Introduction
Mytilus spp. are a group of bioindicator species of great importance as they are widely used as sentinel organisms in pollution monitoring programmes to determine the health status of coastal ecosystems (Nasci et al., Reference Nasci, Nesto, Monteduro and Da Ros2002; Brenner et al., Reference Brenner, Broeg, Frickenhaus, Buck and Koehler2014). They also play a key ecological role in rocky shore communities as they provide essential ecological services such as food and habitat to a multitude of other species and their commercial importance is high among aquaculture products (Beyer et al., Reference Beyer, Green, Brooks, Allan, Ruus, Gomes, Bråte and Schøyen2017). Thus, their population dynamics and the different biotic and abiotic factors that explain stock fluctuation are of importance. Among the factors which drive mussel population dynamics, parasitism can play an important role (Thieltges, Reference Thieltges2006). Consequently, parasitological studies have been widely performed with the aim of investigating the prevalence and pathology of parasitic infections (Villalba et al., Reference Villalba, Mourelle, Carballal and Lopez1997; Özer and Güneydağ, Reference Özer and Güneydağ2015; Vázquez et al., Reference Vázquez, Frizzera and Cremonte2020; Benito et al., Reference Benito, Paleček, Lekube, Izagirre, Marigómez, Zaldibar and Soto2022).
Parasites may influence the composition, structure and dynamics of their hosts’ populations in an important way affecting marine coastal ecosystems (Mouritsen and Poulin, Reference Mouritsen and Poulin2010; Thieltges et al., Reference Thieltges, Engelsma, Wendling and Wegner2013; Feis et al., Reference Feis, Thieltges, Olsen, de Montaudouin, Jensen, Bazaïri, Culloty and Luttikhuizen2015; Galaktionov et al., Reference Galaktionov, Bustnes, Bårdsen, Wilson, Nikolaev, Sukhotin, Skírnisson, Saville, Ivanov and Regel2015; Thieltges et al., Reference Thieltges, Mouritsen, Poulin and Beninger2018; Stout et al., Reference Stout, Garenne and de Montaudouin2022). Among parasites, trematodes are especially prevalent in coastal ecosystems. In particular, trematode parasites using molluscs as first intermediate hosts are common in boreal and Arctic intertidal ecosystems (Galaktionov, Reference Galaktionov1996). Typically, the cercariae are emitted by the first intermediate host and infect the second intermediate hosts (various invertebrates or fishes) in which they settle as metacercariae. The metacercariae develops into egg-producing adults after being ingested by the final hosts, which are vertebrates and, in some species, marine birds (Galaktionov et al., Reference Galaktionov, Bustnes, Bårdsen, Wilson, Nikolaev, Sukhotin, Skírnisson, Saville, Ivanov and Regel2015). The high concentrations of both predators and prey, which act as hosts at different life stages and are common in the intertidal and upper subtidal zones, are conducive to the occurrence of parasites with complex life cycles (Galaktionov et al., Reference Galaktionov, Bustnes, Bårdsen, Wilson, Nikolaev, Sukhotin, Skírnisson, Saville, Ivanov and Regel2015). Among trematode parasites infecting bivalves, those in the Digenea subclass are the most common, including members of the Gymnophallidae (Cremonte et al., Reference Cremonte, Gilardoni, Pina, Rodrigues and Ituarte2015; Magalhães et al., Reference Magalhães, Daffe, Freitas and de Montaudouin2020; Puljas and Burazin, Reference Puljas and Burazin2022; Marchiori et al., Reference Marchiori, Quaglio, Franzo, Brocca, Aleksi, Cerchier, Cassini and Marcer2023). The final host of Gymnophallidae parasites are coastal birds, mostly charadriforms and anseriform species. While bivalves are the first intermediate hosts, bivalves, gastropods, polychaetes and brachiopods act as second intermediate hosts (Cremonte et al., Reference Cremonte, Gilardoni, Pina, Rodrigues and Ituarte2015; Marchiori et al., Reference Marchiori, Quaglio, Franzo, Brocca, Aleksi, Cerchier, Cassini and Marcer2023).
