Introduction
The application of molecular tools for characterisation and phylogenetic analyses has greatly advanced our understanding of the diversity, taxonomy, systematics and phylogeny of virtually all major groups of parasitic worms. Molecular data have become a ‘must-have’ characteristic not only in species discovery and delineation but also in large-scale biodiversity inventories, and ecological and evolutionary research. This is especially true for the trematode subclass Digenea, parasitic flatworms representing a remarkable example of the diversity of complex life-cycles among the Metazoa (Minelli and Fusco, Reference Minelli and Fusco2010), which involve alternation of generations and a diversity of phenotypes in the sequential hosts in the life-cycle.
The digenean genus Diplostomum von Nordmann, 1832 (Diplostomidae) has received increased attention in recent years. Intensive sampling and molecular analyses predominantly of the larval stages of Diplostomum spp. from their intermediate hosts, freshwater lymnaeid snails and fishes, have resulted in delineation of more than 40 species/species-level lineages (Locke et al., Reference Locke, McLaughlin, Dayanandan and Marcogliese2010a, Reference Locke, McLaughlin and Marcogliese2010b, Reference Locke, Al-Nasiri, Caffara, Drago, Kalbe, Lapierre, McLaughlin, Nie, Overstreet, Souza, Takemoto and Marcogliese2015, Reference Locke, Drago, Núñez, Souza and Takemoto2020; Georgieva et al., Reference Georgieva, Soldánová, Pérez-del-Olmo, Dangel, Sitko, Sures and Kostadinova2013; Blasco-Costa et al., Reference Blasco-Costa, Faltýnková, Georgieva, Skírnisson, Scholz and Kostadinova2014; Faltýnková et al., Reference Faltýnková, Georgieva, Kostadinova, Blasco-Costa, Scholz and Skírnisson2014; Pérez-del-Olmo et al., Reference Pérez-del-Olmo, Georgieva, Pula and Kostadinova2014; Selbach et al., Reference Selbach, Soldánová, Georgieva, Kostadinova and Sures2015; Kudlai et al., Reference Kudlai, Oros, Kostadinova and Georgieva2017; Soldánová et al., Reference Soldánová, Georgieva, Roháčová, Knudsen, Kuhn, Henriksen, Siwertsson, Shaw, Kuris, Amundsen, Scholz, Lafferty and Kostadinova2017; Gordy and Hanington, Reference Gordy and Hanington2019; Hoogendoorn et al., Reference Hoogendoorn, Smit and Kudlai2020; Lebedeva et al., Reference Lebedeva, Chrisanfova, Ieshko, Guliaev, Yakovleva, Mendsaikhan and Semyenova2021). Complete mitochondrial genomes have been characterised for four species, Diplostomum spathaceum (Rudolphi, 1819) and D. pseudospathaceum Niewiadomska, 1984 (see Brabec et al., Reference Brabec, Kostadinova, Scholz and Littlewood2015), D. ardeae Dubois, 1969 (see Locke et al., Reference Locke, Drago, Núñez, Souza and Takemoto2020) and Diplostomum baeri Dubois, 1937 (see Landeryou et al., Reference Landeryou, Kett, Ropiquet, Wildeboer and Lawton2020).
However, the number of named molecularly characterised species remains low because of the difficulties in gathering adult worms from their definitive hosts (fish-eating birds) and the virtual lack of taxonomic expertise in identification of the larval stages. These include D. spathaceum (Rudolphi, 1819) (type-species), D. ardeae Dubois, 1969, D. baeri sensu Galazzo et al. (Reference Galazzo, Dayanandan, Marcogliese and McLaughlin2002), D. huronense (La Rue, 1927), D. indistinctum (Guberlet, 1922), D. lunaschiae (Locke, Drago, Núñez, Rangel e Souza & Takemoto, Reference Locke, Drago, Núñez, Souza and Takemoto2020), D. pseudospathaceum Niewiadomska, 1984 and D. parviventosum Dubois, 1932. Phylogenetic analyses have depicted two species complexes among the prevailing unnamed species-level lineages. The D. mergi complex comprises one named species and three species-level lineages (D. parviventosum; D. mergi Lineages 2 and 3 of Georgieva et al. (Reference Georgieva, Soldánová, Pérez-del-Olmo, Dangel, Sitko, Sures and Kostadinova2013); and D. mergi Lineage 4 of Selbach et al. (Reference Selbach, Soldánová, Georgieva, Kostadinova and Sures2015)), and the D. baeri complex sensu Blasco-Costa et al. (Reference Blasco-Costa, Faltýnková, Georgieva, Skírnisson, Scholz and Kostadinova2014) comprises one named species and seven species-level lineages (D. baeri sensu Galazzo et al. (Reference Galazzo, Dayanandan, Marcogliese and McLaughlin2002), Diplostomum sp. Lineages 3–5 of Blasco-Costa et al. (Reference Blasco-Costa, Faltýnková, Georgieva, Skírnisson, Scholz and Kostadinova2014), Diplostomum sp. 2 of Moszczynska et al. (Reference Moszczynska, Locke, McLaughlin, Marcogliese and Crease2009), and Diplostomum spp. 5–7 of Locke et al. (Reference Locke, McLaughlin, Dayanandan and Marcogliese2010a)). Of these, seven lineages have been sequenced and morphologically characterised in Europe based on larval isolates (Blasco-Costa et al., Reference Blasco-Costa, Faltýnková, Georgieva, Skírnisson, Scholz and Kostadinova2014; Faltýnková et al., Reference Faltýnková, Georgieva, Kostadinova, Blasco-Costa, Scholz and Skírnisson2014; Selbach et al., Reference Selbach, Soldánová, Georgieva, Kostadinova and Sures2015; Lebedeva et al., Reference Lebedeva, Chrisanfova, Ieshko, Guliaev, Yakovleva, Mendsaikhan and Semyenova2021).
In a study of larval digenean communities in the snail host Ampullaceana balthica (L.) (Gastropoda: Lymnaeidae) in the River Ruhr drainage, we collected a number of cercarial isolates which at first glance resembled morphologically the known cercariae of the D. baeri species complex. However, sequencing of the cytochrome c oxidase subunit 1 (cox1) mitochondrial gene indicated high similarity with two isolates of Diplostomum phoxini (Faust, 1919) collected in Norway (Soldánová et al., Reference Soldánová, Georgieva, Roháčová, Knudsen, Kuhn, Henriksen, Siwertsson, Shaw, Kuris, Amundsen, Scholz, Lafferty and Kostadinova2017). Therefore, we sampled the specific second intermediate host of this species, Phoxinus phoxinus (L.) (Cypriniformes: Leuciscidae), and sequenced the metacercariae recovered from the brain of the fish. Here, we provide molecular and morphological characterisation of D. phoxini, one of the few species of Diplostomum exhibiting strict host specificity to the second intermediate host (P. phoxinus), and molecular evidence for the identification of its first intermediate host (A. balthica). Phylogenetic analyses revealed changes in the composition of the D. baeri and D. mergi species complexes, and resulted in a re-classification of a large number of sequenced isolates. Finally, we compare the prevalence of D. phoxini and the molecularly characterised species/lineages of the D. baeri complex in the lentic and lotic aquatic habitats of Europe.
Materials and methods
Sample collection and examination
A total of 1599 Ampullaceana balthica (Gastropoda: Lymnaeidae; formerly often reported as Radix balthica) were collected and examined for trematode infections during spring (May), summer (June, July, August), autumn (September, October, November) and winter (December) in 2016 and 2017 and from May to September in 2019. Snails were collected at three sampling sites at the River Ruhr at Neheim (North Rhine-Westphalia, Germany): B0 (51°26′24.4″N, 7°58′35.8″E); B1 (51°26′25.9″N, 7°57′50.9″E) and B2 (51°26′53.2″N, 7°57′09.5″E). All snails were collected by hand and using a strainer from stones, driftwood and macrophytes, or picked directly from the sediment in shallow, slow-moving parts of the river. In the laboratory, snails were measured (shell width and height) and placed separately in containers with filtered river water under a light source to stimulate the emergence of cercariae. All containers were checked under light microscope for three consecutive days for the presence of cercariae in the water column. On the fourth day, all snails were dissected and examined for the presence of prepatent infections (sporocysts) (as described, e.g. in Selbach et al., Reference Selbach, Soldánová, Georgieva, Kostadinova and Sures2015; Schwelm et al., Reference Schwelm, Soldánová, Vyhlídalová, Sures and Selbach2018). Additionally, 15 specimens of the Eurasian minnow Phoxinus phoxinus (L.) were sampled via electrofishing at one site of the River Ruhr at Arnsberg (B4) (51°24′04.3″N, 8°04′01.1″E) in June 2019. In the laboratory, all fish were identified using Kottelat and Freyhof (Reference Kottelat and Freyhof2007), dissected and investigated for the presence of metacercariae in the brain.
Cercariae and metacercariae were fixed in molecular grade ethanol for DNA isolation and sequencing, and in 4% formaldehyde solution for morphological analyses (scanning electron microscopy, SEM). Cercariae studied by SEM were cleaned and subsequently post-fixed in 1% osmium tetroxide for 2 h, washed in 0.1 M phosphate buffer, dehydrated in an ethanol series, critical-point dried, sputter-coated with gold and examined and photographed with a scanning electron microscope (Hitachi 4100 FE Ltd., Tokio, Japan) at 20 kV at the Central Service for Experimental Research (SCSIE), University of Valencia, Spain. Foot tissue from snails was fixed in molecular grade ethanol for molecular identification.
Morphological data
Trematode larval stages were identified live using light microscopy (Olympus BX51, Tokyo, Japan). Cercariae and metacercariae were identified to the species level based on the morphological descriptions of Arvy and Buttner (Reference Arvy and Buttner1954), Rees (Reference Rees1957) and Dönges (Reference Dönges1969a, Reference Dönges1969b). Series of detailed light microscopy photographs of cercariae and metacercariae of D. phoxini were taken with a digital camera (Olympus UC30, Tokyo, Japan) attached to the light microscope and all visible features were recorded. Descriptions of the cercariae are based on examination of live material and digital photomicrographs from both, light microscopy and SEM. Measurements were taken with the program ImageJ 1.47v (available from https://imagej.nih.gov/ij/download.html) and are given in micrometres as the range followed by the mean in parentheses. The following abbreviations were used in the description of the cercaria: AOW, anterior organ width; BL, body length; FL, furca length; TSL, tail stem length; VSW, ventral sucker width.
