Introduction
Echinococcus granulosus sensu lato (Eg) is a cestode parasite with a life cycle involving two mammalian hosts. The adult worm develops in the small intestine of dogs and wild canids, shedding infective eggs through feces into the environment, whereas the larval form (metacestode), acquired through oral ingestion of eggs, persists mainly in the liver and lungs of several species of ungulates, in particular livestock intermediate hosts including sheep, cattle, swine and camel. Humans may act as accidental dead-end intermediate hosts, developing cystic echinococcosis (CE) (Casulli et al., Reference Casulli, Siles-Lucas and Tamarozzi2019).
CE is a complex disease and the clinical presentation depends on the location of the cyst within the body, the size and number of cysts, the interaction with surrounding structures and possibly the parasite genotype (Kern et al., Reference Kern, Menezes da Silva, Akhan, Müllhaupt, Vizcaychipi, Budke and Vuitton2017). The liver is affected in approximately 70% of cases, followed by the lungs (20%). Other organs can be affected such as the abdominal or pleural cavities, kidney, spleen, bone, heart, central nervous system and other sites (Hizem et al., Reference Hizem, M'rad, Oudni-M'rad, Mestiri, Hammedi, Mezhoud, Zakhama, Mokni and Babba2016). Rare immune-mediated reactions such as urticaria, asthma, membranous nephropathy and anaphylaxis may also occur (Agudelo et al., Reference Agudelo Higuita, Brunetti and McCloskey2016). Clinical signs, if they ever develop, may arise after a highly variable incubation period of several months to years following infection. CE represents a considerable public health problem in many countries of the world, especially in pastoral regions and rural settings (Possenti et al., Reference Possenti, Manzano-Román, Sánchez-Ovejero, Boufana, La Torre, Siles-Lucas and Casulli2016; Tamarozzi et al., Reference Tamarozzi, Akhan, Cretu, Vutova, Akinci, Chipeva, Ciftci, Constantin, Fabiani, Golemanov, Janta, Mihailescu, Muhtarov, Orsten, Petrutescu, Pezzotti, Popa, Popa, Popa, Velev, Siles-Lucas, Brunetti and Casulli2018; Casulli, Reference Casulli2020).
Among the management options for human CE (Brunetti et al., Reference Brunetti, Kern and Vuitton2010), drug treatment is currently based on the use of poorly soluble benzimidazoles (BMZ), i.e. albendazole (ABZ) or mebendazole (MBZ). To date, the only BMZ licensed for human use are Albendazole, Triclabendazole, Flubendazole, Mebendazole and Thiabendazole. Before the introduction of BMZs, surgery was the only option for the management of human CE. Since then, BMZs have clearly improved the prognosis for infected patients, and decreased the risk of secondary echinococcosis in patients undergoing surgery (Gollackner et al., Reference Gollackner, Langle, Auer, Maier, Mittlbock, Agstner, Karner, Langer, Aspock, Loidolt, Rockenschaub and Steininger2000; Arif et al., Reference Arif, Shams Ul, Wani, Zargar, Wani, Tabassum, Hussain, Baba and Lone2008). However, due to the lack of parasiticidal activity of these compounds (Siles-Lucas et al., Reference Siles-Lucas, Casulli, Cirilli and Carmena2018), and the requirement for long-term treatment, which often lasts for years or even lifelong, there is ample room for improvement in terms of drug efficacy. Alternative formulations of BMZ, nano-compounds of BMZ and different drugs have been tested mainly in vitro; however, new formulations and compounds have consistently shown largely similar or lower efficacy than ABZ and only a few candidates reached clinical use (Li et al., Reference Li, Song, Shao, Aili, Ahan and Wen2016; Siles-Lucas et al., Reference Siles-Lucas, Casulli, Cirilli and Carmena2018; Bakhtiar et al., Reference Bakhtiar, Akbarzadeh, Casulli, Mahami-Oskouei, Ahmadpour, Nami, Rostami and Spotin2019).
One drawback of the currently used ABZ, and its metabolite ricobendazole (RBZ and ABZ sulphoxide), is the poor intestinal absorption. Thus, the drugs do not reach the desired concentrations to be efficacious against CE or other parasitic diseases against which they are active. Therefore, high dosages and repeated and/or long-term treatments are needed, which expose patients to side-effects, and decrease their quality of life (Horton, Reference Horton1997).
