kobayashi, Kajisa, Farid, Yamanaka and Horii2009), adult S. papillosus were surgically implanted into the duodenum and established in Mongolian gerbils. These worms persisted in the small intestine for at least 4 days after implantation. Following decreased fecal output, however, increased death rate and decreased survival time were observed in the gerbils. The increase in death rate and decrease in survival time correlated with increases in the number of implanted adult worms. Animals were then intraperitoneally inoculated with extracts from homogenized adult worms of S. papillosus, and the effects on gastrointestinal motility assessed by contrast radiography after oral administration of barium sulphate. Paralytic ileus was observed, and these symptoms intensified with increasing inoculation of adult worm extract, but were completely lost with heat treatment of the extract. Such results suggest that paralytic ileus underlies the subsequent death observed in Mongolian gerbils after implantation of adult S. papillosus. However, the effector molecules causing gastrointestinal motor disturbance in Strongyloides infections remain unknown. Besides the sudden cardiac death in calves and lambs, S. papillosus infection in sheep may cause oxidative/nitrosative stress, depending on infection intensity, and the condition is relieved by treatment with albendazole (Dimitrijevic et al. Reference Dimitrijevic, Borozan, Katic-Radivojevic and Stojanovic2012).
Treatment and prevention
Strongyloides papillosus-related early damage can be treated and/or completely prevented by use of effective anthelmintics. Macrocyclic lactones such as ivermectin, doramectin and moxidectin are the most popular drugs to treat S. papillosus (Tassi et al. Reference Tassi, Barth and Gross1990; Marley et al. Reference Marley, Illyes, Keller, Meinert, Logan, Hendrickx and Conder1999; Ranjan and Delay, Reference Ranjan and Delay2004). Albendazole is also effective (Dimitrijevic et al. Reference Dimitrijevic, Borozan, Katic-Radivojevic and Stojanovic2012). Again, it is important to emphasize the risk of selecting for anthelmintic resistance in other worm species when unnecessary treatments are applied; treatments should be applied on an evidence basis. As general preventive measures, the first is to avoid mass rearing of naïve calves in a pen or mass deworming before grouping, and the second is keeping bedding dry by using a roof fan, avoiding saw dust, or frequent renewal of litter.
The relationship between Strongyloides spp. in cattle and in small ruminants
To date, most veterinary parasitologists consider that all Strongyloides of farm ruminants to belong to the same species, S. papillosus. Eberhardt et al. (Reference Eberhardt, Mayer, Bonfoh and Streit2008) reported that, in southern Germany, the predominant Strongyloides species found in cattle and in sheep belonged to separate genetically isolated populations. They prepared L3 by culturing collected feces from each farm and single worms were used for polymerase chain reaction (PCR) for sequencing portions of the small subunit (SSU) sequence of 18S rDNA. While mixed haplotype infections were found in cattle, one form clearly dominated. This heterogeneity was not found in the sheep population, indicating that the two forms have different host preferences. By comparing with molecular phylogenetic analysis of the human parasite, S. stercoralis, and the laboratory model species, S. ratti, these authors concluded that Strongyloides from sheep and the predominant Strongyloides from cattle should be considered separate species, suggesting the name S. papillosus for Strongyloides in sheep and the name S. vituli for the predominant Strongyloides in cattle. However, supporting studies by others have yet to be published.