Infection by gymnophallids in molluscan hosts has a wide array of pathogenic effects which also depend on the parasitic stage (sporocysts or metacercariae). In the second intermediate host, metacercariae can initiate the formation of pearls, causing one of the greatest commercial damage in mussels (Stunkard and Uzmann, Reference Stunkard and Uzmann1958; Gosling, Reference Gosling2015; Marchiori et al., Reference Marchiori, Quaglio, Franzo, Brocca, Aleksi, Cerchier, Cassini and Marcer2023). Pearl formation is a defence mechanism by mussel mantle which is activated by exogenous organic (e.g. parasite) and inorganic materials and that ends isolating this material through conchiolin encapsulation. The host reaction to the trematodes larval stage infection leads to the death of the parasite by the calcification of its body in the pearl centre (Marchiori et al., Reference Marchiori, Quaglio, Franzo, Brocca, Aleksi, Cerchier, Cassini and Marcer2023). Different metacercariae infections are associated with changes in mantle tissue due to degenerative and inflammatory processes, including compression and displacement of gonads with consequent decrease in reproductive potential and pearl formation (Gosling, Reference Gosling2015).
In early autumn 2016 and late summer 2017, alleged gymnophallid metacercariae were observed together with pearls in the mantle of mussels collected in Iceland and Tromsø (Benito et al., Reference Benito, Paleček, Lekube, Izagirre, Marigómez, Zaldibar and Soto2022). The aim of the present study was to identify the trematode species corresponding to metacercariae found in mussels collected from Tromsø at morphological and molecular levels, and analyse the relationship between the gymnophallid parasite and the biological material encapsulated inside the pearls. For that purpose, morphological analysis of the metacercariae were carried out by histology and DNA analysis was performed on isolated metacercariae and pearl powder.
Material and methods
Host sampling, dissection and parasite dissection
Mussels (Mytilus edulis) were collected from a rocky beach (69.642089 N, 18.94639 E) and a harbour (69.654177 N, 18.968459 E) in Tromsø, Norway, on 20/10/2016. Individuals ranged 2–4.5 cm in shell length and were collected from the lower first metre of the intertidal zone. Mussels were transported to the laboratory at ambient temperature. Mussels used for histological analysis were dissected and a transverse section from each one, including mantle, gills and digestive gland tissues, was fixed in formalin. The rest of the histological procedure was carried out as indicated in Benito et al. (Reference Benito, Paleček, Lekube, Izagirre, Marigómez, Zaldibar and Soto2022). Frozen mussels and paraffin blocks were stored in the Biscay Bay Environmental Biospecimen Bank. In this particular case, a paraffin block corresponding to a mussel that presented heavy metacercarial infection was selected and serial consecutive sections (5 μm thick) were performed, getting serial sections of 2 different metacercariae, named A and B (‘A’ metacercariae: coronal sections and ‘B’ metacercariae: sagittal sections).
For parasite dissection, whole mussels were deep frozen (−80°C) and stored until dissection. After thawing the mussels (n = 15), mantle tissue was dissected out and placed between 2 glass plates, which were used to apply pressure on the tissue in order to locate the parasites under the stereomicroscope (Nikon SMZ800). Once located, metacercariae and pearls (pearls were retrieved from the mussels before using the glass plates in order to avoid breaking them) were carefully isolated with the aid of fine forceps and brushes. Parasites for molecular identification were deep frozen again, and the metacercariae for morphological identification (n = 13) were measured at the microscope (Olympus BX61) with attached camera (Olympus DP74) using the Olympus CellSens Entry 2.2 software. After removing the remaining soft tissues of the hosts, pearls were air dried and stored in Eppendorf tubes at room temperature until processing.
DNA extraction and PCR amplification
Pearls were individually grounded in an agate hand mortar and the fine powder was collected and placed into Eppendorf tubes. To avoid cross–contamination, all the tools used to grind the pearls were cleaned with bleach diluted to 10%. DNA extraction of the pearls was carried out using the DNeasy blood and tissue kit (Qiagen, Germany), and DNA was eluted in RNAse-free water.