CE is a neglected disease (Brunetti et al., Reference Brunetti, Garcia and Junghanss2011). In this context, no new drugs or few new formulations of already existing lead drugs for its treatment have been specifically patented in the last 40 years (Patentscope database, 2020). Within the framework of HERACLES European funded project (Casulli et al., Reference Casulli, Siles-Lucas, Cretu, Vutova, Akhan, Vural, Cortés Ruiz, Brunetti and Tamarozzi2020), new formulations of antiparasitic soluble drugs (‘Salts of compounds having a benzimidazolic structure’; PCT/IT2016/000191) have recently been patented for the European (EP16788256) and US (US20190016686A1) markets (Cirilli et al., Reference Cirilli, Carradori, Casulli and De Monte2020). Among the new BMZ formulations included in this patent, Cirilli and colleagues reported on novel salt formulations of ABZ (ABZ-Na), and of RBZ (RBZ-Na) as well as its enantiomers [(R)-(+)-RBZ-Na and (S)-(−)-RBZ-Na]. These formulations allow improved intestinal absorption. In fact, the poor solubility in water of RBZ (0.06 mg mL−1) has been increased at physiological pH to 14.49 mg mL−1 for RBZ-Na (Cirilli et al., Reference Cirilli, Guglielmi, Formica, Casulli and Carradori2017).
Here we provide a preliminary assessment of these newly described salt formulations with respect to their efficacy against E. granulosus metacestodes in terms of mean weight of parasite cyst reduction and the parasite damage they induce in a mouse model of secondary infection. We define secondary CE infection as newly formed hydatid cysts in the peritoneum, pleura or meningeal space, induced by the intraperitoneal injection of protoscoleces in such areas.
Materials and methods
Parasites
Hydatid cysts were collected from naturally infected sheep in slaughterhouses in Tekirdag (Turkey). Protoscoleces were aspirated from the cysts under aseptic conditions and washed four times with sterile phosphate-buffered saline (PBS) (pH 7.2) supplemented with gentamycin (50 μg mL−1; I.E. Ulagay Pharmaceutical Industry, Istanbul, Turkey). Viability of protoscoleces was determined by methylene blue exclusion test and flame cell activity (Casado et al., Reference Casado, Rodriguez-Caabeiro and Hernandez1986). Echinococcus granulosus genotype was determined by sequencing the mitochondrial cytochrome c oxidase subunit 1 (cox1) gene, as previously described (Vural et al., Reference Vural, Unsal Baca, Gauci, Bagci, Yunus and Lightowlers2008). Protoscoleces preparations used for infections showed more than 95% viability and were of parasite genotype G1 (Cucher et al., Reference Cucher, Mourglia-Ettlin, Prada, Costa, Kamenetzky, Poncini, Dematteis and Rosenzvit2013).
Benzimidazole preparation
Salts of the enantiomers of RBZ were prepared according to the procedure previously described (Cirilli et al., Reference Cirilli, Guglielmi, Formica, Casulli and Carradori2017). Synthesis of enantiopure and racemic forms of RBZ-Na was carried out by adding a weighed amount of sodium hydroxide (1.1 eq) (solubilized in ethanol) to the ethanol solution of the non-salified form (1.0 eq). The reaction mixture was stirred for 20 min at room temperature, filtered and the solvent evaporated under reduced pressure. The drugs assessed in this study were: (1) ABZ; (2) ABZ-Na (ABZ sodium salt); (3) RBZ-Na (RBZ sodium salt); (4) (R)-(+)-RBZ-Na enantiomer and (5) (S)-(−)-RBZ-Na enantiomer.
Experimental infection and drug treatment
Seventy-two female BALB/c mice, 10 weeks of age, were housed with free access to food and water. After 1 week of quarantine period, they were marked by individual ear tag numbers. Each mouse was infected by intraperitoneal injection of 1500 viable protoscoleces suspended in 500 μL sterile PBS. After infection and before treatment, only abdominal enlargement was observed due to the growth of the cyst. Nine months post-infection, the mice were randomly assigned to six distinct treatment groups of 12 mice each: (1) ABZ, (2) ABZ-Na, (3) RBZ-Na, (4) (R)-(+)-RBZ-Na, (5) (S)-(−)-RBZ-Na and (6) placebo control. Since ABZ and ABZ-Na are poorly water soluble, they were formulated in dimethyl sulphoxide (DMSO) similarly to other published studies (Siles-Lucas et al., Reference Siles-Lucas, Casulli, Cirilli and Carmena2018). Since RBZ-Na, (R)-(+)-RBZ-Na and (S)-(−)-RBZ-Na are highly soluble, they were formulated in PBS for human delivery. Drugs were administered at a concentration of 10 mg kg−1 body weight in a volume of 100 μL by oral gavage on a daily basis for a period of 30 days. The control group was daily dosed with a corresponding amount of DMSO. All formulations were prepared freshly every day.