Molecular data
Total genomic DNA (gDNA) was isolated from ethanol-fixed snail tissue, pooled samples of 10–15 cercariae or single metacercariae by placing the samples in 200 μL of a 5% suspension of deionised water and Chelex®, containing 0.1 mg mL−1 proteinase K, followed by incubation at 56°C for 3 h, boiling at 90°C for 8 min, and centrifugation at 14 000× g for 10 min. Polymerase chain reaction (PCR) amplification was carried out using 2× MyFi Mix (Meridian Bioscience, Cincinnati, USA), 8 pmol of each primer and c.50 ng of gDNA in a total volume of 20 μL. Partial fragments of the mitochondrial cytochrome c oxidase subunit 1 (cox1) gene, the nuclear 28S rRNA gene and the complete ITS1-5.8S-ITS2 gene cluster (ITS2 only for A. balthica) were sequenced for the snail host and larval stages of D. phoxini using the primers and cycling conditions listed in Online Resource Table S1.
PCR amplicons were purified using QIAquick PCR purification kit (Qiagen Ltd, Hilden, Germany) following the manufacturer's instructions. PCR fragments were sequenced directly with ABI BigDye chemistry (ABI Perkin-Elmer, UK), alcohol-precipitated and run on an ABI Prism 3730XL DNA analyser using the primers listed in Online Resource Table S1.
Newly generated and published sequences were aligned with MAFFT v.7 (Kuraku et al., Reference Kuraku, Zmasek, Nishimura and Katoh2013; Katoh et al., Reference Katoh, Rozewicki and Yamada2019). The cox1 sequences were aligned with reference to the amino acid translation, using the echinoderm and flatworm mitochondrial code (translation table 9; Telford et al., Reference Telford, Herniou, Russell and Littlewood2000) for parasite isolates and the invertebrate mitochondrial code (translation table 5) for snail host isolates; the alignments contained no insertions or deletions.
Molecular identification/delimitation of the snail and parasite samples was achieved using neighbour-joining (NJ) analyses of Kimura 2-parameter distances for the cox1 alignments conducted with MEGA v.7 (Kumar et al., Reference Kumar, Stecher and Tamura2016); nodal support was estimated using 1000 bootstrap replicates. Genetic distances (uncorrected p-distance) were calculated with MEGA v.7. Non-metric multidimensional scaling (NMDS) plot was generated with Primer v.6 software (Anderson et al., Reference Anderson, Gorley and Clarke2008) to visualize the raw pairwise distances between species/lineages of the D. baeri species complex. Unique haplotypes were identified with DnaSP (Rozas et al., Reference Rozas, Sanchez-DelBarrio, Messeguer and Rozas2003) against the recently published sequences for D. phoxini by Lebedeva et al. (Reference Lebedeva, Chrisanfova, Ieshko, Guliaev, Yakovleva, Mendsaikhan and Semyenova2021).
Species relationships within Diplostomum were assessed using Bayesian inference (BI) analysis of cox1 data. Prior to analysis, the best-fitting model of nucleotide substitution (HKY + Г + I) was estimated based on the Bayesian information criterion (BIC) using jModelTest v. 2.1.4 (Darriba et al., Reference Darriba, Taboada, Doallo and Posada2012). BI analysis was carried out with MrBayes v. 3.2.7 (Ronquist et al., Reference Ronquist, Teslenko, Van Der Mark, Ayres, Darling, Höhna, Larget, Liu, Suchard and Huelsenbeck2012) on the CIPRES Science Gateway v.3.3 (Miller et al., Reference Miller, Pfeiffer and Schwartz2010) using Markov chain Monte Carlo searches on two simultaneous runs of four chains for 107 generations, sampling trees every 103 generations. The ‘burn-in’ determined by stationarity of lnL assessed with Tracer v.1.5 (http://beast.bio.ed.ac.uk/Tracer) was set for the first 25% of the trees sampled, and a consensus topology and nodal support estimated as posterior probability values (Huelsenbeck et al., Reference Huelsenbeck, Ronquist, Nielsen and Bollback2001) were calculated from the remaining trees. Phylogenetic trees were visualised and finalised in FigTree v. 1.4.4 (http://tree.bio.ed.ac.uk/software/figtree/.)).
Results
Prevalence of Diplostomum phoxini in the intermediate hosts
A total of 1599 Ampullaceana balthica representing 41 distinct individual samples (i.e. collected at a given place and date, as per the definition of Bush et al., Reference Bush, Lafferty, Lotz and Shostak1997) were collected in the River Ruhr at Neheim from May to December during 2016, 2017 and 2019, and examined for prepatent and patent infections with Diplostomum spp. Infections with D. phoxini were detected from July to November (prevalence range: 3.3–13.6%, see Online Resource Table S2 for details) with the largest number of infected snails and greatest prevalence being recorded in September (five samples). Although large samples were examined in May (12.v.–22.v.; n = 255) and June (7.vi.–25.vi.; n = 250), no snails infected with D. phoxini were found (see Online Resource Table S2).
All dissected P. phoxinus (n = 15) were infected with large numbers of metacercariae of D. phoxini (n > 100) located in the optical lobes of the brain.
Molecular identification of the snail host
Representative cox1 (591–610 nt; n = 2), 28S (1048 nt; n = 1) and ITS2 (402 nt; n = 1) sequences were generated for the snail host A. balthica. The new cox1 sequences differed at two nucleotide (nt) positions (0.03%). The new 28S rDNA sequence was identical with a sequence for A. balthica originally identified as R. ovata (EF417136; see Sonnenberg et al., Reference Sonnenberg, Nolte and Tautz2007) and differed at a single-nucleotide position from an isolate originating from Russia (MH168039; Aksenova et al., Reference Aksenova, Bolotov, Gofarov, Kondakov, Vinarski, Bespalaya, Kolosova, Palatov, Sokolova, Spitsyn, Tomilova, Travina and Vikhrev2018). ITS2 sequence comparisons revealed differences at 0–3 nt positions between the present isolate and the data for A. balthica available on GenBank; the new ITS2 sequence was identical with sequences for a total of 55 isolates of A. balthica originating from Belgium, Iceland, Norway and the UK.
Molecular identification of the snail host was further carried out on a cox1 alignment corresponding to the clade representing the subfamily Amphipepleinae Pini, 1877 in Aksenova et al. (Reference Aksenova, Bolotov, Gofarov, Kondakov, Vinarski, Bespalaya, Kolosova, Palatov, Sokolova, Spitsyn, Tomilova, Travina and Vikhrev2018) (36 species; 43 sequences; 636 nt) using Galba truncatula (O.F. Müller) (Lymnaeidae) as the outgroup. As shown in the NJ tree in Online Resource Fig. S1, the two newly generated cox1 sequences fell within the strongly supported clade of Ampullaceana spp. and clustered with five sequences for A. balthica originating from Europe and Asia with high support, thus confirming their identification based on morphology and the reclassification of Radix balthica to Ampullaceana (see Aksenova et al., Reference Aksenova, Bolotov, Gofarov, Kondakov, Vinarski, Bespalaya, Kolosova, Palatov, Sokolova, Spitsyn, Tomilova, Travina and Vikhrev2018).
Molecular characterization of Diplostomum phoxini
Partial cox1 sequences (349–407 nt) of D. phoxini were generated for a total of nine isolates (seven cercarial and two metacercarial), representing eight haplotypes (Table 1). Genetic divergence between seven cercarial and one metacercarial isolate and the two isolates of D. phoxini sequenced from Norway (see Soldánová et al., Reference Soldánová, Georgieva, Roháčová, Knudsen, Kuhn, Henriksen, Siwertsson, Shaw, Kuris, Amundsen, Scholz, Lafferty and Kostadinova2017) ranged between 0% and 1.2% (0–5 nt difference), whereas one newly sequenced metacercarial isolate (MZ615639) exhibited considerable divergence (2.2–3.1%, 9–12 nt difference) in the comparisons with the remaining isolates of D. phoxini from River Ruhr. A comparison with the recently published sequences for D. phoxini from P. phoxinus in Finland and Russia (Lebedeva et al., Reference Lebedeva, Chrisanfova, Ieshko, Guliaev, Yakovleva, Mendsaikhan and Semyenova2021) revealed an overall range for genetic divergence of 0–1.3%, excluding the most divergent haplotype sequenced from River Ruhr (MZ615639) and one most divergent haplotype sequenced from River Varzuga, Russia (MT982208: 3.5–4.8%; the upper limit represents the divergence between these two most divergent haplotypes). A total of 15 haplotypes were identified among the isolates sampled in Europe, including six novel haplotypes from the present material, six haplotypes from the material of Lebedeva et al. (Reference Lebedeva, Chrisanfova, Ieshko, Guliaev, Yakovleva, Mendsaikhan and Semyenova2021) and one haplotype (KY513185) reported from Norway by Soldánová et al. (Reference Soldánová, Georgieva, Roháčová, Knudsen, Kuhn, Henriksen, Siwertsson, Shaw, Kuris, Amundsen, Scholz, Lafferty and Kostadinova2017). One haplotype (MZ615634) was shared with an isolate ex P. phoxinus from Lake Ovre Heimdalsvatnet, Norway (KY513186; Soldánová et al., Reference Soldánová, Georgieva, Roháčová, Knudsen, Kuhn, Henriksen, Siwertsson, Shaw, Kuris, Amundsen, Scholz, Lafferty and Kostadinova2017) and an isolate ex P. phoxinus from River Uksa, Russia (MT982204; Lebedeva et al., Reference Lebedeva, Chrisanfova, Ieshko, Guliaev, Yakovleva, Mendsaikhan and Semyenova2021) and one haplotype was represented by two cercarial isolates from the River Ruhr (MZ615631 and MZ615632). Genetic divergence between D. phoxini and the species of the D. baeri complex ranged between 6.9% and 12.3% (Table 2).
Table 1. Summary data for isolates of Diplostomum phoxini and Ampullaceana balthica from the River Ruhr at Neheim (Germany) used for generation of the new cox1, ITS1-5.8S-ITS2 and 28S rDNA (domains D1–D3) sequences

Abbreviations: A, adult; C, cercaria; M, metacercaria.
a 28S.
b ITS1-5.8S-ITS2.
c ITS2 only.
Table 2. Percent interspecific genetic divergence (p-distance model) for D. phoxini compared with the species/lineages of the D. baeri species complex based on all cox1 sequences available on GenBank (retrieved on 29 June 2021)
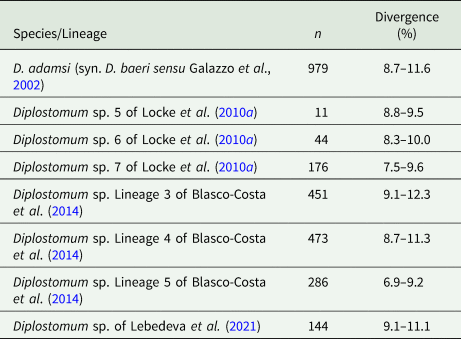
Abbreviation: n, number of pairwise comparisons
Additionally, two partial 28S (1214–1223 nt) and two complete ITS1-5.8S-ITS2 (1046–1049 nt) rDNA sequences were generated for representative cercarial and metacercarial isolates of D. phoxini (Table 1). The newly generated 28S sequences were identical and differed at six positions from the published sequence for D. phoxini by Olson et al. (Reference Olson, Cribb, Tkach, Bray and Littlewood2003).