PORCINE STRONGYLOIDOSIS
Occurrence and impact
Strongyloides ransomi (s. S. suis) is found in wild boars (Barutzki et al. Reference Barutzki, Randelzhofer and Gothe1992) and domestic pigs throughout the world, usually at low levels. The worms are found predominantly in young piglets, as adult worms, while sows host somatic larval stages. The incidence of infection is most likely related to management procedures, but a large epidemiological study in the Nordic countries provided no conclusive information on the distribution of this parasite in different production systems due to the sporadic occurrence and low prevalence (as measured by coproscopy) in all age groups (<2%) (Roepstorff et al. Reference Roepstorff, Nilsson, Oksanen, Gjerde, Richter, Ortenberg, Christensson, Martinsson, Bartlett, Nansen, Eriksen, Helle, Nikander and Larsen1998). Similar prevalence levels (3·3%) were observed in fattening pigs on a Polish commercial farm (Knecht et al. Reference Knecht, Popiołek and Zale2011). Some studies indicate that small, traditional herds and low levels of hygiene may predispose to infection (Raynaud et al. Reference Raynaud, Sennelier and Irisarri1975; Roepstorff, Reference Roepstorff1991), but prevalence levels on these farms may in fact not be related to hygiene per se but rather, a high rate vertical transmission as infections occur in young piglets (Raynaud et al. Reference Raynaud, Sennelier and Irisarri1975). In these studies, whole litters were infected with mean fecal egg counts of 5500 EPG (range 300–9500 EPG) at 5–8 weeks of age. Infections occur in both indoor and outdoor reared pigs. Cross-sectional studies in tropical production systems with variable outdoor access have found a prevalence of 3·4% by necropsy (Nganga et al. Reference Nganga, Karanja and Mutune2008) or 1·1% by fecal examination during dry season (Kabululu et al. Reference Kabululu, Ngowi, Kimera, Lekule, Kimbi and Johansen2015). Burdens varied from 1 to 449 worms in the former study, performed during a relatively dry period. Although organic farms in many cases experience more severe helminth infections, a study on nine pig farms with an organic farming history of up to 8 years, found no S. ransomi by repeated fecal examination (Carstensen et al. Reference Carstensen, Vaarst and Roepstorff2002). A range of experimental infection studies, performed in the 1960–1970s in the USA, showed a substantial impact of this parasite on weight gains and feed efficiency in weaners and fatteners, depending on infection levels, and even lethality at high infection levels (up to 30%)(reviewed by Stewart and Hale, Reference Stewart and Hale1988). Infection levels ranged from 5000 to 20 000 L3 kg−1 body weight, and it is difficult to judge how relevant these levels are in the context of field infections at the current time. What these experimental studies do demonstrate though is that, if S. ransomi is present in sufficient numbers, subclinical and clinical disease can occur and, indeed, this has been observed in the field in a relatively recent outbreak on a Danish commercial pig farm (Roepstorff et al. Reference Roepstorff, Mejer, Nejsum and Thamsborg2011). On the flip side, many researchers do not consider S. ransomi as a major pathogen in piglets anymore (e.g. Joachim and Daugschies, Reference Joachim and Daugschies2000) as infections are becoming relatively rare. Intensive indoor rearing and associated high levels of hygiene, and the widespread use of macrocyclic lactones for control of e.g. sarcoptic mange, may be reasons for this low disease incidence.
Biology and transmission
Pigs can be infected orally or percutaneously by free-living infective L3. Oral infection is expected to take place through the oral mucosa. Like other Strongyloides spp., the larval stages undergo migration through the lungs to be become egg-laying females in the small intestine. A prominent feature is the very efficient vertical transfer of infections via colostrum to suckling piglets (Moncol and Batte, Reference Moncol and Batte1966; Stewart et al, Reference Stewart, Stone and Marti1976), while prenatal infection is very unusual (Enigk et al. Reference Enigk, Weingartner, Hazem, Schmelzle and Stoye1974b ). Larvae were usually shed in the colostrum within 24 h post-partum, but were also found for up to 20 days (Stewart et al. Reference Stewart, Stone and Marti1976). Reactivation of hypobiotic somatic stages in sows is most likely related to acquired immunity. Reactivation of larvae deposited in the fat tissues around the mammary gland takes place during pregnancy but the exact mechanism, i.e. the triggering stimulus, is not known. Evidence indicates that a sow may transmit L3 for 4–5 litters without reinfection needing to take place (Stewart et al. Reference Stewart, Stone and Marti1976). Colostral infections are followed by a brief pre-patent period (4 days) as no somatic migration takes place in the infected piglets (Moncol and Batte, Reference Moncol and Batte1966; Enigk et al. Reference Enigk, Weingartner, Hazem, Schmelzle and Stoye1974b ), and nematode egg excretion is observed for a relatively short period as the piglets rapidly acquire protective immunity (Murrell, Reference Murrell1981). If other routes of infection take place, the pre-patent period has been reported as up to 9 days (Deplazes et al. Reference Deplazes, Eckert, von Samson-Himmelstjerna and Zahner2013).
Clinical signs, pathogenesis and diagnosis
Clinical symptoms are mainly observed in the neonatal period up to 2 weeks of age. Clinical signs in acute disease include anorexia, anaemia, diarrhoea, growth retardation or weight loss and, in some cases, sudden death. Severe experimental infections show that profuse diarrhoea can be observed 8–10 days post infection (Dey-Hazra et al. Reference Dey-Hazra, Sallmann, Enigk and Harisch1979). Like many other gastrointestinal nematode infections, infection is accompanied by increased intestinal losses of plasma proteins and reduced amino acid absorption, reflected in hypoalbuminaemia and, occasionally, hypoproteinaemia (Enigk and Dey-Hazra, Reference Enigk and Dey-Hazra1975).