Blood and tissue sampling
Blood and parasite cyst samples were collected on day 30 at different time points after the last drug application, namely 0, 15, 30 and 180 min after dosing. Three mice per time point and group were sampled, with the exception of group ABZ-Na (two mice sampled at 30 min), and groups RBZ-Na (two mice sampled at 15 min), and (S)-(−)-RBZ-Na (two mice sampled at 15 min), since three mice died at different days during daily check in the 9-month infection period, prior to the treatment. Autopsy was performed on these three animals and revealed that some cysts bursted and there was fluid in the abdominal cavity. Therefore, we think that the cause of death may be due anaphylaxis consequent to the rupture of cysts. We did not find any unusual findings other than cyst burst at autopsy.
Mice were anaesthetized and euthanasia was conducted by exsanguination by cardiac puncture. Blood was collected in EDTA-containing tubes and stored at 4°C for 8 h. Blood samples from each time point within groups were pooled and centrifuged for 10 min at 1300 g at 4°C. Plasma was separated in 500 μL aliquots and stored at −20°C until use. After death by exsanguination, mice were dissected by opening the peritoneal cavity, and the hydatid cysts were removed, separated from host tissue and the total cystic mass was weighed. From each mouse, cyst fluid samples were collected from five randomly selected cysts using a sterile syringe and samples were stored at −20°C until use. One large (diameter ranging from 10 to 25 mm) and one medium-sized (diameter ranging from 5 to 9 mm) cyst from six animals per group were randomly selected and processed for microscopical evaluation.
Histochemical evaluation of parasite cysts
Methanol-carnoy (methacarn) fixative (Puchtler et al., Reference Puchtler, Waldrop, Meloan, Terry and Conner1970) was injected into the cysts immediately following cyst fluid aspiration. After 1 h at room temperature, cysts were cut into two pieces and fixation resumed for another 4 h. Tissue samples were then sequentially dehydrated in absolute ethanol processed in methyl benzoate and benzole and then embedded in paraffin (Heym and Forssmann, Reference Heym and Forssmann1981).
Consecutive 5–6 μm thick sections were cut and placed onto glass slides, and were stained using trichrome staining for histological structures (Gray, Reference Gray1954), and PAS-Alcian blue-(PAS/AB) for neutral and acid mucopolysaccharides (Bancroft et al., Reference Bancroft, Stevens and Turner1990). Specimens were assessed microscopically using an Olympus BX51 microscope (Tokyo, Japan).
Scanning electron microscopy (SEM)
Following cyst fluid aspiration, cysts were injected with 4% glutaraldehyde in 0.2 m Sorensen's buffer at pH 7.2 (Murtey and Ramasamy, Reference Murtey, Ramasamy, Milos and Robert2016). After 1 h, cysts were cut in half and were further fixed for 4 h. For SEM examination, cyst samples were rinsed in Sorensen's buffer and were dehydrated in graded series of ethanol, and then air-dried. The cysts were placed onto SEM stubs with the germinal layer (GL) exposed upwards, and the samples were inspected without coating in a QUANTA 250 FEI (Oregon, USA) at 100 Pa according to the protocol recommended by Murtey and Ramasamy (Reference Murtey, Ramasamy, Milos and Robert2016).
HPLC analysis
Reagents and instruments
High performance liquid chromatography (HPLC) analyses were performed by using a stainless-steel Chiralpak IA (250 mm × 4.6 mm i.d., 3 μm) column (Daicel, Tokyo, Japan). HPLC-grade solvents were supplied by Sigma-Aldrich (Milan, Italy). The HPLC system consisted of a Dionex P580 LPG pump (Dionex, Sunnyvale, CA, USA), an ASI-100T autosampler, a STH 585 column oven and a PDA-100 UV detector. The data were acquired and processed by using a Chromeleon Datasystem (Dionex).