Morphological characterisation of Diplostomum phoxini
Description of the cercaria
[Based on 40 live specimens; (Figs 1–3; Table 3 and Online Resource Table S3)] Body elongate-oval, 138–154 × 37–50, shorter than tail stem (TSL/BL = 1.34–1.62). Anterior organ elongate-oval, 52–59 × 27–31. Ventral sucker subspherical, with small undulating membrane (Figs 2 and 3), just post-equatorial, 30–34 × 30–33; width exceeding width of anterior organ (AOW/VSW = 0.84–0.94). Mouth opening ventro-subterminal; prepharynx long, narrower in anterior organ; pharynx round to elongate-oval, muscular, 11–14 × 12–16, followed by short oesophagus bifurcating anterior to ventral sucker; intestinal caeca well developed, terminating almost at posterior extremity of body. Penetration gland-cells two pairs with fine granular content, similar in size, posterior to ventral sucker, overlap caeca, posterior pair not reaching extremities of caeca; ducts open antero-laterally to mouth, two on either side. Anlagen of reproductive organs a compact mass of small cells just anterior to excretory vesicle. Tail stem 212–226 long, 29–37 wide at base, nearly as long as furcae, 212–239 long, 13–24 wide at base (TSL/FL = 0.95–1.06), with six pairs of caudal bodies with slightly irregular margins along excretory duct. Furcae 212–239 long, 13–24 wide at base, without fin-folds. Excretory vesicle small, V-shaped, with round stem; caudal excretory duct passes through tail stem; excretory pores at mid-length of furcae.

Fig. 1. Cercaria of Diplostomum phoxini ex Ampullaceana balthica (light microscopy). A, Resting position; B, Cercarial body.

Fig. 2. Cercaria of Diplostomum phoxini ex Ampullaceana balthica (scanning electron microscopy), ventral view. A, Entire cercaria; B, Cercarial body.

Fig. 3. Cercaria of Diplostomum phoxini ex Ampullaceana balthica (scanning electron microscopy), ventral view. A, Anterior organ, ventral view; B, Pre-oral spines, apical view; C, Transverse rows of tegumental spines on the body, ventral view; D, Ventral sucker with a well-developed undulating membrane, ventral view; E, Cercarial body, dorsal view; F, Anterior part of cercarial body, dorsal view at a higher magnification.
Table 3. Comparative data for the cercariae of Diplostomum phoxini and species of the D. baeri species complex

Body armature: Pre-oral spines arranged in a single median group of 10 spines in two rows, anterior row comprised of four spines; two central larger than the lateral; lateral groups of pre-oral spines lacking. Post-oral spines more robust than spines on body, in seven alternate rows encircling body to about mid-level of anterior organ; spines in first two rows much larger than remaining spines, all of similar size. Wide zone of smaller, less dense, irregularly dispersed spines present posterior to post-oral spines. Transverse rows of spines 8, extending to about mid-level of ventral sucker ventrally. Rows 1–5 complete ventrally; rows 6–8 incomplete ventrally; only row 1 complete dorsally. Two ventro-lateral non-confluent fields of smaller spines present in posterior body third. Ventral sucker armed with two rows of spines (c. 40 spines per row). Tail stem and furcae armed with minute spines; spines along tail stem in two ventral and two dorsal bands with two medio-lateral bands consisting of small, irregularly dispersed scale-like spines. Spines on furcae in one medial band laterally, consisting of 1–3 scale-like spines, size and density of spines decreasing distally.
Resting position: Tail stem straight, body slightly bent ventrally.
Description of the metacercaria
[Based on 20 live specimens from the optical lobes of the brain of Phoxinus phoxinus; Fig. 4; Online Resource Table S4] Body elongate-oval, 326–411 (358), with maximum width just anterior to ventral sucker, 145–227 (186). Oral sucker subterminal, subspherical, 38–56 × 36–50 (49 × 44). Pseudosuckers two, contractile, small-sized, 28–42 × 20–40 (34 × 30). Prepharynx indistinct; pharynx muscular, elongate-oval, 18–39 × 12–22 (31 × 18); oesophagus very short, bifurcates close posterior to pharynx; intestinal caeca narrow, encroach holdfast organ and terminate blindly at mid-level of excretory vesicle. Ventral sucker subspherical, 34–48 × 43–54 (43 × 47), similar in size to oral sucker or slightly larger [VSW/OSW = 1.0–1.1 (1.1)], at mid-body length or slightly posterior. Holdfast organ massive, 47–95 × 78–102 (71 × 91), bi-partite with median slit, transversely oval, contiguous with ventral sucker and excretory vesicle. Excretory vesicle large, conspicuous, V-shaped; reserve excretory system of diplostomid type; excretory concretions predominantly large, 345–579 (454) in number, grouped into two lateral and one median fields. Hindbody short, 24–53 (42).
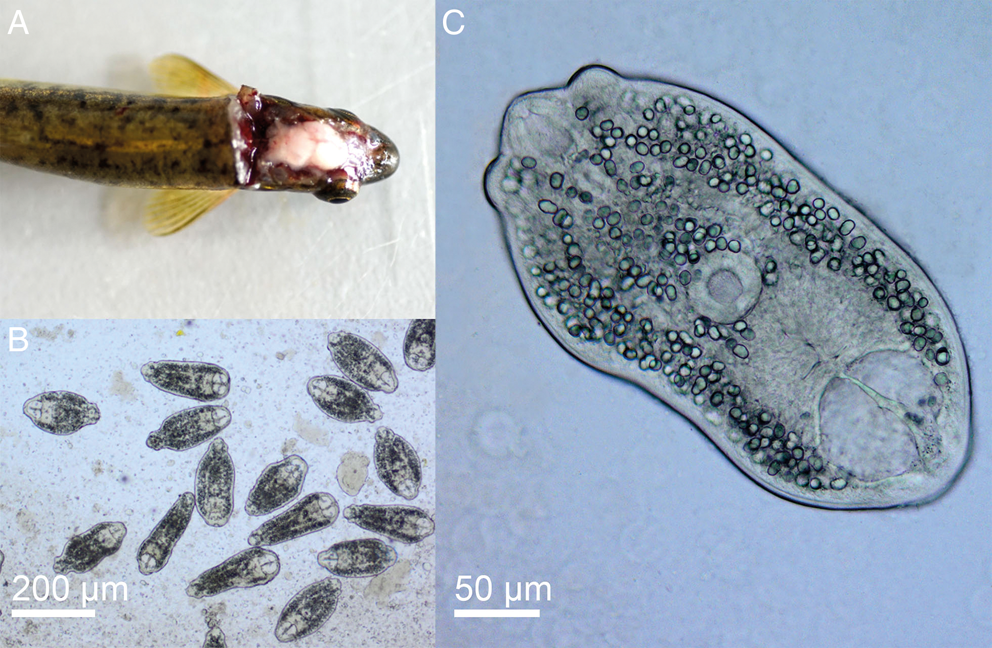
Fig. 4. Phoxinus phoxinus (A) and live metacercariae of Diplostomum phoxini (B, C).
Remarks
The present detailed descriptions expand the known range of variation of the metrical features and provide morphological detail that will facilitate the morphological identification of the larval stages of D. phoxini. The cercaria of D. phoxini resembles the known cercariae of the D. baeri species complex in the lack of the lateral group of pre-oral spines and the resting position with a straight tail stem but differs in the following species-specific features: (i) BL < TSL ⩾ FL; (ii) VSW > AOW; (iii) eight transverse rows of spines on the body; (iv) two rows of spines on the ventral sucker; (v) penetration gland-cells not covering ends of caeca (Table 3). Although the metrical data exhibit overlapping ranges for some features, the cercaria of D. phoxini can be distinguished from the cercaria of both D. baeri sensu Niewiadomska and Kiselienė (Reference Niewiadomska and Kiselienė1994) and Diplostomum sp. Lineage 4 of Blasco-Costa et al. (Reference Blasco-Costa, Faltýnková, Georgieva, Skírnisson, Scholz and Kostadinova2014) in having on average smaller body, shorter tail and furcae, a narrower apical organ; the tail in D. phoxini is also much longer than the body (Faltýnková et al., Reference Faltýnková, Georgieva, Kostadinova, Blasco-Costa, Scholz and Skírnisson2014; see Online Resource Table S3 for details).
The metacercaria of D. phoxini exhibits overlapping ranges for the metrical data with Diplostomum sp. Lineages 3–5 of Blasco-Costa et al. (Reference Blasco-Costa, Faltýnková, Georgieva, Skírnisson, Scholz and Kostadinova2014) but the means for the latter species-level lineages are greater (Online Resource Table S4; see also Faltýnková et al., Reference Faltýnková, Georgieva, Kostadinova, Blasco-Costa, Scholz and Skírnisson2014). Comparisons with the metacercariae measured by Lebedeva et al. (Reference Lebedeva, Chrisanfova, Ieshko, Guliaev, Yakovleva, Mendsaikhan and Semyenova2021) revealed an overall agreement for the metrical data except for the somewhat smaller body dimensions and the greater number of excretory concretions (Online Resource Table S4). Both differences are due to the fact that Lebedeva et al. (Reference Lebedeva, Chrisanfova, Ieshko, Guliaev, Yakovleva, Mendsaikhan and Semyenova2021) examined fixed material; this may have led to misinterpretations of excretory concretions.
Parasitism in a specific second intermediate host (P. phoxinus) can be also used to distinguish D. phoxini from Diplostomum sp. Lineage 4 of Blasco-Costa et al. (Reference Blasco-Costa, Faltýnková, Georgieva, Skírnisson, Scholz and Kostadinova2014), the only species of the D. baeri complex with a European distribution which was also recorded in the brain of Gasterosteus aculeatus.
