Introduction
Coccidiosis of sheep is a frequently encountered disease caused by members of the apicomplexan genus Eimeria (Kaufmann, Reference Kaufmann1996). Coccidiosis manifests as diarrhoea, slow weight gain and occasionally death, and represents a significant economic burden to the sheep farming industry worldwide (Chartier and Paraud, Reference Chartier and Paraud2012). Chronic asymptomatic Eimeria infections are extremely common; surveys around the world have shown that most individuals are infected and shed oocysts in their feces, typically in low numbers, thereby providing a constant source of infection for other animals (O'Callaghan et al., Reference O'Callaghan, O'Donoghue and Moore1987; Dittmar et al., Reference Dittmar, Mundt, Grzonka, Daugschies and Bangoura2010). At least 15 different Eimeria species have been encountered in sheep (Kaufmann, Reference Kaufmann1996), although not all have been associated with coccidiosis. Eimeria ovinoidalis, E. crandallis and, to a lesser extent, E. bakuensis, E. parva and E. ahsata are more frequently linked with the disease (Skirnisson, Reference Skirnisson2007) than other species. The relative frequencies with which infection by different Eimeria species is encountered vary; E. crandallis and E. parva appear to be the most abundant species worldwide (Gul and Deger, Reference Gul and Deger2002), although E. weybridgensis, E. ovinoidalis and E. bakuensis are also common in Europe (Reeg et al., Reference Reeg, Gauly, Bauer, Mertens, Erhardt and Zahner2005), but mixed infections by two or more Eimeria species are the norm (Arslan et al., Reference Arslan, Umur and Kara1999). Epidemiological surveys have identified numerous risk factors for Eimeria infection including animal age, with more infections in younger individuals, and season, with a higher prevalence of infection during wetter periods of year (de Souza et al., Reference de Souza, da Cruz, Neto, Albuquerque, Melo and Tapia2015). An individual's sex may also be influential (de Souza et al., Reference de Souza, da Cruz, Neto, Albuquerque, Melo and Tapia2015) as is their physiological status; detectable levels of shedding are more common during pregnancy (Dittmar et al., Reference Dittmar, Mundt, Grzonka, Daugschies and Bangoura2010). Furthermore, correlates with infection intensity have also been reported, with more intense infections in young animals (Reeg et al., Reference Reeg, Gauly, Bauer, Mertens, Erhardt and Zahner2005).
Campylobacter is the most frequently reported agent of bacterial gastroenteritis in many developed countries including the UK, where over 50 000 laboratory-confirmed cases are reported each year (PHE, 2018). Campylobacteriosis is a zoonosis and ruminant livestock are an important source of human infections (Thépault et al., Reference Thépault, Méric, Rivoal, Pascoe, Mageiros, Touzain, Rose, Béven, Chemaly and Sheppard2017). Surveys in the UK have revealed a prevalence of infection of up to about 50% of grazing animals (Stanley et al., Reference Stanley, Wallace, Currie, Diggle and Jones1998; Jones, Reference Jones2001; Grove-White et al., Reference Grove-White, Leatherbarrow, Cripps, Diggle and French2010; Sproston et al., Reference Sproston, Ogden, MacRae, Dallas, Sheppard, Cody, Colles, Wilson, Forbes and Strachan2011). Epidemiological studies have identified various risk factors for Campylobacter infection in sheep including a higher prevalence associated with increased stocking density and pasture quality, and a season pattern, with a peak prevalence of infections coinciding with lambing, weaning and the summer months (Stanley et al., Reference Stanley, Wallace, Currie, Diggle and Jones1998; Jones., Reference Jones2001; Grove-White et al., Reference Grove-White, Leatherbarrow, Cripps, Diggle and French2010; Sproston et al., Reference Sproston, Ogden, MacRae, Dallas, Sheppard, Cody, Colles, Wilson, Forbes and Strachan2011).
Eimeria and Campylobacter represent two of a broad diversity of parasitic taxa associated with sheep that includes viruses, bacteria and eukaryotes such as protists, helminths and arthropods. Studies in sheep and other vertebrate hosts have demonstrated that co-infecting parasites often interact thereby shaping susceptibility to, and dynamics of, infection by each parasite (Craig et al., Reference Craig, Tempest, Pilkington and Pemberton2008; Telfer et al., Reference Telfer, Birtles, Bennett, Lambin, Paterson and Begon2008; Salgame et al., Reference Salgame, Yap and Gause2013; Leung et al., Reference Leung, Graham and Knowles2018). These co-infections may have specific veterinary and food safety implications (Thumbi et al., Reference Thumbi, Bronsvoort, Poole, Kiara, Toye, Mbole-Kariuki, Conradie, Jennings, Handel, Coetzer, Steyl, Hanotte and Woolhouse2014; Van Wyk et al., Reference Van Wyk, Goddard, de C Bronsvoort, Coetzer, Handel, Hanotte, Jennings, Lesosky, Kiara, Thumbi, Toye, Woolhouse and Penzhorn2014) and, ecologically, have consequences for host condition and fitness (Telfer et al., Reference Telfer, Lambin, Birtles, Beldomenico, Burthe, Paterson and Begon2010). The impact of co-infectors on ovine Eimeria infections has yet to be assessed, but studies in other vertebrates have indicated Eimeria interacts with other parasites and influence their infection dynamics (Knowles et al., Reference Knowles, Fenton, Petchey, Jones, Barber and Pedersen2013; Mason et al., Reference Mason, Dubey, Smith and Boag2015) and host response (Zhang et al., Reference Zhang, Thabet, Hiob, Zheng, Daugschies and Bangoura2018).