Preparation of the calibration curves
Solutions for calibration curves were prepared with pooled plasma samples from non-infected and non-treated mice (400 μL) spiked with known amounts of ABZ (5.140, 2.570, 1.028 and 0.206 μg), RBZ (5.340, 2.670, 1.068 and 0.214 μg) and albendazole sulphone (ABZ-SO2) (5.180, 2.590, 1.036 and 0.207 μg) in methanol (2.0 mL of standard solution) containing a fixed amount of fenbendazole (FBZ) (0.519 μg) used as an internal standard. The samples were centrifuged at 2000 g for 5 min. The supernatant was filtered through a 0.22 μm filter and applied to a 250 mm × 4.6 mm Chiralpak IA column. The mobile phase was acetonitrile–water 100:120, at a flow of 1.0 mL min−1 and the injection volume was 20 μL. Detection was done by UV absorption at 291 nm. Calibration curves are shown in Supplementary Fig. 1.
The calibration curves were constructed by plotting the peak area ratio for each compound with respect to the internal standard, against the amount of the compound added to each blank plasma sample.
The solutions followed Beer–Lambert's law over the concentration range investigated at the selected wavelength with the resulting regression equations: (ABZ) y = 0.0352x − 0.1436 (R 2 = 0.9998); [(R)-(+)-RBZ, first eluted enantiomer] y = 0.0437x − 0.2546 (R 2 = 0.9990); [(S)-(−)-RBZ, second eluted enantiomer] y = 0.0415x + 0.1669 (R 2 = 0.9995); (ABZ-SO2) y = 0.0502x − 0.0743 (R 2 = 0.9996). The limit of quantitation values for ABZ, (R)-(+)-RBZ, (S)-(−)-RBZ and ABZ-SO2 were 5.15, 4.00, 4.12 and 4.25 μg mL−1, respectively.
Preparation of samples
Plasma concentrations of ABZ, (R)-(+)-RBZ, (S)-(−)-RBZ and ABZ-SO2 were measured by HPLC. In total, 400 μL of plasma were mixed with 2 mL of methanol and 20 μL of internal standard (0.4 μg) and centrifuged at 2000 g for 5 min. The supernatant was filtered through 0.22 μm mesh and analysed by HPLC as described above.
Statistics
The mean weight of the whole cysts was compared between mice of each treated group and those of the control group by one-way analysis of variance (ANOVA) after having verified the assumptions of normality and homoscedasticity through the Shapiro–Wilk test and Bartlett's test, respectively. These differences were also presented in terms of efficacy of treatment calculated with the formula (μ C − μ T)/μ C × 100, where μ C is the mean weight of the whole cystic mass in the control group and μ T is the mean weight of the whole cystic mass in each treated group (Ceballos et al., Reference Ceballos, Virkel, Elissondo, Canton, Canevari, Murno, Denegri, Lanusse and Alvarez2013). ANOVA was also used to evaluate differences in mean weight of the whole cystic mass by treatment separately for the compounds dissolved in DMSO and the compounds dissolved in PBS, using the Bonferroni adjustment for multiple pairwise comparisons.
Results
Efficacy of salts of BMZ against E. granulosus metacestodes in the murine model
Following infection of mice with E. granulosus sensu stricto (G1 genotype) protoscoleces, the development of hydatid cysts proceeded over a time span of 9 months, followed by daily treatments by oral gavage during 30 days. Necropsies were performed 10 months after infection. Cysts were found in the abdominal cavity of all mice. The mean weights of the total cyst load in all treatment groups were significantly different from the control group, with the exception of the (S)-(−)-RBZ-Na treatment. The highest efficacy, expressed as a mean weight decrease of the whole echinococcal cyst mass, was obtained by treatment with ABZ-Na (69.0%), followed by (R)-(+)-RBZ-Na (56.2%), RBZ-Na (41.0%) and ABZ (36.4%). (S)-(−)-RBZ-Na was the least effective (13.3%) (Fig. 1 and Table 1). Of note, treatment with the salt of ABZ (ABZ-Na) resulted in significantly lower cystic weight compared to treatment with conventional ABZ formulation diluted in DMSO (P = 0.037). Overall, differences were also found among the hydro-soluble drugs (P = 0.001). In particular, mean cyst weights were significantly lower in the group treated with RBZ-Na compared to the (S)-(−)-RBZ-Na group (P = 0.039). In terms of cyst weights, (R)-(+)-RBZ-Na treatment was also significantly more effective compared to treatment with (S)-(−)-RBZ-Na (P = 0.001). No significant differences were found between groups treated with RBZ-Na and (R)-(+)-RBZ-Na.