Phylogenetic analyses
The newly generated cox1 sequences were analysed together with all published sequences for Diplostomum spp. (1203 sequences; 407 nt). The neighbour-joining analysis depicted 44 species/species-level lineages of Diplostomum with typically maximum or very high support (see Table 4 and Online Resource Fig. S2); these included seven taxa represented by singletons: Diplostomum sp. 5 and Diplostomum sp. 8 of Locke et al. (Reference Locke, McLaughlin, Dayanandan and Marcogliese2010a); Diplostomum sp. 11 of Locke et al. (Reference Locke, Al-Nasiri, Caffara, Drago, Kalbe, Lapierre, McLaughlin, Nie, Overstreet, Souza, Takemoto and Marcogliese2015); Diplostomum sp. A of Gordy and Hanington (Reference Gordy and Hanington2019), Diplostomum spp. A, B and C of Kudlai et al. (Reference Kudlai, Oros, Kostadinova and Georgieva2017).
Table 4. Species and species-level lineages of Diplostomum with a re-identification of some isolates (GenBank data as of 29 June 2021; see the neighbour-joining tree based on all available sequences in Online Resource Fig. S2 and Online Resource Table S5 for details)
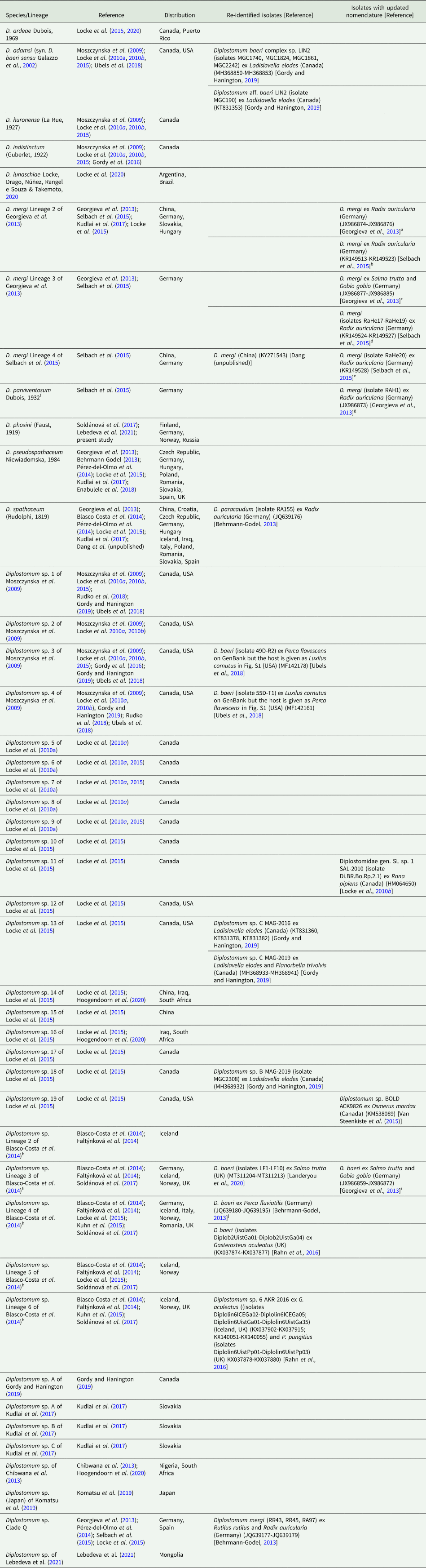
a D. mergi Lineage 2 (“D. mergi 2” and “Clade 3-2”) in Georgieva et al. (Reference Georgieva, Soldánová, Pérez-del-Olmo, Dangel, Sitko, Sures and Kostadinova2013)
b D. mergi Lineage 2 in Selbach et al. (Reference Selbach, Soldánová, Georgieva, Kostadinova and Sures2015) and Kudlai et al. (Reference Kudlai, Oros, Kostadinova and Georgieva2017)
c D. mergi Lineage 3 (“D. mergi 3” and “Clade 3-3”) in Georgieva et al. (Reference Georgieva, Soldánová, Pérez-del-Olmo, Dangel, Sitko, Sures and Kostadinova2013)
d D. mergi Lineage 3 in Selbach et al. (Reference Selbach, Soldánová, Georgieva, Kostadinova and Sures2015)
e D. mergi Lineage 4 in Selbach et al. (Reference Selbach, Soldánová, Georgieva, Kostadinova and Sures2015)
f Member of the D. mergi species complex, indicated as D. mergi Lineage 1 in Georgieva et al. (Reference Georgieva, Soldánová, Pérez-del-Olmo, Dangel, Sitko, Sures and Kostadinova2013)
g D. mergi Lineage 1 (“D. mergi 1” and “Clade 3-1”) in Georgieva et al. (Reference Georgieva, Soldánová, Pérez-del-Olmo, Dangel, Sitko, Sures and Kostadinova2013)
h Lineages discovered in Iceland and characterised molecularly and morphologically by Blasco-Costa et al. (Reference Blasco-Costa, Faltýnková, Georgieva, Skírnisson, Scholz and Kostadinova2014) and Faltýnková et al. (Reference Faltýnková, Georgieva, Kostadinova, Blasco-Costa, Scholz and Skírnisson2014), respectively
i D. baeri Lineage 1 (“D. baeri 1”, “Clade 4-1” or “trout lineage”) in Georgieva et al. (Reference Georgieva, Soldánová, Pérez-del-Olmo, Dangel, Sitko, Sures and Kostadinova2013)
j D. baeri Lineage 2 (“D. baeri 2”, “Clade 4-2” or “perch lineage”) in Georgieva et al. (Reference Georgieva, Soldánová, Pérez-del-Olmo, Dangel, Sitko, Sures and Kostadinova2013)
The sequences for D. phoxini clustered in a strongly supported reciprocally monophyletic lineage associated with three lineages of the D. baeri species complex sensu Blasco-Costa et al. (Reference Blasco-Costa, Faltýnková, Georgieva, Skírnisson, Scholz and Kostadinova2014): Diplostomum spp. 5 and 6 of Locke et al. (Reference Locke, McLaughlin, Dayanandan and Marcogliese2010a), and Diplostomum sp. Lineage 5 of Blasco-Costa et al. (Reference Blasco-Costa, Faltýnková, Georgieva, Skírnisson, Scholz and Kostadinova2014). The sequences for the second lineage of Diplostomum sp. from the brain of P. phoxinus discovered in Mongolia by Lebedeva et al. (Reference Lebedeva, Chrisanfova, Ieshko, Guliaev, Yakovleva, Mendsaikhan and Semyenova2021) also formed a strongly supported monophyletic clade (Online Resource Fig. S2).
Bayesian inference phylogenetic reconstruction for representatives of the genus Diplostomum (currently comprising 44 species/species-level lineages, see Table 4) depicted a composition of the D. baeri species complex similar to that in Blasco-Costa et al. (Reference Blasco-Costa, Faltýnková, Georgieva, Skírnisson, Scholz and Kostadinova2014) (Fig. 5). The only differences are the addition of D. phoxini and Diplostomum sp. of Lebedeva et al. (Reference Lebedeva, Chrisanfova, Ieshko, Guliaev, Yakovleva, Mendsaikhan and Semyenova2021) and the exclusion of Diplostomum sp. 2 of Moszczynska et al. (Reference Moszczynska, Locke, McLaughlin, Marcogliese and Crease2009). There was support for a close association with the D. baeri complex for 5 lineages: Diplostomum spp. 2, 12, 18 and 19 of Locke et al. (Reference Locke, Al-Nasiri, Caffara, Drago, Kalbe, Lapierre, McLaughlin, Nie, Overstreet, Souza, Takemoto and Marcogliese2015) forming a cluster, albeit with poor support, plus the singleton ex Rana pipiens (Diplostomum sp. 11). Within the D. baeri species complex, there was a strongly supported sister-group relationship between: (i) Diplostomum sp. Lineage 5 of Blasco-Costa et al. (Reference Blasco-Costa, Faltýnková, Georgieva, Skírnisson, Scholz and Kostadinova2014) and Diplostomum sp. 6 of Locke et al. (Reference Locke, McLaughlin, Dayanandan and Marcogliese2010a); and (ii) D. baeri sensu Galazzo et al. (Reference Galazzo, Dayanandan, Marcogliese and McLaughlin2002) and Diplostomum sp. 5 of Locke et al. (Reference Locke, McLaughlin, Dayanandan and Marcogliese2010a).