For parasite DNA extraction, Trizol (Invitrogen, California, USA) method was used following manufacturer's instructions. DNA was dissolved in RNAse-free water. Conventional PCR was performed in SimplyAmp Applied Biosystems (AB, Waltham, Massachusetts, USA) thermocycler using NZYTECH 2X Green Taq polymerase master mix with 0.1 μM concentrations of Fw an Rv primers of 18S rDNA, COI and ITS genes (Table 1). PCR final reaction volume was 25 μL, being 2 μL of DNA template. Briefly, the reaction was as follows: Taq polymerase activating temperature step of 95°C for 2 min, followed by denaturation step at 94°C for 30 s, an annealing temperature of Tm (Table 1) for 30 s, ending with an elongation step of 72°C for 30 s. These 3 steps were repeated 35 times. The reaction ended at 72°C for 7 min, and kept at 4°C until electrophoresis was performed. In the case of pearl powder extract samples, mentioned PCR was repeated on top of the PCR product (volume used as template in the second PCR, 1 μL). All conventional PCR reactions were performed with a non-template control containing water as template. All amplicons were visualized in 2% agarose gels stained with real safe. Samples positive for PCR results were sequenced, for both strands, using Sanger procedures in the Sequencing and Genotyping Unit of the SGIker of the University of the Basque Country (UPV/EHU).
Table 1. List of primers used for PCR amplification in Mytilus edulis pearls and parasite samples
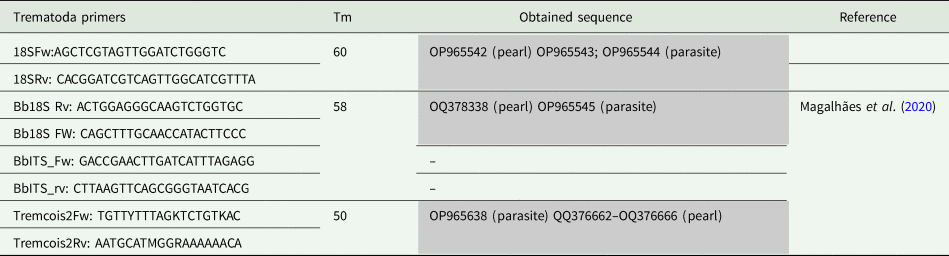
Primers melting temperature (Tm), obtained and published sequences, and references for used primers are indicated. No reference means primers designed by the authors.
Bioedit was used to visualize sequencing quality of the Sanger files. Forward and reverse Fasta sequences of the same sequence were clustered together, primer section trimmed and consensus sequences built using IUPAC nomenclature for undetermined bases. Consensus sequences were used to search for sequence similarities in NCBI GenBank using BlastN (database of 30/11/2022).
Results
Morphological description of the metacercariae
Body oval or pyriform (Figs 1A, B and 2A) presenting a spiny tegument (Fig. 2B). Metacercariae displayed a subterminal and muscular oral sucker, similar in size to the ventral sucker. Well-developed muscular pharynx. Digestive caeca reached the posterior third part of body, where the ventral sucker was located. Inconspicuous genital pore (Fig. 3A), pars prostatica (Fig. 3C) and genital atrium were only found in histological samples (Fig. 3B). Excretory vesicle Y-shaped reaching pharyngeal level. Morphometric data are presented in Table 2 as mean value (± standard deviation): metacercariae length 669.54 (±66.01) μm, metacercariae width 380.88 (±43.13) μm, oral sucker dimensions 121.11 (±13.09) × 110.23 (±11.92) μm and ventral sucker dimensions 123.02 (±22.29) × 112.23 (±15.37) μm. More detailed morphometric data are shown in Table 2.