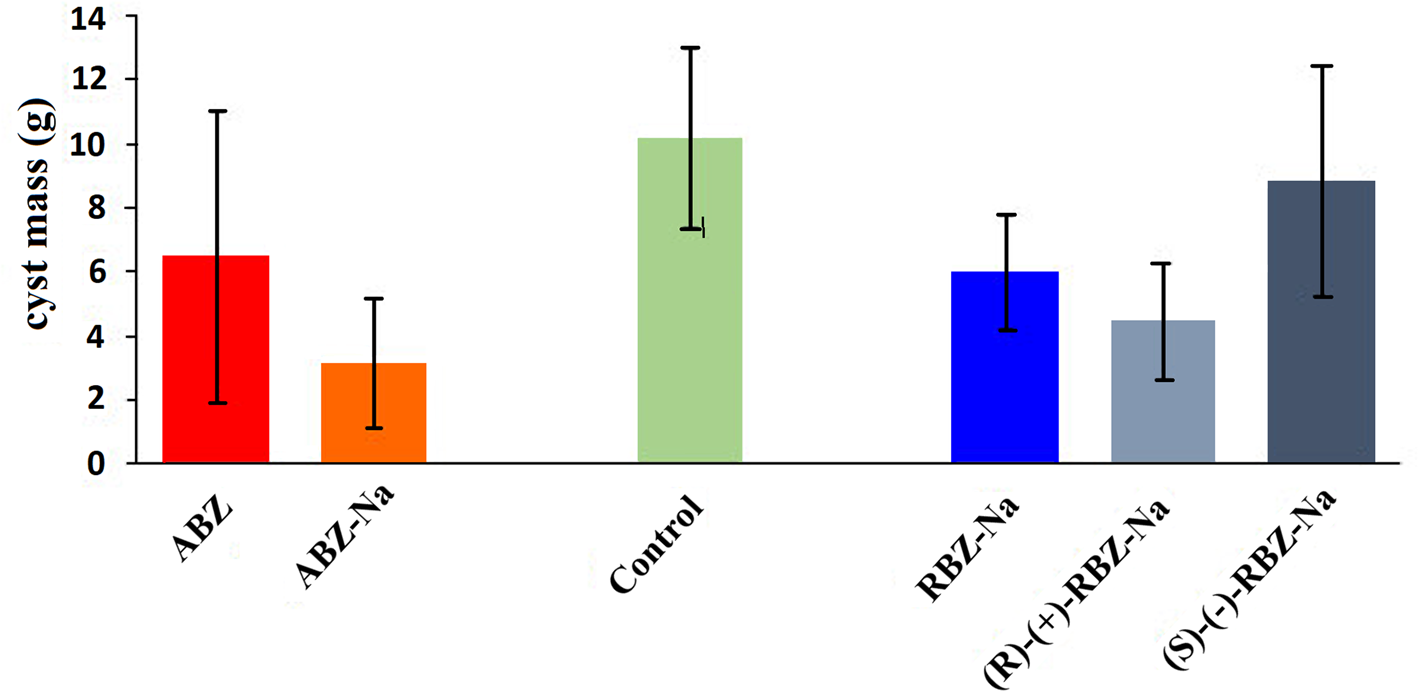
Fig. 1. Mean E. granulosus cyst weights per mouse (±s.d.) recovered from the different experimental groups after 30 days of treatments with various formulations of ABZ and RBZ.
Table 1. Overview of the experimental groups and outcome of treatments in terms of cyst weight and efficacy

s.d., standard deviation.
a One mouse in the groups ABZ-Na, RBZ-Na and S-(+)-RBZ-Na died before treatment was initiated.
*Statistically significant efficacy in reducing cyst weight assuming the placebo group as reference (P < 0.05 based on ANOVA).
Histopathology: salts of BMZ induced alterations in the GL and laminated layer (LL) of E. granulosus metacestodes
Trichrome staining (Fig. 2) showed that the GL of cysts in control animals consisted of 3–4 sequential cellular layers, including the tegument, with an average thickness of 12.8 μm. Nuclei of GL cells were clearly visible (Fig. 2A). In cysts from drug-treated mice, the GL was substantially reduced to a thin layer, sometimes only represented by the tegument, with only a few discernible nuclei (Figs 2B and 2E). In cysts recovered from (S)-(−)-RBZ-Na treated mice, these alterations were less pronounced (Fig. 2F). Other changes detected in the GL of cysts in drug treated groups were desquamation, disruption, vacuolization, degeneration and in some cases also complete absence of germinal cells.