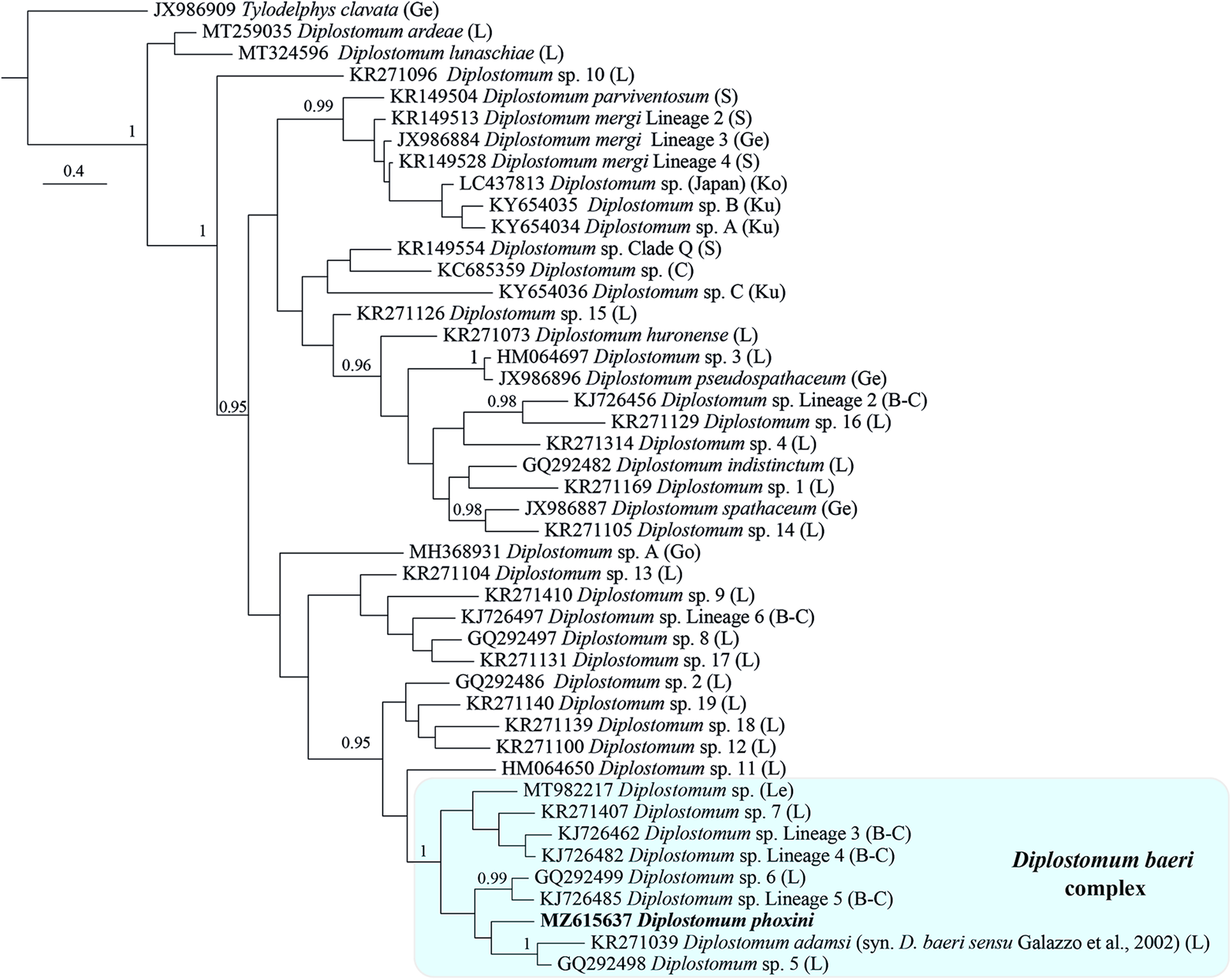
Fig. 5. Phylogram from Bayesian inference (BI) analysis of the cox1 sequence alignment (407 nt) for 44 species/species-level lineages of Diplostomum. Outgroup: Tylodelphys clavata. Nodal support is given as posterior probabilities; only values ⩾ 0.95 are shown. The scale-bar indicates the expected number of substitutions per site. The shaded rectangle indicates the content of the Diplostomum baeri species complex inferred from the present study. Abbreviations: B-C, Blasco-Costa et al. (Reference Blasco-Costa, Faltýnková, Georgieva, Skírnisson, Scholz and Kostadinova2014); C, Chibwana et al. (Reference Chibwana, Blasco-Costa, Georgieva, Hosea, Nkwengulila, Scholz and Kostadinova2013); Ge, Georgieva et al. (Reference Georgieva, Soldánová, Pérez-del-Olmo, Dangel, Sitko, Sures and Kostadinova2013); Go, Gordy and Hanington (Reference Gordy and Hanington2019); L, Locke et al. (Reference Locke, McLaughlin, Dayanandan and Marcogliese2010a, Reference Locke, McLaughlin and Marcogliese2010b, Reference Locke, Al-Nasiri, Caffara, Drago, Kalbe, Lapierre, McLaughlin, Nie, Overstreet, Souza, Takemoto and Marcogliese2015, Reference Locke, Drago, Núñez, Souza and Takemoto2020); Le, Lebedeva et al. (Reference Lebedeva, Chrisanfova, Ieshko, Guliaev, Yakovleva, Mendsaikhan and Semyenova2021); Ko, Komatsu et al. (Reference Komatsu, Itoh and Ogawa2019); Ku, Kudlai et al. (Reference Kudlai, Oros, Kostadinova and Georgieva2017); S, Selbach et al. (Reference Selbach, Soldánová, Georgieva, Kostadinova and Sures2015).
The present phylogenetic hypothesis for Diplostomum spp. depicted two additional well supported clusters: a group of 10 lens-infecting species/lineages containing the type-species of the genus, D. spathaceum; and the species/lineages of the D. mergi species complex sensu Selbach et al. (Reference Selbach, Soldánová, Georgieva, Kostadinova and Sures2015) which also included one additional lineage from Japan (see Komatsu et al., Reference Komatsu, Itoh and Ogawa2019) and two singletons from River Danube in Slovakia (see Kudlai et al., Reference Kudlai, Oros, Kostadinova and Georgieva2017) (Fig. 5). The relationships of the remaining species/lineages remained unresolved.
By means of raw pairwise interspecific divergence, the species/lineages of the D. baeri species complex appear well differentiated except for the two loose groups indicated by ellipses in the NMDS plot (Fig. 6) comprising D. phoxini and Diplostomum sp. Lineage 5 of Blasco-Costa et al. (Reference Blasco-Costa, Faltýnková, Georgieva, Skírnisson, Scholz and Kostadinova2014) + Diplostomum sp. 6 of Locke et al. (Reference Locke, McLaughlin, Dayanandan and Marcogliese2010a) (range for the latter two lineages: 3.5–5.5%) (see ranges for D. phoxini in Table 2). Importantly, comparisons of the isolates of D. baeri sensu Galazzo et al. (Reference Galazzo, Dayanandan, Marcogliese and McLaughlin2002) sampled in North America with the species/lineages of the D. baeri species complex revealed levels of genetic divergence (7.7–15.3%, see Table 5 for details) within the range reported for distinct species/lineages of Diplostomum (4.2–16.4%, see Georgieva et al., Reference Georgieva, Soldánová, Pérez-del-Olmo, Dangel, Sitko, Sures and Kostadinova2013; 4.3–14.7%, see Selbach et al., Reference Selbach, Soldánová, Georgieva, Kostadinova and Sures2015). The intraspecific divergence for these isolates was low (0–1.3%; based on 3872 pairwise comparisons).

Fig. 6 Non-metric multidimensional scaling ordination plot derived from the raw pairwise distances (p-distance) calculated for the species/lineages of the D. baeri complex based on the cox1 dataset.
Table 5. Percent interspecific genetic divergence (p-distance model) for D. adamsi (syn. D. baeri sensu Galazzo et al., Reference Galazzo, Dayanandan, Marcogliese and McLaughlin2002) sampled in North America compared with the species/lineages of the D. baeri species complex based on all cox1 sequences available on GenBank (retrieved on 29 June 2021)

Abbreviation: n, number of pairwise comparisons
Re-classification and an updated nomenclature for Diplostomum spp.
The present phylogenetic analyses helped update the nomenclature of a total of 144 sequenced isolates of Diplostomum spp. (see Table 4 for a summary and Online Resource Table S5 for a detailed list of all isolates; these are also indicated in the tree in Online Resource Fig. S2). Of these, we updated the species/lineage definitions with links to the GenBank accession numbers for 46 isolates (indicated in blue in Online Resource Fig. S2 and Table S5) and re-classified 98 isolates (indicated in red in Online Resource Fig. S2 and Table S5) assigned to 11 lineages as follows:
(i) D. adamsi (syn. D. baeri sensu Galazzo et al., Reference Galazzo, Dayanandan, Marcogliese and McLaughlin2002): reported as “Diplostomum baeri LIN2” by Gordy and Hanington (Reference Gordy and Hanington2019) and annotated on GenBank as “Diplostomum baeri complex sp. LIN2”and “Diplostomum aff. baeri LIN2”;
(ii) D. mergi Lineage 4 of Selbach et al. (Reference Selbach, Soldánová, Georgieva, Kostadinova and Sures2015): annotated as D. mergi on GenBank by Dang (unpublished sequence); this lineage is also not correctly annotated by Selbach et al. (Reference Selbach, Soldánová, Georgieva, Kostadinova and Sures2015);
(iii) D. spathaceum: reported and annotated on GenBank as D. paracaudum by Behrmann-Godel (Reference Behrmann-Godel2013); this isolate has been re-classified by Georgieva et al. (Reference Georgieva, Soldánová, Pérez-del-Olmo, Dangel, Sitko, Sures and Kostadinova2013);
(iv) Diplostomum sp. 3 of Moszczynska et al. (Reference Moszczynska, Locke, McLaughlin, Marcogliese and Crease2009): reported and annotated on GenBank as D. baeri by Ubels et al. (Reference Ubels, DeJong, Hoolsema, Wurzberger, Nguyen, Blankespoor and Blankespoor2018);
(v) Diplostomum sp. 4 of Moszczynska et al. (Reference Moszczynska, Locke, McLaughlin, Marcogliese and Crease2009): reported and annotated on GenBank as D. baeri by Ubels et al. (Reference Ubels, DeJong, Hoolsema, Wurzberger, Nguyen, Blankespoor and Blankespoor2018);
(vi) Diplostomum sp. 13 of Locke et al. (Reference Locke, Al-Nasiri, Caffara, Drago, Kalbe, Lapierre, McLaughlin, Nie, Overstreet, Souza, Takemoto and Marcogliese2015): reported and annotated on GenBank as “Diplostomum sp. C” by Gordy and Hanington (Reference Gordy and Hanington2019);
(vii) Diplostomum sp. 18 of Locke et al. (Reference Locke, Al-Nasiri, Caffara, Drago, Kalbe, Lapierre, McLaughlin, Nie, Overstreet, Souza, Takemoto and Marcogliese2015): reported and annotated on GenBank as “Diplostomum sp. B” by Gordy and Hanington (Reference Gordy and Hanington2019);
(viii) Diplostomum sp. Lineage 3 of Blasco-Costa et al. (Reference Blasco-Costa, Faltýnková, Georgieva, Skírnisson, Scholz and Kostadinova2014): reported as D. baeri by Landeryou et al. (Reference Landeryou, Kett, Ropiquet, Wildeboer and Lawton2020);
(ix) Diplostomum sp. Lineage 4 of Blasco-Costa et al. (Reference Blasco-Costa, Faltýnková, Georgieva, Skírnisson, Scholz and Kostadinova2014): reported and annotated on GenBank as D. baeri by Behrmann-Godel (Reference Behrmann-Godel2013) (assigned to the “perch” lineage or “D. baeri ” by Georgieva et al., Reference Georgieva, Soldánová, Pérez-del-Olmo, Dangel, Sitko, Sures and Kostadinova2013); reported as “D. baeri 2” and annotated on GenBank as D. baeri by Rahn et al. (Reference Rahn, Krassmann, Tsobanidis, MacColl and Bakker2016);
(x) Diplostomum sp. Lineage 6 of Blasco-Costa et al. (Reference Blasco-Costa, Faltýnková, Georgieva, Skírnisson, Scholz and Kostadinova2014): reported as “D. Lineage 6” but annotated on GenBank as Diplostomum sp. 6 by Rahn et al. (Reference Rahn, Krassmann, Tsobanidis, MacColl and Bakker2016); and
(xi) Diplostomum sp. Clade Q: reported and annotated on GenBank as D. mergi by Behrmann-Godel (Reference Behrmann-Godel2013); these isolates have been re-classified by Georgieva et al. (Reference Georgieva, Soldánová, Pérez-del-Olmo, Dangel, Sitko, Sures and Kostadinova2013).