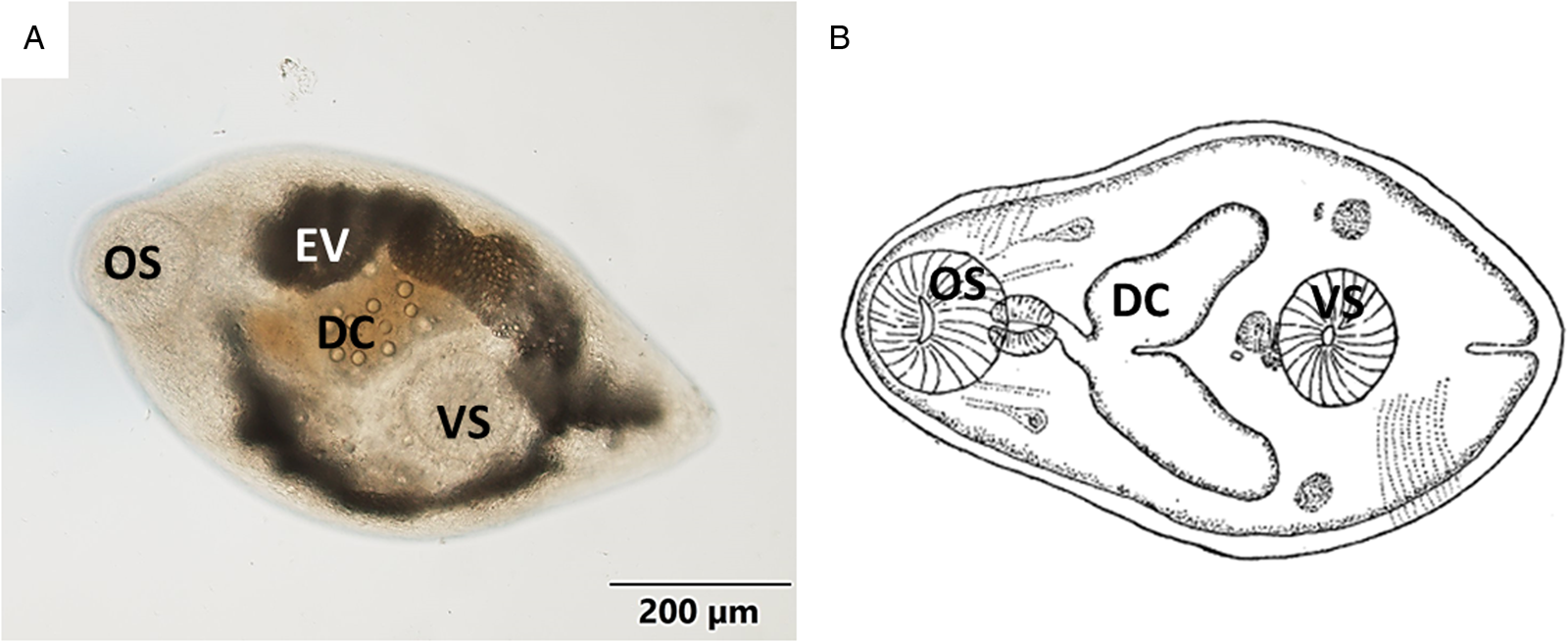
Figure 1. (A) Micrograph of the general view of a metacercariae isolated from mantle tissue. (B) Schematic drawing of Gymnophallus bursicola metacercariae from Stunkard and Uzmann (Reference Stunkard and Uzmann1958). OS, oral sucker; EV, excretory vesicle; DC, digestive caeca; VS, ventral sucker.