Fig. 2. Sections of paraffin-embedded E. granulosus cysts recovered from non-treated control (A, G) and drug treated (B–F, H) mice. Sections were stained with trichrome (A–F) and PAS (G) and PAS/AB (H), to visualize the GL, the adventitial layer (AL) and the LL. In (B), mice were treated with ABZ; in (C) with ABZ-Na; in (D) with RBZ-Na; in (E) and (H) with (R)-(+)-RBZ-Na; in (F) with (S)-(−)-RBZ-Na.
Histopathological changes of the LL were visualized by PAS, which stains glycogen and neutral mucopolysaccharides, and PAS/AB, which stains acid mucopolysaccharides. Although in cysts from control mice the LL was evenly stained and showed its typical lamellar, layered appearance (Fig. 2G), parasites from (R)-(+)-ABZ-Na-treated animals generally exhibited a structurally distorted LL, with many irregularities and AB staining intensity was stronger than PAS staining (Fig. 2H). This staining pattern was observed more frequently after treatment with the sodium salts of the drugs.
SEM: salts of BMZ induced alterations in the germinal layer of E. granulosus metacestodes
SEM was performed to visualize the GL and potential drug-induced alterations (Fig. 3). In control specimens (Fig. 3A), the GL exhibited a distinct surface structure, which was covered with numerous round cells of 3–5 μm in diameter which, based on their size and shape, were most likely undifferentiated cells that form an important part of the GL. In ABZ (Fig. 3B) and ABZ-Na (Fig. 3C) treated cysts, the surface of the GL was more flattened, the presence of these round cells was not as distinct, although structures resembling undifferentiated cells were also visible. In contrast, the GL surface of cysts obtained from RBZ-Na (Fig. 3D) (R)-(+)-RBZ-Na (Fig. 3E) and (S)-(−)-RBZ-Na (Fig. 3F) treated mice exhibited a much more fibrous structure, with small round cells only occasionally visible. In all specimens, larger bud-like formations of various sizes (up to 20 μm in diameter) could be detected (Figs 3B, 3C and 3F), which most likely represent various stages of cellular aggregates that could potentially be in the process of forming brood capsules.

Fig. 3. SEM of the GL surface of E. granulosus cysts recovered from non-treated control (A) and drug-treated (B–F) mice. Treatments in (B) = ABZ; (C) = ABZ-Na; (D) = RBZ-Na; (E) = (R)-(+)-RBZ-Na; (F) = (S)-(−)-RBZ-Na. Note the bud-like cellular aggregates marked with arrows.
Pharmacokinetic properties of BMZ and their salts in E. granulosus-infected mice analysed by HPLC
After final drug dosing, plasma and hydatid fluid samples were collected at different time points from E. granulosus-infected mice, and the concentrations of the metabolites of RBZ (ABZ-SO2), (R)-(+)-RBZ-Na and (S)-(−)-RBZ were measured by HPLC. Maximum plasma levels occurred after 15 min. The highest metabolite concentration was measured for the enantiomer (S)-(−)-RBZ after ABZ treatment (Fig. 4A), and at a similar level (approximately 50 μg mL−1) after treatment with the enantiomer itself (Fig. 4E). However, (S)-(−)-RBZ was also the major metabolite after treatments with RBZ-Na and ABZ-Na, albeit at considerably lower levels (approximately 20 and 8 μg mL−1, respectively; Figs 4B and 4C). (S)-(−)-RBZ was virtually undetectable in mice treated with (R)-(+)-RBZ-Na (Fig. 4D). ABZ-SO2 levels were in a similar range (peak of 8–10 μg mL−1) after ABZ, RBZ-Na and (R)-(+)-RBZ-Na treatments. The (R)-(+)-RBZ enantiomer exhibited low levels (5 μg mL−1 or below) after treatment with all compounds, except for after treatment with (R)-(+)-RBZ-Na (Fig. 4D). Latest at 180 min after initiation of drug treatment, none of the metabolites was detectable any more, except for after the application of ABZ, where the (S)-(−)-RBZ level was still elevated (Fig. 4A).

Fig. 4. Kinetics of the RBZ enantiomers (R)-(+)-RBZ-Na, (S)-(−)-RBZ and RBZ (ABZ-SO2) in plasma of mice treated with ABZ (A), ABZ-Na (B), RBZ-Na (C), (R)-(+)-RBZ-Na (D) and (S)-(−)-RBZ (E).