Discussion
Diplostomum phoxini is a well-delimited species which differs from all described species of Diplostomum in the morphology of the adult stage, the strict host specificity to the second intermediate hosts (P. phoxinus) and the specific location in the fish brain. Nevertheless, in light of the expansive development of molecular studies on Diplostomum spp. and the current uncertainty in linking sequence data from larval stages to named species of this genus, it is desirable to describe the sequenced forms and thus anchor the molecular data to morphological reference (see e.g. Blasco-Costa et al., Reference Blasco-Costa, Faltýnková, Georgieva, Skírnisson, Scholz and Kostadinova2014; Faltýnková et al., Reference Faltýnková, Georgieva, Kostadinova, Blasco-Costa, Scholz and Skírnisson2014; Pérez-del-Olmo et al., Reference Pérez-del-Olmo, Georgieva, Pula and Kostadinova2014; Selbach et al., Reference Selbach, Soldánová, Georgieva, Kostadinova and Sures2015). Our study thus anchors the molecular data to detailed descriptions of the larval stages and provides molecular evidence for the identification of the first intermediate host, A. balthica. Furthermore, the phylogenetic analyses resulted in: (i) a re-identification/re-classification of 98 isolates including D. baeri sensu Galazzo et al. (Reference Galazzo, Dayanandan, Marcogliese and McLaughlin2002); (ii) re-definition of the composition of the D. baeri species complex which now includes nine molecularly characterised species/lineages, i.e. D. adamsi (syn. D. baeri sensu Galazzo et al., Reference Galazzo, Dayanandan, Marcogliese and McLaughlin2002), D. phoxini, Diplostomum sp. Lineages 3–5 of Blasco-Costa et al. (Reference Blasco-Costa, Faltýnková, Georgieva, Skírnisson, Scholz and Kostadinova2014), Diplostomum spp. 5–7 of Locke et al. (Reference Locke, Al-Nasiri, Caffara, Drago, Kalbe, Lapierre, McLaughlin, Nie, Overstreet, Souza, Takemoto and Marcogliese2015), and Diplostomum sp. of Lebedeva et al. (Reference Lebedeva, Chrisanfova, Ieshko, Guliaev, Yakovleva, Mendsaikhan and Semyenova2021); (iii) re-definition of the composition of the D. mergi species complex which now includes seven molecularly characterised species/lineages, i.e. D. parviventosum, D. mergi Lineages 2 and 3 of Georgieva et al. (Reference Georgieva, Soldánová, Pérez-del-Olmo, Dangel, Sitko, Sures and Kostadinova2013), Diplostomum mergi Lineage 4 of Selbach et al. (Reference Selbach, Soldánová, Georgieva, Kostadinova and Sures2015), Diplostomum spp. A and B of Kudlai et al. (Reference Kudlai, Oros, Kostadinova and Georgieva2017), and Diplostomum sp. of Komatsu et al. (Reference Komatsu, Itoh and Ogawa2019); and (iv) an updated nomenclature for the molecularly characterised species-level lineages of Diplostomum.
Prevalence and life-cycle of Diplostomum phoxini
The summarised data for the prevalence of the molecularly characterised species/lineages of Diplostomum in the snail intermediate hosts in Europe (Table 6) indicate that prevalence is generally low when estimated from pooled samples (0.7–4.7%), whereas the prevalence estimated from distinct individual samples (i.e. as per the definition of Bush et al., Reference Bush, Lafferty, Lotz and Shostak1997) ranges between 1.0 and 13.6% but is typically greater than 3.0%. The prevalence of D. phoxini in A. balthica studied in the River Ruhr fell within the latter range but with values greater than 3.0% in all distinct samples and a maximum prevalence of 13.6% recorded to date for Diplostomum spp. in Europe (Table 6; see also Online Resource Table S2 for details).
Table 6. Comparative data for the prevalence of D. phoxini and molecularly characterised species/lineages of Diplostomum spp. in intermediate snail hosts examined in Europe

a Overall prevalence for pooled samples taken during 1959–1965.
b Data from pooled samples.
c Water reservoirs of the River Ruhr catchment area in North Rhine-Westphalia.
d Pooled data for all lineages of the D. mergi species complex.
Although most of the populations of Diplostomum spp. originate from lentic aquatic habitats (lakes, reservoirs, ponds) it is worth noting that both studies with prevalence estimated for multiple distinct samples originating from lotic waterbodies (River Ruhr in Germany, present study; River Veude in France, see Arvy and Buttner, Reference Arvy and Buttner1954) revealed a high prevalence range of D. phoxini in both A. balthica and R. auricularia. This is in contrast with the expectation that flow conditions in the aquatic habitat affect digenean dispersal (Radke et al., Reference Radke, Ritchie and Rowan1961) with lentic habitats guaranteeing accelerated transmission rates (Soldánová and Kostadinova, Reference Soldánová and Kostadinova2011).
The data from our longitudinal study of the prevalence in A. balthica indicate that significant and consistent foci of infection with D. phoxini exist in the River Ruhr. This is further strengthened by the narrow transmission window estimated for D. phoxini in the riverine habitats studied. In Germany, the life span of A. balthica is estimated as one year, with copulation and egg-laying occurring in March (Glöer, Reference Glöer2002). We assume that in the River Ruhr juveniles hatch in April and the first patent infections in the new generation develop during June. This is supported by the fact that although large samples of snails were examined at all sites in May and June, the first patent infections with D. phoxini were registered as early as July. Our data thus indicate a transmission window of six months (June to November) with infection restart in each new snail generation.
Metacercariae of D. phoxini can survive in a minnow brain for up to five years and are accumulated by their fish hosts (Dönges, Reference Dönges1969b). This explains the maximum prevalence of 100% and high abundance of D. phoxini recorded in P. phoxinus host as reported previously (e.g. Arvy and Buttner, Reference Arvy and Buttner1954; Rees, Reference Rees1955, Reference Rees1957). The longevity and accumulation of metacercariae in fish counteract the narrow transmission window for the larval stages and ensure the existence of a reservoir for maintenance of the infection with this species in the River Ruhr. However, the definitive host of D. phoxini is still poorly known since the only natural infection has been reported in Mergus merganser L. (the host of D. pelmatoides (Dubois, 1932), a synonym of D. phoxini). The distribution of this bird species in Germany is generally confined to the wintering areas around the coasts of the Baltic Sea (between October−November and March−April) and there is an isolated declining breeding population in Bavaria (Keller, Reference Keller2009).
Recent observations, however, suggest an increase in the number of breeding pairs of M. merganser along the River Ruhr, that might contribute to the infection foci in A. balthica along the river.
Shigin (Reference Shigin1986, Reference Shigin1993) reported the existence of intense and persistent foci of infection with D. phoxini in fish populations of waterbodies where Mergus spp. are practically lacking. Studies on the life-cycle indicate that D. phoxini is not highly specific to its definitive host as adult flukes containing eggs and mature sperm have been obtained from both avian and mammalian hosts. Adults of D. phoxini have been raised experimentally in ducklings of Anas platyrhynchos (see Arvy and Buttner, Reference Arvy and Buttner1954; Dönges, Reference Dönges1969a, Reference Dönges1969b; Erasmus, Reference Erasmus1969), Cairina moschata domestica (see Arvy and Buttner, Reference Arvy and Buttner1954), L. argentatus (see Berrie, Reference Berrie1960) and laboratory mice (Berrie, Reference Berrie1960; Shigin, Reference Shigin1986, Reference Shigin1993), but not in L. ridibundus (see Dönges, Reference Dönges1969a); a very rapid rate of development in ducklings (3–5 days post-infection) has been observed (Rees, Reference Rees1955; Berrie, Reference Berrie1960; Dönges, Reference Dönges1969a, Reference Dönges1969b; Erasmus, Reference Erasmus1969). This rapid rate of adult development and compatibility with anatid and mammalian hosts, in association with a possibility of parasite-induced changes in fish behaviour and mortality at high intensity of infection levels, tend to support the hypothesis that purely facultative ichthyophages such as aquatic rodents (Shigin, Reference Shigin1986, Reference Shigin1993) and/or A. platyrhynchos (see Miroshnichenko and Sten'ko, Reference Miroshnichenko and Sten'ko1983) may also act as definitive hosts of D. phoxini.
Diplostomum baeri species complex
Including in the phylogenetic analysis D. phoxini and the additional 26 species-level lineages molecularly characterised during 2015–2021 resulted in a change of the composition of the D. baeri species complex with the inclusion of D. phoxini and Diplostomum sp. of Lebedeva et al. (Reference Lebedeva, Chrisanfova, Ieshko, Guliaev, Yakovleva, Mendsaikhan and Semyenova2021) and the exclusion of Diplostomum sp. 2 of Moszczynska et al. (Reference Moszczynska, Locke, McLaughlin, Marcogliese and Crease2009) which was associated with three North American lineages (Diplostomum spp. 12, 18 and 19 of Locke et al., Reference Locke, Al-Nasiri, Caffara, Drago, Kalbe, Lapierre, McLaughlin, Nie, Overstreet, Souza, Takemoto and Marcogliese2015) sequenced recently by Locke et al. (Reference Locke, Al-Nasiri, Caffara, Drago, Kalbe, Lapierre, McLaughlin, Nie, Overstreet, Souza, Takemoto and Marcogliese2015).
Metacercariae of all species/lineages of the D. baeri species complex represent non-lens-dwelling forms recovered from the eye vitreous humour and retina and the brain of the fish hosts. The microhabitat within the fish outside the lens utilised by the metacercariae of Diplostomum spp. is an important species characteristic (Shigin, Reference Shigin1986) and defining the exact location of the metacercariae can facilitate identification/differentiation as illustrated by Blasco-Costa et al. (Reference Blasco-Costa, Faltýnková, Georgieva, Skírnisson, Scholz and Kostadinova2014) who sequenced and differentiated morphologically two species-level lineages (Diplostomum sp. Lineage 3 from the vitreous humour of the eye and Diplostomum sp. Lineage 5 from the eye retina) in the salmonids Salmo trutta L. and Salvelinus alpinus (L.) and two lineages (Diplostomum sp. Lineage 4 from the eye retina and brain and Diplostomum sp. Lineage 6 from the retina) in the gasterosteid Gasterosteus aculeatus L.
