No CrossRef data available.
Article contents
Accepted manuscript
Parasite species co-occurrence patterns on North American red squirrels (Tamiasciurus hudsonicus)
Published online by Cambridge University Press: 18 November 2024
Abstract
An abstract is not available for this content so a preview has been provided. As you have access to this content, a full PDF is available via the ‘Save PDF’ action button.
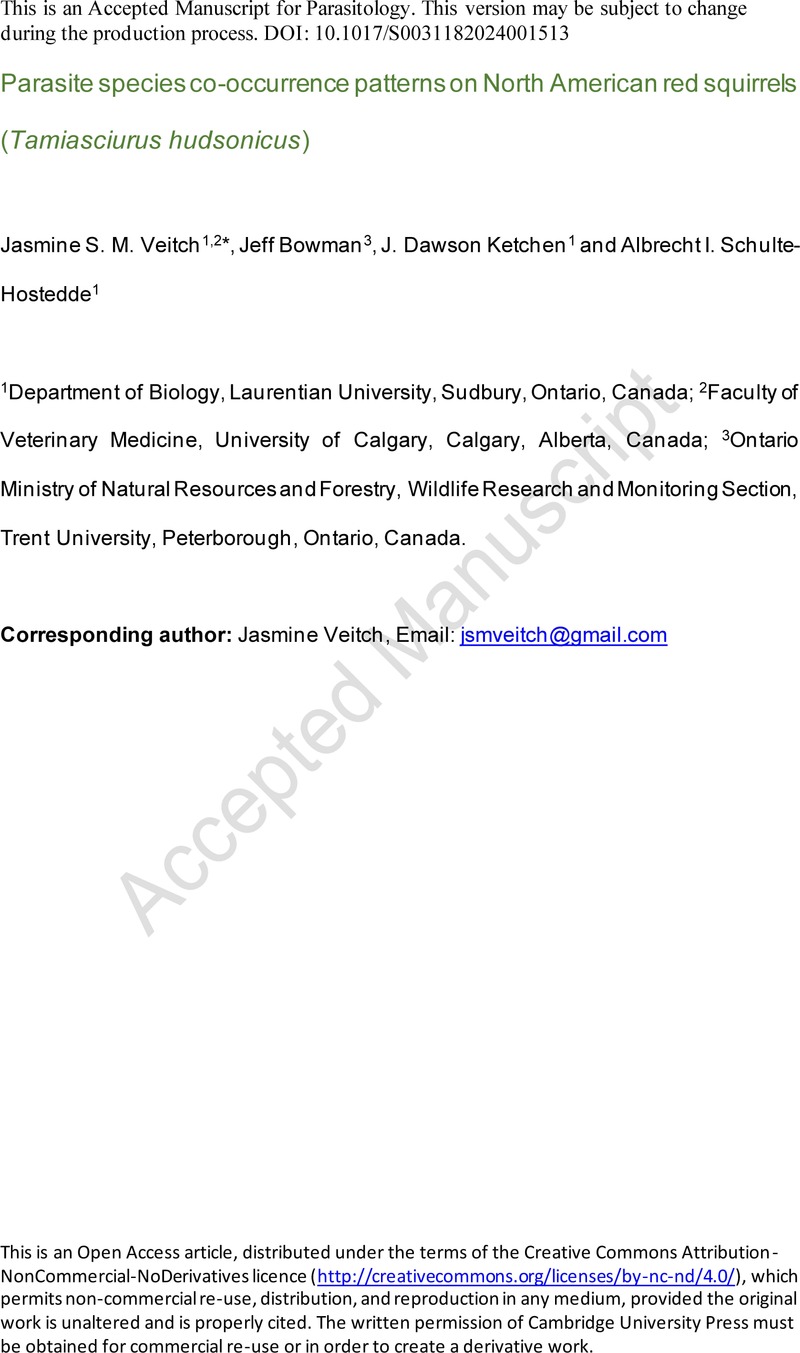
- Type
- Research Article
- Information
- Creative Commons
- This is an Open Access article, distributed under the terms of the Creative Commons Attribution-NonCommercial-NoDerivatives licence (http://creativecommons.org/licenses/by-nc-nd/4.0/), which permits non-commercial re-use, distribution, and reproduction in any medium, provided the original work is unaltered and is properly cited. The written permission of Cambridge University Press must be obtained for commercial re-use or in order to create a derivative work.
- Copyright
- Copyright © The Author(s), 2024. Published by Cambridge University Press