The concentrations of the two enantiomers of RBZ were also measured in the hydatid cyst fluids of the different experimental groups. These data and a comparison with the corresponding plasma levels are shown in Fig. 5. After treatments with ABZ, ABZ-Na, RBZ-Na and (S)-(−)-RBZ, only (S)-(−)-RBZ was detectable in cyst fluid (Fig. 5). (R)-(+)-RBZ-Na was only detected in cyst fluid upon treatment with that particular enantiomer (Fig. 5). Maximum levels of metabolites in the cyst fluid were reached 30 min after drug applications, thus 15 min after maximum levels in plasma were detected.

Fig. 5. Comparative kinetics of RBZ enantiomers (R)-(+)-RBZ-Na and (S)-(−)-RBZ in plasma and cyst fluid samples of mice treated with ABZ (A), ABZ-Na (B), RBZ-Na (C), (R)-(+)-RBZ-Na (D) and (S)-(−)-RBZ (E).
Discussion
The currently applied chemotherapeutical treatment of CE relies on the use of benzimidazole carbamate derivatives such as ABZ and mebendazole. In particular, ABZ has been widely used for the treatment of CE, but also for the treatment of alveolar echinococcosis, caused by the closely related cestode E. multilocularis (Siles-Lucas et al., Reference Siles-Lucas, Casulli, Cirilli and Carmena2018). However, ABZ exhibits a low water solubility, and is therefore not well absorbed, which in turn warrants drug administration high dosage and prolonged periods. This may increase the risk of adverse events and makes the therapy cumbersome for patients. Formulations that exhibit higher water solubility are predicted to be more efficacious in a shorter period, addressing both drawbacks. The development of new formulations of benzimidazoles with improved water-solubility reported here is a major step towards improved chemotherapeutical options against CE.
Our results show that sodium salt formulations of ABZ, RBZ and (R)-(+)-RBZ-Na formulations, but not of the (S)-(−)-RBZ enantiomer, exhibit promising efficacy in the murine model of secondary CE, as assessed by weight decrease of the total intra-peritoneal cyst mass after 1 month of treatment subsequent to a 9-month old infection. In addition, alterations of the GL and LL structure of cysts from mice treated with these drug formulations were observed at the microscopic level when compared to those recovered from control mice.
The higher efficacy of (R)-(+)-RBZ-Na in this in vivo study confirms what has been previously suggested for (R)-(+)-RBZ in others in vitro or ex vivo studies on zoonotic helminths such as Taenia solium, Fasciola hepatica, Trichinella spiralis and E. granulosus (Alvarez et al., Reference Alvarez, Imperiale, Sánchez, Murno and Lanusse2000; Bolás-Fernández et al., Reference Bolás-Fernández, Rama-Iñiguez and Torrado2004; Bao et al., Reference Bao, Zhang, Jing, Qiao and Wang2008; Paredes et al., Reference Paredes, de Campos Lourenço, Marzal, Rivera, Dorny, Mahanty, Guerra-Giraldez, García, Nash and Cass2013).
Pharmacokinetic studies of ABZ application in mice have been reported earlier in a study focusing on E. multilocularis infection (Stettler et al., Reference Stettler, Rossignol, Fink, Walker, Gottstein, Merli, Theurillat, Thormann, Dricot, Segers and Hemphill2004), but only ABZ-SO2 levels were measured. In this earlier study, ABZ-SO2 levels peaked at 1 h post drug application (no earlier time points were measured), whereas serum levels reached a maximum of 6 μg mL−1, and declined rapidly thereafter. Taking into account the different experimental settings in terms of vehicle, timing of drug application, dosing etc., the results of this study are rather similar. The novelty of the approach reported in this paper is the analysis of the RBZ enantiomer plasma levels. Interestingly, plasma levels of (S)-(−)-RBZ were always higher than those of (R)-(+)-RBZ in our model, which is in accordance with the known predominance of (S)-enantiomer formation after treatment with a racemic drug in rats and mice (Delatour et al., Reference Delatour, Benoit, Caude and Tambute1990, Reference Delatour, Benoit, Besse and Boukraa1991; García et al., Reference Garcia, Bolás-Fernández and Torrado1999, Reference García, Bolás and Torrado2003; Capece et al., Reference Capece, Navarro, Arcalis, Castells, Toribio, Perez, Carretero, Ruberte, Arboixa and Cristòfola2003). Also, intra-cystic levels of (R)-(+)-RBZ were not measurable in any group, with the exception of mice directly treated with (R)-(+)-RBZ-Na, suggesting that intra-cystic level of (R)-(+)-RBZ could be below the detection limit even at the second assessed time point (15 min post-dosage). These results, together with the shape of the pharmacokinetic plasma curves, indicate a rapid metabolization of the compounds, which impairs measurements of the actual C max in our experiments. However, the presence of measurable plasma levels of the (R)-(+)-RBZ-Na enantiomer coincided with the most efficacious treatments with ABZ-Na and (R)-(+)-RBZ-Na in terms of mean cyst weight reduction.