Unfortunately, Locke et al. (Reference Locke, McLaughlin, Dayanandan and Marcogliese2010a) made no distinction between the sub-retinal space, retina and vitreous humour of the eye “because metacercariae in these sites often detach in frozen material”. This applies to six species/lineages for which additional clarification of the metacercarial microhabitat in fish is required based on examination of unfrozen material: D. baeri sensu Galazzo et al. (Reference Galazzo, Dayanandan, Marcogliese and McLaughlin2002); Diplostomum sp. 2 of Moszczynska et al. (Reference Moszczynska, Locke, McLaughlin, Marcogliese and Crease2009); and Diplostomum spp. 5–9 of Locke et al. (Reference Locke, McLaughlin, Dayanandan and Marcogliese2010a). Locke et al. (Reference Locke, Al-Nasiri, Caffara, Drago, Kalbe, Lapierre, McLaughlin, Nie, Overstreet, Souza, Takemoto and Marcogliese2015) applied the division of “lens” vs “non-lens (eye)” for these species and for five additional lineages (Diplostomum spp. 12, 13, 17–19). Overall, there is conflicting information for the location of the metacercariae between the two large inventories of Locke et al. (Reference Locke, McLaughlin, Dayanandan and Marcogliese2010a) and Locke et al. (Reference Locke, Al-Nasiri, Caffara, Drago, Kalbe, Lapierre, McLaughlin, Nie, Overstreet, Souza, Takemoto and Marcogliese2015) and between the text and the supplementary data of Locke et al. (Reference Locke, Al-Nasiri, Caffara, Drago, Kalbe, Lapierre, McLaughlin, Nie, Overstreet, Souza, Takemoto and Marcogliese2015) for 12 isolates of one lineage (Diplostomum sp. 2 of Moszczynska et al. (Reference Moszczynska, Locke, McLaughlin, Marcogliese and Crease2009)) and nine isolates of six lineages, respectively (highlighted in red in Online Resource Table S5). Regarding the lineages of the D. baeri species complex, conflicting information for isolate microhabitats in the fish hosts has been provided for one isolate (KR271039) of D. baeri sensu Galazzo et al. (Reference Galazzo, Dayanandan, Marcogliese and McLaughlin2002) and five isolates of Diplostomum sp. 7 of Locke et al. (Reference Locke, McLaughlin, Dayanandan and Marcogliese2010a) (KR271398, KR271399, KR271402, KR271404, KR271407) (see Online Resource Table S5; Locke et al., Reference Locke, Al-Nasiri, Caffara, Drago, Kalbe, Lapierre, McLaughlin, Nie, Overstreet, Souza, Takemoto and Marcogliese2015).
What is D. baeri sensu Galazzo et al. (Reference Galazzo, Dayanandan, Marcogliese and McLaughlin2002)?
In the Guide to the Parasites of Fishes of Canada, Gibson (Reference Gibson1996) provided a key for the metacercariae of seven species of Diplostomum, including three non-lens-dwelling forms: D. scudderi (Olivier, 1941) Dubois, 1966 (syn. Diplostomulum baeri eucaliae Hoffman & Hundley, 1957) from the brain or retina of gasterosteids; D. baeri bucculentum Dubois & Rausch, 1948 from the retina or vitreous humour of the eye of salmonids; and D. adamsi Lester & Huizinga, (Reference Lester and Huizinga1977) from the retina of Perca flavescens (Mitchill).
Galazzo et al. (Reference Galazzo, Dayanandan, Marcogliese and McLaughlin2002) developed experimentally adults in Larus delawarensis fed metacercariae from the “vitreous humour” of P. flavescens collected in the St Lawrence River near Montreal, Canada. These authors found a substantial differentiation (3.8%, 23 nt positions) in the ITS1 rDNA region between the specimens sequenced in North America and Europe and concluded that the two forms are not conspecific. However, Galazzo et al. (Reference Galazzo, Dayanandan, Marcogliese and McLaughlin2002) used the name D. baeri for their experimentally developed adults. Locke et al. (Reference Locke, McLaughlin, Dayanandan and Marcogliese2010a) generated cox1 sequences “from archived DNA of three vouchered adult specimens” studied by Galazzo et al. (Reference Galazzo, Dayanandan, Marcogliese and McLaughlin2002) and from eight additional adult specimens and 64 metacercariae from the “vitreous humour” of P. flavescens collected in Canada. These authors also used the name D. baeri based on the sequence matching with the adults identified by Galazzo et al. (Reference Galazzo, Dayanandan, Marcogliese and McLaughlin2002).
All recent molecular phylogenies indicate that the North American lineage named as D. baeri by Galazzo et al. (Reference Galazzo, Dayanandan, Marcogliese and McLaughlin2002) and Locke et al. (Reference Locke, McLaughlin, Dayanandan and Marcogliese2010a, Reference Locke, McLaughlin and Marcogliese2010b, Reference Locke, Al-Nasiri, Caffara, Drago, Kalbe, Lapierre, McLaughlin, Nie, Overstreet, Souza, Takemoto and Marcogliese2015) and the species-level lineages of the D. baeri complex are genetically distinct (Georgieva et al., Reference Georgieva, Soldánová, Pérez-del-Olmo, Dangel, Sitko, Sures and Kostadinova2013; Blasco-Costa et al., Reference Blasco-Costa, Faltýnková, Georgieva, Skírnisson, Scholz and Kostadinova2014; Faltýnková et al., Reference Faltýnková, Georgieva, Kostadinova, Blasco-Costa, Scholz and Skírnisson2014; Selbach et al., Reference Selbach, Soldánová, Georgieva, Kostadinova and Sures2015; Soldánová et al., Reference Soldánová, Georgieva, Roháčová, Knudsen, Kuhn, Henriksen, Siwertsson, Shaw, Kuris, Amundsen, Scholz, Lafferty and Kostadinova2017) and the present analyses strongly support this (Figs 5, 6; Table 4; Online Resource Fig. S2). Therefore, there is no justification for perpetuating use of this name for the North American lineage from P. flavescens and Larus spp. Compared with the original description of D. baeri based on specimens from Europe, the experimentally obtained material measured and illustrated by Galazzo et al. (Reference Galazzo, Dayanandan, Marcogliese and McLaughlin2002) differs in having: a much larger body with a longer and narrower forebody and a substantially longer and narrower hindbody; an oral sucker much larger than pharynx (mean OSW/PHW = 1.5 vs oral sucker slightly larger than pharynx; OSW/PHW = 0.96–1.26) that is also equal to ventral sucker (mean VSW/OSW = 1.01 vs oral sucker slightly smaller than ventral sucker in D. baeri). Additionally, the anterior margins of the vitelline fields reach to the level of ventral sucker in the material described by Galazzo et al. (Reference Galazzo, Dayanandan, Marcogliese and McLaughlin2002) whereas they extend anteriorly to ventral sucker up to mid-distance between pharynx and ventral sucker in D. baeri (see Dubois, Reference Dubois1970).
Unfortunately, Galazzo et al. (Reference Galazzo, Dayanandan, Marcogliese and McLaughlin2002) did not compare their material with the description of D. adamsi, the only species with metacercariae known to develop in P. flavescens in Canada and North America in general (see Gibson, Reference Gibson1996; Zelmer and Arai, Reference Zelmer and Arai1998). The life-cycle of D. adamsi was completed experimentally by Lester and Huizinga (Reference Lester and Huizinga1977) using Lymnaea stagnalis (L.) and Ladislavella elodes (Say) as the first intermediate hosts, P. flavescens as the only susceptible host out of five fish species tested, and Larus argentatus Pontoppidan as the experimental definitive host. In addition to the detailed descriptions of the life-cycle stages of D. adamsi, these authors provided histological and scanning electron microscopy evidence for the microhabitat of the metacercariae in P. flavescens, i.e. “in the peripheral retina, in a cavity between the photoreceptor cells and the pigment epithelium”.
The solution for the confusion with the identification of D. baeri sensu Galazzo et al. (Reference Galazzo, Dayanandan, Marcogliese and McLaughlin2002) comes from the detailed histological study of Ubels et al. (Reference Ubels, DeJong, Hoolsema, Wurzberger, Nguyen, Blankespoor and Blankespoor2018) clearly showing that infection with D. baeri sensu Galazzo et al. (Reference Galazzo, Dayanandan, Marcogliese and McLaughlin2002) is confined to tissues associated with the eye retina (choroidal vasculature) of P. flavescens; these authors also generated sequence data for the metacercariae from the retinal tissues of P. flavescens. As shown in Online Resource Fig. S2, these sequences clustered with the sequences from the same fish host and Larus spp. in the studies of Galazzo et al. (Reference Galazzo, Dayanandan, Marcogliese and McLaughlin2002), Moszczynska et al. (Reference Moszczynska, Locke, McLaughlin, Marcogliese and Crease2009) and Locke et al. (Reference Locke, McLaughlin, Dayanandan and Marcogliese2010a, Reference Locke, McLaughlin and Marcogliese2010b, Reference Locke, Al-Nasiri, Caffara, Drago, Kalbe, Lapierre, McLaughlin, Nie, Overstreet, Souza, Takemoto and Marcogliese2015). All of the above considerations clearly suggest that the metacercariae originating from P. flavescens and sequenced by these authors and by Ubels et al. (Reference Ubels, DeJong, Hoolsema, Wurzberger, Nguyen, Blankespoor and Blankespoor2018) represent the retinal form D. adamsi. The reclassification of the sequences labelled as “Diplostomum baeri complex sp. LIN2” and “Diplostomum aff. baeri LIN2” by Gordy and Hanington (Reference Gordy and Hanington2019) (see above) provides molecular evidence that, in agreement with the original description of D. adamsi, the snail L. elodes acts as the first intermediate host of this species. The introduction of D. adamsi as the only plausible identification for the lineage D. baeri sensu Galazzo et al. (Reference Galazzo, Dayanandan, Marcogliese and McLaughlin2002) sequenced by Galazzo et al. (Reference Galazzo, Dayanandan, Marcogliese and McLaughlin2002), Locke et al. (Reference Locke, McLaughlin, Dayanandan and Marcogliese2010a, Reference Locke, Al-Nasiri, Caffara, Drago, Kalbe, Lapierre, McLaughlin, Nie, Overstreet, Souza, Takemoto and Marcogliese2015) and Gordy and Hanington (Reference Gordy and Hanington2019) does not require changing the name of the D. baeri species complex as there is a number of lineages within it awaiting taxonomic scrutiny.
Re-classification and an updated nomenclature for Diplostomum spp.
Based on the present phylogenetic analyses, an updated nomenclature was applied and a large number of isolates of Diplostomum spp. published before 29 June 2021 was re-identified/re-classified (Table 4, Fig. 5, Online Resource Table S5, Fig. S2). The present re-classification revealed new linkages between life-cycle stages for three species/lineages, i.e. D. adamsi, Diplostomum sp. 13 of Locke et al. (Reference Locke, Al-Nasiri, Caffara, Drago, Kalbe, Lapierre, McLaughlin, Nie, Overstreet, Souza, Takemoto and Marcogliese2015) and Diplostomum sp. 18 of Locke et al. (Reference Locke, Al-Nasiri, Caffara, Drago, Kalbe, Lapierre, McLaughlin, Nie, Overstreet, Souza, Takemoto and Marcogliese2015). Cercarial isolates of these forms were sequenced from L. elodes in Canada by Gordy and Hanington (Reference Gordy and Hanington2019) (see Table 4 and Online Resource Table S5).