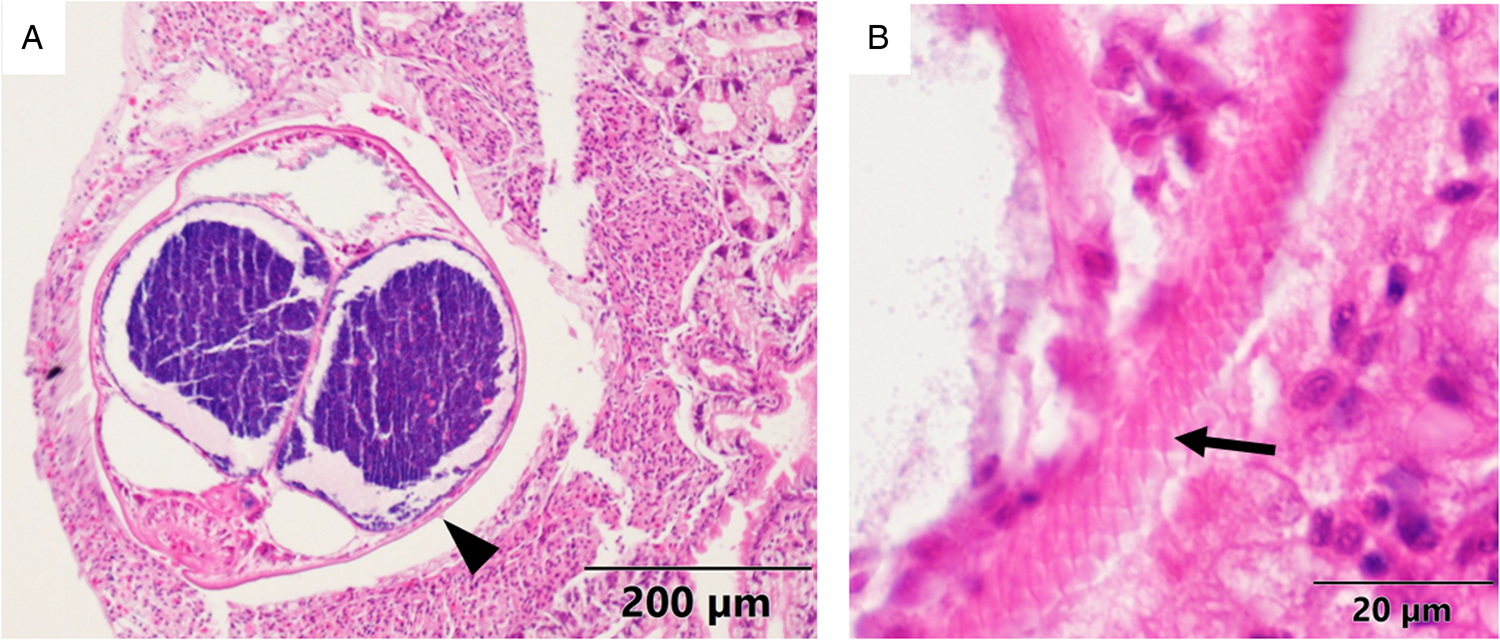
Figure 2. Histological micrographs (haematoxylin and eosin) of a metacercariae encapsulated in mantle tissue. (A) General view of the ‘A’ metacercariae (arrowhead). (B) Detailed view of the spiny tegument (arrow) of the ‘B’ metacercariae.

Figure 3. Histological micrographs (haematoxylin and eosin) in which genital traits of metacercariae can be visualized. (A, B) Serial sections of the ‘B’ metacercariae in which the genital pore and genital atrium can be distinguished. (C) A section of the ‘A’ metacercariae in which the pars prostatica can be appreciated. VS, ventral sucker; DC, digestive caeca; GA, genital atrium. Asterisk: genital pore; arrow: pars prostatica; arrowhead: prostatic cells.
Table 2. Morphometrics of the metacercariae analysed in the present work compared to morphometrics of Gymnophallus bursicola collected from Mytilus edulis in Massachusetts described by Stunkard and Uzmann (Reference Stunkard and Uzmann1958), Parvatrema sp. metacercariae collected from Mytilus galloprovincialis in the Adriatic sea in 2 different studies (Puljas and Burazin, Reference Puljas and Burazin2022; Marchiori et al., Reference Marchiori, Quaglio, Franzo, Brocca, Aleksi, Cerchier, Cassini and Marcer2023)

Data from the present study and Puljas and Burazin (Reference Puljas and Burazin2022) are presented as range (mean ± s.d.), data from Stunkard and Uzmann (Reference Stunkard and Uzmann1958) are presented as range, data from Marchiori et al. (Reference Marchiori, Quaglio, Franzo, Brocca, Aleksi, Cerchier, Cassini and Marcer2023) are presented as mean ± s.d. Asterisk indicates that the measurement was done on a fixed individual.