Our results are an important addition to the potential arsenal of benzimidazole formulations that could be used for the treatment of CE (Siles-Lucas et al., Reference Siles-Lucas, Casulli, Cirilli and Carmena2018). Drug repurposing or modification of existing drugs are the way forward, since the development of novel compounds for the treatment of CE is hampered by the expected low-level market return, inducing the pharmaceutical industry not to invest in drug discovery for this disease. Benzimidazoles are attractive compounds as they are widely used, and data on their pharmacological properties are widely available. Improving these properties through novel formulations will be an important step in drug development for neglected diseases, including CE. Despite considerable efforts and interesting results obtained in vitro (Siles-Lucas et al., Reference Siles-Lucas, Casulli, Cirilli and Carmena2018), only a few drugs investigated for repurposing for CE have been shown to be efficacious in vivo. Combination treatments using FBZ and netobimin, which is a prodrug of ABZ used as a veterinary anthelmintic (Garcia-Llamazares et al., Reference Garcia-Llamazares, Alvarez-de-Felipe, Redondo- Cardeña, Voces-Alonso and Prieto-Fernández1997), were shown to be efficacious in a rodent CE model. In E. granulosus-infected sheep and goats, oxfendazole and oxfendazole combined with nitazoxanide, were shown to be as efficacious as ABZ whereas not requiring daily uptake of the drug due to their prolonged bioavailability (Gavidia et al., Reference Gavidia, Gonzalez, Lopera, Jayashi, Angelats, Barron, Ninaquispe, Villarreal, Garcia, Verastegui and Gilman2009; Gonzalez et al., Reference Gonzalez, Codd, Horton, Garcia and Gilman2019). Other benzimidazoles exhibiting interesting protoscolicidal and metacestodicidal activity in vivo are flubendazole (Elissondo et al., Reference Elissondo, Ceballos, Dopchiz, Andresiuk, Alvarez, Sánchez Bruni, Lanusse and Denegri2007; Ceballos et al., Reference Ceballos, Elissondo, Sánchez B, Denegri, Lanusse and Alvarez2015) and nocodazole (Shkoliar et al., Reference Shkoliar, Kukhaleva, Legon'kov and Kovalenko2014). However, all these compounds have not been licensed for the use in humans to date.
Conclusion and future perspectives
These new formulations of salts of RBZ and its enantiomers exhibit a number of potential advantages such as increased water solubility, a possible parenteral administration for animal and human use, improved bioavailability in plasma, and thus better anthelmintic activity. Moreover, the improved bioavailability helps to decrease adverse reactions of BMZ drugs (namely hepatotoxicity, alopecia, gastrointestinal disturbances, thrombocytopaenia and severe leucopaenia), through decreasing dosage of racemic and enantiopure formulations. In addition to this, since in vivo racemization was not observed after administration of individual enantiomers, a therapeutic application of enantiopure forms of sulphoxides could be envisaged.
Moreover, because of these encouraging experimental results and the simple, rapid and affordable synthesis of both racemic and enantiopure forms of salts of BMZ, the proposed formulations are suitable for a sustainable and affordable large-scale pharmaceutical development of these alkaline salts for treatment of helminth infectious diseases (Cirilli et al., Reference Cirilli, Guglielmi, Formica, Casulli and Carradori2017).
The way forward will be to test the efficacy, pharmacokinetics and more interestingly the toxicity of these potential new drug formulations in different helminth species.
Supplementary material
The supplementary material for this article can be found at https://doi.org/10.1017/S0031182020001225.
Acknowledgements
The authors would like to thank Francesca Tamarozzi for her critical comments.
Financial support
The research leading to these results received funding from the European Union Seventh Framework Programme (FP7/2007-2013) under the project HERACLES (http://www.heracles-fp7.eu/), grant agreement no. 602051. The funder of the study had no role in study design, data collection, data analysis, data interpretation or writing of the report.
Conflict of interest
None.
Ethical standards
This study was previously approved by the Namik Kemal University Animal Experiments Local Ethics Committee (NKUHADYEK 2015/12). The experimental test strictly followed all procedures recommended by Regulation on the welfare and protection of animals used for experimental and other scientific purposes, Ministry of Food, Agriculture and Livestock, Turkey (Reg: 2011/28141).