Here, we would like to highlight two cases with relevance to the data for the D. baeri complex discussed above. Landeryou et al. (Reference Landeryou, Kett, Ropiquet, Wildeboer and Lawton2020) used cox1 and ITS sequences to identify the metacercariae from the vitreous humour of Salmo trutta collected in Scotland and used for characterisation of the mitochondrial genome of a species they believed to be D. baeri. However, these authors selected for their analysis cox1 sequences for just two lineages of the D. baeri species complex, i.e. D. baeri sensu Galazzo et al. (Reference Galazzo, Dayanandan, Marcogliese and McLaughlin2002) from North America and Diplostomum sp. Lineage 3 of Blasco-Costa et al. (Reference Blasco-Costa, Faltýnková, Georgieva, Skírnisson, Scholz and Kostadinova2014) (the “trout clade” of the D. baeri species complex sensu Georgieva et al., Reference Georgieva, Soldánová, Pérez-del-Olmo, Dangel, Sitko, Sures and Kostadinova2013). Although the cox1 sequences of Landeryou et al. (Reference Landeryou, Kett, Ropiquet, Wildeboer and Lawton2020) clearly fell within the clade of Diplostomum sp. Lineage 3 of Blasco-Costa et al. (Reference Blasco-Costa, Faltýnková, Georgieva, Skírnisson, Scholz and Kostadinova2014) (the “trout clade” of Georgieva et al., Reference Georgieva, Soldánová, Pérez-del-Olmo, Dangel, Sitko, Sures and Kostadinova2013), they named the species as “D. baeri”. Our analysis revealed that the material sequenced by Landeryou et al. (Reference Landeryou, Kett, Ropiquet, Wildeboer and Lawton2020) in fact belongs to and should be referred to as Diplostomum sp. Lineage 3 of Blasco-Costa et al. (Reference Blasco-Costa, Faltýnková, Georgieva, Skírnisson, Scholz and Kostadinova2014) (Table 4, Online Resource Table S5 and Fig. S2).
Ubels et al. (Reference Ubels, DeJong, Hoolsema, Wurzberger, Nguyen, Blankespoor and Blankespoor2018) reported as D. baeri two sequences from metacercariae ex P. flavescens and Luxilus cornutus (Mitchill) collected in Douglas Lake, Michigan, USA. However, there is a conflict with host annotation in their paper and the Supplementary Fig. S1 provided by these authors (see Table 4). Whichever the host, our analysis clearly showed that the sequence MF142178 belongs to Diplostomum sp. 3 of Moszczynska et al. (Reference Moszczynska, Locke, McLaughlin, Marcogliese and Crease2009) and the sequence MF142161 belongs to Diplostomum sp. 4 of Moszczynska et al. (Reference Moszczynska, Locke, McLaughlin, Marcogliese and Crease2009).
The nomenclature of the genetic lineages of Diplostomum is in a state of flux since scientific names for 35 species-level lineages of Diplostomum have not yet been suggested. Identification to the species level via linking the genetic and morphological data for these lineages will be a long process and some lineages will remain unidentified for indefinite time. Locke et al. (Reference Locke, Al-Nasiri, Caffara, Drago, Kalbe, Lapierre, McLaughlin, Nie, Overstreet, Souza, Takemoto and Marcogliese2015) highlighted the problems associated with name discrepancies in the publications vs GenBank annotations for the expanding number of molecularly delineated species-level lineages within the Diplostomidae. Whilst we agree with their criticisms, we should like to highlight that the publication should be the leading source for the identification and host/microhabitat data for the newly sequenced isolates and the precise linking to GenBank sequences (and their annotations) should be part of the publication. In an ideal world with a centralised system for registering the lineage number sequence, the numbering system would be effective (as suggested by Locke et al., Reference Locke, Al-Nasiri, Caffara, Drago, Kalbe, Lapierre, McLaughlin, Nie, Overstreet, Souza, Takemoto and Marcogliese2015) but this is not the case; the same applies to a lettering system, e.g. there are pairs of lineages currently labelled as A, B and C (see Kudlai et al., Reference Kudlai, Oros, Kostadinova and Georgieva2017; Gordy and Hanington, Reference Gordy and Hanington2019).
Lineage ‘names’ (labels) are not species binomens and thus no compliance with the International Code of Zoological Nomenclature is required. However, it would be wise to follow Code's principles of homonymy and priority of publication to ensure that the ‘name’ of each genetic lineage is unique and distinct, and that the oldest available ‘name’ is used for already characterised lineages. The uniqueness is ensured by consistently using the ‘name’ in association with the reference of the first publication, e.g. Diplostomum sp. 1 of Moszczynska et al. (Reference Moszczynska, Locke, McLaughlin, Marcogliese and Crease2009), Diplostomum sp. A of Gordy and Hanington (Reference Gordy and Hanington2019), Diplostomum sp. A of Kudlai et al. (Reference Kudlai, Oros, Kostadinova and Georgieva2017), Diplostomum sp. Lineage 2 of Blasco-Costa et al. (Reference Blasco-Costa, Faltýnková, Georgieva, Skírnisson, Scholz and Kostadinova2014) or simply Diplostomum sp. of Chibwana et al. (Reference Chibwana, Blasco-Costa, Georgieva, Hosea, Nkwengulila, Scholz and Kostadinova2013) (see Table 4 for all updated lineage labels).
The updated data on the nomenclature and distribution for molecularly characterised species/lineages of Diplostomum based on our global analysis provided in Table 4 indicate that, in spite of the accumulation of sequences from recent studies, current distribution of Diplostomum spp. is the result of uneven sampling effort and suggest our knowledge of the species and genetic diversity in this group is still rudimentary in Africa, Asia and South America. Thus, nearly half of the molecularly characterised species/lineages (21; 48%) have only been recorded in North America. Of these, 12 (57%) taxa, including four singletons, have only been recorded in Canada. Nearly a third of the species/lineages (12 taxa, 27%, including 3 singletons) have only been recorded in Europe and there are fewer molecular records from Asia (eight taxa, including three also found in Europe: D. spathaceum; D. mergi Lineage 2 of Georgieva et al. (Reference Georgieva, Soldánová, Pérez-del-Olmo, Dangel, Sitko, Sures and Kostadinova2013); D. mergi Lineage 4 of Selbach et al. (Reference Selbach, Soldánová, Georgieva, Kostadinova and Sures2015)) and Africa (three taxa, including two also found in Asia: Diplostomum spp. 14 and 16 of Locke et al. (Reference Locke, Al-Nasiri, Caffara, Drago, Kalbe, Lapierre, McLaughlin, Nie, Overstreet, Souza, Takemoto and Marcogliese2015)). Just one species has been characterised molecularly in South America.
Finally, the present updated synopsis of Diplostomum species/lineages highlights an important caveat for enhancing the knowledge of the diversity of Diplostomum spp. in fish hosts, i.e. the virtual lack of sequences for metacercariae of salmonid and gasterosteid hosts from North America. Currently, only four sequences are available from these host groups, three sequences from metacercariae in salmonids, two for Diplostomum sp. 7 and one for Diplostomum sp. 9, and a single sequence for Diplostomum sp. 13 of Locke et al. (Reference Locke, Al-Nasiri, Caffara, Drago, Kalbe, Lapierre, McLaughlin, Nie, Overstreet, Souza, Takemoto and Marcogliese2015) (possibly D. scudderi (Olivier, 1941)) from G. aculeatus. We predict that, similar to the current situation in Europe, focused sampling of gasterosteids and salmonids with a careful identification of the location of the non-lens-dwelling metacercariae will reveal a number of additional species/lineages of the D. baeri complex in North America. Furthermore, precise identification of the microhabitat in salmonid hosts anchored to novel morphological and sequence data may help assess the status of D. baeri bucculentum Dubois & Rausch, 1948 and distinguish it from the retinal form reported from salmonoids in Canada (see Gibson, Reference Gibson1996) and from the European lineages molecularly and morphologically characterised by Blasco-Costa et al. (Reference Blasco-Costa, Faltýnková, Georgieva, Skírnisson, Scholz and Kostadinova2014) and described by Faltýnková et al. (Reference Faltýnková, Georgieva, Kostadinova, Blasco-Costa, Scholz and Skírnisson2014). Sequencing of metacercariae from salmonids and gasterosteids will also provide additional data for testing the hypothesis for North America being an ancestral area for the D. baeri species complex (Blasco-Costa et al., Reference Blasco-Costa, Faltýnková, Georgieva, Skírnisson, Scholz and Kostadinova2014) and shed light on the evolution of this group.
Supplementary material
The supplementary material for this article can be found at https://doi.org/10.1017/S0031182021001372
Data
The data supporting the findings of this study are available within the article and its supplementary materials. All newly generated sequences were deposited in the GenBank database under the following accession numbers: MZ615631-MZ615639 (cox1, D. phoxini); MZ616379 and MZ616380 (28S, D. phoxini); MZ616381 and MZ616382 (ITS1-5.8S-ITS2, D. phoxini); MZ615629 and MZ615630 (cox1, A. balthica); MZ616383 (28S, A. balthica); and MZ616378 (ITS2, A. balthica). Raw data are available on request from the corresponding author [JS].
Acknowledgements
We are grateful to Julian Enß, Lisa Fuchs, Katrin Honka, Jenia Kremers, Lena Kusanke, Anne Ladwig, Diana Michler, Louisa Rothe, Till Stempel, Sylvester Watzlawczyk, Laura Witthaus and Tim Zimara for their assistance during sampling and in the laboratory; Drs Anna Faltýnková and Miroslava Soldánová for their help with preliminary species identification; Drs Armin Lorenz and Kathrin Januschke for their help with selection of sampling sites; Dr Bernd Stemmer for fishing and providing minnows and Jonas Heidebrecht for his kind help with the figures.
Author contributions
BS and AK conceived the study and supervised the project. JS and DG carried out the sampling, dissection of snails and fish and data analyses. SG carried out the sequencing and performed the phylogenetic analyses. JS and SG drafted the manuscript. BS and AK oversaw the analyses and writing, and reviewed the manuscript. All authors read and approved the final manuscript.
Financial support
JS received a research grant from the University of Duisburg-Essen. SG benefitted from a postdoctoral fellowship “Juan de la Cierva – Formación” of the MICINN (FJCI-2016-29535) Spain.
Conflict of interest
The authors declare that they have no conflicts of interest.
Ethical standards
All applicable institutional, national and international guidelines for the care and use of animals were followed. The necessary permit for collecting and euthanizing fish was obtained prior to sampling (No. 51.3.1-6.2 Bezirksregierung Arnsberg).