Metacercariae and pearl prevalence
Multiple metacercariae and pearls were found in 62.5% of the dissected mussels (n = 16). The size of the pearls ranged from roughly the same size as the metacercariae (300–700 μm) up to 2980 × 2400 μm. Remarkably, a dead metacercaria surrounded by host haemocytes and chitin deposits was observed too in a histological section (Fig. 4A). While dissecting, some pearls were seen attached to the nacreous layer of the inner side of mussel valves (Fig. 4B).

Figure 4. (A) Histological micrograph (haematoxylin and eosin) in which a dead metacercariae (arrowhead) can be seen surrounded by layers of haemocytes (arrows) and chitin (asterisk). (B) Photograph of 2 pearls embedded in the nacreous layer (NL) of a mussel shell.
Pearl DNA extraction
DNA was successfully extracted from the powder of the 16 pearls tested, with an average concentration of 80.74 ng μL−1 ± 34.38. The 260/280 DNA quality ratio measured spectrophotometrically ranged from 1.56 to 1.89.
PCR amplification of 18s rDNA
Both pearl powder and parasites positively amplified 18S rDNA using primers from Magalhães et al. (Reference Magalhães, Daffe, Freitas and de Montaudouin2020). Five sequences were obtained, 2 in the pearl extract (ID: OP965542 and OQ378338) and the rest in isolated parasites (ID: OP965543; OP965544 and OP965545) being each one of 514, 596, 540, 450 and 504 bp in length, respectively. It is worth mentioning that out of all tested pearls, 14 were positive for 18S rDNA amplicons, however, only 2 were sequenced with homology related to parasitic microorganism; the others corresponded to M. edulis 18S rDNA sequence. BlastN results of OP965542 and OQ378338 showed strong similarity with digenean trematodes, particularly with the genus Gymnophallus. Detailed information of the identity percentages of 18S rDNA sequences is given in Table 3.
Table 3. Identity percentage (%) comparison among the 18S rDNA sequences from the present work and the top 4 most similar species: G. choledochus (MN544854); M. minutus (MN879355); G. seoi (JQ955636.1); G. deliciosus (OM699016.1)

Bold numbers indicate values higher than 97% of identity. Asterisk: highest identity percentage among Gymnophalus species.
The 3 sequences from parasites were 100% identical, being different in length. The 18S rDNA sequences obtained from the pearl extract, overall, had lower Sanger sequencing quality, and contained 5 undetermined bases. Nonetheless, pearl sequences were identical to the parasite ones (Table 3).
COI primers also amplified both, parasite and pearl DNA extract. The consensus sequence for parasite (ID: OP965638; 259 bp in length) was most similar to G. choledochus (MN547969.1) with an identity 85% and 1e−73 e-value, followed by Renicola buchanani (KF512572) with an 84% of identity and 5e−66 e-value. In the case of the pearls, PCR cycles were increased. Out of the 16 pearls, 7 were positive to COI. However, only 5 were uploaded in NCBI for similarity searching due to sequence quality issues (ID: OQ376662–OQ376666; length range: 330–386 bp). Blast homology results of pearl's COI amplicon displayed lowest similarity e-values with species different from the parasite amplified COI: Plagiorchis sp (MW520081; 2e−93); R. buchanani (KF512572), 3e−90 and Neogplagioporus elongates (LC196193) 4e−89. However, the highest identity of percentage 83.21% was with Gymnophallus choledochus (MN547969) that was the fourth hit of the blastN with an e-value of 9e−71. Regarding ITS primers, they did not amplify either the parasite or the pearl materials.