Infection is diagnosed in pigs by the detection of larvated eggs in feces by flotation; for example, by the modified McMaster method. Fecal egg counts are generally higher in young pigs (thousands EPG) compared with older stock, e.g. gilts and sows have counts of 100–300 EPG (Moncol, Reference Moncol1975). Egg size is 20–35 × 40–55 µm and should be differentiated from Metastrongylus (lung worm) eggs, which are slightly larger (45–50 × 55–60 µm) and generally have a thicker shell (Deplazes et al. Reference Deplazes, Eckert, von Samson-Himmelstjerna and Zahner2013). The eggs hatch within 8–12 h at room temperature. Note that false negative results can occur if fecal samples are kept at room temperature too long without refrigeration. False positives also have to be considered if eggs are misidentified, e.g. eggs of free-living nematodes. Studies based on fecal samples picked up from the ground/pen floor need to be considered cautiously.
Treatment and prevention
Intestinal stages are sensitive to modern benzimidazoles, levamisole and macrocyclic lactones (Deplazes et al. Reference Deplazes, Eckert, von Samson-Himmelstjerna and Zahner2013). The somatic larval stages are susceptible to macrocyclic lactones such as ivermectin or repeated administrations of benzimidazoles, while levamisole is less efficacious (Enigk et al. Reference Enigk, Weingartner and Schmelzle1974a ). Treatment of infected sows in late gestation with in-feed ivermectin (100 µg kg−1 BWT for 7 days) has been demonstrated to result in almost complete elimination of L3 in the milk post-partum with no measurable transfer to piglets (Barth et al. Reference Barth, Rehbein, Reid and Barrick1996; Drag et al. Reference Drag, Green, Howser, Wallace, Cox and Barrick1998). Single subcutaneous injection of 300 µg kg−1 ivermectin had a similar effect (Barth and Preston, Reference Barth and Preston1985). The high efficacy and persistence of ivermectin and other macrocyclic lactones, for example, doramectin (Logan et al. Reference Logan, Weatherley and Jones1996) and abamectin (Lopes et al. Reference Lopes, Teixeira, Felippelli, Cruz, Buzulini, Maciel, Fávero, Gomes, Prando, Bichuette, Santos and Costa2014), is probably the main reason for relatively few clinical cases and less importance of this infection in modern pig production systems, as milk-borne infections are effectively prevented by the administration of such compounds to pregnant sows. Alternatively, or additionally, one or more strategic treatments of the piglets can be considered. Other preventive measures include sanitation of the pen environment (i.e. cleaning and drying), thus reducing the numbers of free-living larvae and avoiding maceration of the skin with less risk of percutaneous transmission. Recently introduced welfare-promoting changes in pig management (i.e. the provision of sprinklers and rooting material) may result in a higher risk of infection (Roepstorff et al. Reference Roepstorff, Mejer, Nejsum and Thamsborg2011).
Concluding remarks
To conclude, all domestic livestock species, equine, cats and dogs can be affected by members of the Strongyloides genus. Generally, these infections are of low relative importance compared with other (for example, Clade V) nematode species. In livestock and equines, this is because Strongyloides infections are probably not as common as they used to be due to extensive use of macrocyclic lactone anthelmintics that prevent and/or reduce the important route of vertical transmission via the milk after parturition. Furthermore, unlike other gastrointestinal nematode species in domestic animals, young hosts develop protective immunity rapidly to these parasites so that the effects are generally short lived and immature hosts play a negligible part in the transmission cycle. Nevertheless, infections are not uncommon in colder temperate climates in livestock and equines and in all climates in dogs. Further, low-input and organic livestock production systems, where use of macrocyclic lactones is either banned or limited, or management systems applying selective targeted treatments may experience more intense transmission and higher risk of disease. However, as mentioned above, to date the limited evidence available does not indicate increased prevalence on organic pig farms. Clinical cases, when they occur, are usually only seen in very young animals due to the acquisition of high levels of infection, which can be associated with low levels of hygiene and/or maceration of pedal skin. The subclinical impact of early life infection with these nematodes is largely unknown and deserves further investigation, including studies on the putative increase in prevalence in foals. Future prevalence studies need to include molecular typing of different Strongyloides species in relation to different hosts e.g., the uncertainties regarding species (or subtypes) of Strongyloides that are exchanged between different ruminant species. Also, more research is urgently needed on the potential zoonotic capacity of Strongyloides from dogs and cats based on molecular typing, detailed information on risk factors and mapping of transmission routes.
FINANCIAL SUPPORT
This research received no specific grant from any funding agency, commercial or not-for-profit sectors.