Discussion
The aims of the present study were (1) to identify the parasite infecting mussel mantle in Tromsø as metacercariae, and (2) to decipher whether the pearls observed in the mantle of blue mussels (M. edulis) were produced by the parasitic infection that was causing histopathological alterations. Molecular and histological procedures confirmed causation of pearl formation and the presence of digenean parasites.
According to the morphological and anatomical characters, including the taxonomic key proposed by Cremonte et al. (Reference Cremonte, Gilardoni, Pina, Rodrigues and Ituarte2015), detected metacercariae were identified as likely members of the genus Gymnophallus. This idea reinforced by morphometric data and by comparison with the schematic drawings published by Stunkard and Uzmann (Reference Stunkard and Uzmann1958) of Gymnophallus bursicola. Moreover, Galaktionov et al. (Reference Galaktionov, Bustnes, Bårdsen, Wilson, Nikolaev, Sukhotin, Skírnisson, Saville, Ivanov and Regel2015) indicated that G. bursicola is the only species of the Gymnophallus genus present in European mussels. Presently, there is compelling evidence suggesting that the metacercariae found in blue mussels (M. edulis) collected from Tromsø belong to the G. bursicola species. Nevertheless, there are anatomical traits defined in the bibliography that could not be assessed with absolute certainty (i.e. the identification of genital traits). This uncertainty could be due to the non-optimal condition of the specimens as they were thawed for testing. In addition, analysed metacercariae were almost 3 times larger than the ones described in the recent bibliography, which could have hindered the observation of the inner organs by light microscopy. Lastly, according to the descriptions of Stunkard and Uzmann (Reference Stunkard and Uzmann1958), the metacercariae analysed in the present study might be a very immature asexual stage of G. bursicola, and consequently they would present underdeveloped genital systems important for accurate identification.
Morphological studies need to be coupled with molecular tools to safely develop quick and precise diagnostics. The approach used herein has allowed morphological discrimination of a parasite species, and confirming its presence based on molecular evidence. Although no G. bursicola sequence was available in NCBI, consequently, the morphological identification could not be molecularly linked to an existing sequence.
The infection prevalence reported in the present study is coherent with data reported by Galaktionov et al. (Reference Galaktionov, Bustnes, Bårdsen, Wilson, Nikolaev, Sukhotin, Skírnisson, Saville, Ivanov and Regel2015), which ranged from 46.7% to 51.6% in Tromsø, but went up to 83.9% in Iceland. The prevalence data reported by Benito et al. (Reference Benito, Paleček, Lekube, Izagirre, Marigómez, Zaldibar and Soto2022) in histological samples of mussels collected in the same sampling sites as in the present study were lower (15.79–31.58%) than that reported in the present study. This decrease might indicate an underestimation of parasite prevalence and infection intensity when using only histological techniques. The results of the present study together with the histopathological analysis performed in previous studies (Benito et al., Reference Benito, Paleček, Lekube, Izagirre, Marigómez, Zaldibar and Soto2022), and the negative effects described in Mediterranean mussel populations caused by Gymnophallid trematodes (Puljas and Burazin, Reference Puljas and Burazin2022) make this family a subject of great parasitological interest.
Previous studies indicated Gymnophallid trematodes as possible inducers of pearl formation in mussels (Ituarte et al., Reference Ituarte, Cremonte and Zelaya2005; Marchiori et al., Reference Marchiori, Quaglio, Franzo, Brocca, Aleksi, Cerchier, Cassini and Marcer2023). The formation of the pearls is the result of the response of the host to any tissue-invading foreign bodies in an attempt to encapsulate them when they cannot be phagocytized. The encapsulating haemocytes release cytotoxic products to destroy the parasite and create a multicellular chitinous capsule that adheres and encloses the pathogen (Lauckner, Reference Lauckner and Kinne1983; De Vico and Carella, Reference De Vico and Carella2012; Gao et al., Reference Gao, Liao, Wang, Bao, Fan, Li, Wu and Xia2015; Marchiori et al., Reference Marchiori, Quaglio, Franzo, Brocca, Aleksi, Cerchier, Cassini and Marcer2023). The mucous layer surrounding the parasite, as seen under the microscope (Fig. 4A), constitutes a matrix for the deposition of calcareous material (Marchiori et al., Reference Marchiori, Quaglio, Franzo, Brocca, Aleksi, Cerchier, Cassini and Marcer2023). In addition, in the present study, pearls were found embedded in the nacreous layer of the shell of infected mussels (Fig. 4B), which indicates that pearls end up trapped in the mineral matrix while the CaCO3 is being deposited on the shell surface during the growth and thickening processes of the shell. Overall, the described defence system that includes cytotoxic action and organic and inorganic encapsulation of the parasites can be an important stress source for Mytilus species, which makes it a key pathological phenomenon that should be taken into account for the assessment of the health status of wild and cultured mussels.
In the Norwegian coast, high prevalence of pearls in mussels was reported in 2018 (Bråte et al., Reference Bråte, Buenaventura and Lusher2018), with some mussels including more than 360 pearls per individual. Bråte et al. (Reference Bråte, Buenaventura and Lusher2018) found no correlation between the occurrence of microplastics and pearls. Although they hypothesized that the presence of parasites is an aetiological factor for pearl formation, they did not present any evidence. Thus, as suggested by Benito et al. (Reference Benito, Paleček, Lekube, Izagirre, Marigómez, Zaldibar and Soto2022), it seems clear that the importance of parasitological studies for the health status assessment of mussels is critical. Co-occurrence of pearl formation and Gymnophalidae infection has been recently observed in Mediterranean mussels (Puljas and Burazin, Reference Puljas and Burazin2022; Marchiori et al., Reference Marchiori, Quaglio, Franzo, Brocca, Aleksi, Cerchier, Cassini and Marcer2023). Furthermore, when comparing the morphometric and anatomical characteristics with the cited Mediterranean studies, it is clear that the metacercariae infecting Mediterranean mussels and Northern Atlantic mussels (the ones in the present study) belong to different species. These facts make the presence of pearls in Mytilus spp. and their aetiology a significant research subject, at least, at European level.
The present study confirms that the pearls are formed by the encapsulation of gymnophallid metacercariae. In previous studies, pearl (host) DNA had been only extracted to determine the origin of cultivable pearls (Meyer et al., Reference Meyer, Cartier, Pinto-Figueroa, Krzemnicki, Hänni and McDonald2013). The positive amplification of the parasitic DNA encapsulated inside the pearls reported in the present study, as far as the authors are aware, is a novel achievement.
In conclusion, the present study combines morphological, histological and molecular approaches for the characterization of G. bursicola metacercariae infecting Norwegian M. edulis, allowing the authors to undoubtedly report the first 18S rDNA and COI sequences for the mentioned species. In addition, parasite DNA from the core of the pearls was extracted and amplified for the first time, which confirmed the parasitological origin of these pearls and allows the possibility of identifying different parasitic organisms that cause the generation of pearls in bivalves.
Data availability statement
The data that support the findings of this study are available from the corresponding author, [D.B.], upon reasonable request.
Acknowledgements
SGIker technical support (University of the Basque Country) is greatly acknowledged. Thanks are also due to Perrine Geraudie from Akvaplan-Niva for her tremendous support.
Author contributions
Denis Benito, Urtzi Izagirre, Xabier Lekube, Beñat Zaldibar, Manu Soto and Oihane Diaz de Cerio conceived and designed the study. Denis Benito, Oihane Diaz de Cerio, Xavier De Montaudouin and Guillemine Daffe conducted data gathering. Denis Benito, Urtzi Izagirre, Xabier Lekube, Beñat Zaldibar, Antonio Villalba, Xavier De Montaudouin, Guillemine Daffe, Manu Soto and Oihane Diaz de Cerio wrote and revised the article.
Financial support
This study was funded by the Basque Government (grant to consolidated research groups IT1302-19) and EU GRACE Project (grant agreement number 679266).
Competing interests
None.
Ethical standards
Not applicable.