In this study, we used longitudinal monitoring of farmed sheep flocks in north-west England to determine the seasonal dynamics of Eimeria and Campylobacter infections and to explore the biotic and abiotic determinants of these.
Materials and methods
Sheep surveys
Surveys were carried out on two sheep farms, stock from both of which were grazed on Bethecar Moor, an extensive tract of common land (approximately 550 hectares) in southern Cumbria (north-west England). Farm A maintained a flock of approximately 600 Swaledale sheep which spent most of the year (about 300 days) on the Moor and were herded only five times per annum for brief periods (Table 1). Farm B maintained a flock of about 200 sheep, the majority of which were Texel but some Swaledale too. These animals were more intensively managed than those on Farm A, being herded far more regularly and spending markedly more time (about 160 days) on pasture around the farm (Table 1).
Table 1. Details of husbandry practices on study farms

On Farm A, sheep were surveyed on 11 occasions between September 2014 and September 2016. Each survey corresponded to the events described above bar March 2015. On Farm B, sheep were surveyed on 12 occasions between July 2015 and September 2016 (roughly once a month bar December 2015, January 2016 and June 2016). On each visit to each farm, freshly voided fecal samples were collected between 20 and 60 opportunistically selected sheep. Approximately 5 g of feces were collected into a 60 mL collection pot which was then immediately placed on ice held in a cold box.
The age of each sheep was estimated as being either <12, between 12 and 24, or >24 months on the basis of its age, size and condition on the advice of and with the help of farmers/experienced farm hands.
Isolation, quantification and identification of Eimeria species
About 4 g of feces were added to 56 mL sterile distilled water and repeatedly mixed until a homogeneous suspension was obtained, which was then transferred to a sterile container through a tea-strainer. Ten microlitres of this filtrate were centrifuged at 172 × g for 5 min then the supernatant discarded, and the pellet resuspended in 4 mL of flotation fluid (saturated NaCl solution containing 500 g per litre of glucose). In total, 50 μL of this suspension were transferred to two chambers of a McMaster slide then left for 5 min to allow any oocysts in the suspension to rise to the surface. Oocysts were counted by observation under 100 × magnification and standardized to oocysts per 1 g of feces.
Any sample in which Eimeria oocysts were observed was subjected to an induced sporulation process. One volume of sheep feces was resuspended in two volumes of 2.5% (w/v) potassium dichromate solution, sieved to remove coarse material then poured as a thin layer into a petri dish. These oocyst suspensions were then held for 10 days at room temperature to allow sporulation to occur. After this period, sporulated oocysts were pelleted by centrifugation at 1077 × g for 5 min then resuspended in flotation fluid such that a meniscus is formed at the top of the centrifuge tube. A cover slide was placed on the top of the centrifuge tube and left for 5 min, then carefully removed and observed under 100× magnification. The identity of oocysts present was determined by reference to a taxonomic key (Eckert et al., Reference Eckert, Taylor, Catchpole, Licois, Coudert, Bucklar, Eckert, Braun, Shirley and Coudert1995).
Isolation and identification of Campylobacter species
In total, 0.5 g of fresh (<24 h since collection) fecal material were suspended in 5 mL modified Preston broth (Oxoid, Basingstoke, Hampshire, UK) by vortexing. The suspension was incubated at 42°C for 24 h in a microaerophilic atmosphere, then 100 μL of this suspension was spread onto charcoal cefoperazone deoxycholate agar agar (Oxoid). Plates were incubated at 42°C for 48 h in a microaerophilic atmosphere after which plates were examined for growth. Putative Campylobacter isolates were identified on the basis of colonial morphology and Gram staining. In order to confirm the identity of these isolates, boiled suspensions of colonies were incorporated as a template into a Campylobacter genus-specific PCR (Linton et al., Reference Linton, Lawson, Owen and Stanley1997). A subset of 20 amplicons obtained from samples collected on Farm A were further characterized using Sanger sequencing, and all samples from Farm B that yielded an amplicon were retested using additional Campylobacter-specific PCRs (Yang et al., Reference Yang, Jiang, Huang, Zhu, Yin, Gong and Yu2004; Vondrakova et al., Reference Vondrakova, Pazlarova and Demnerova2014).
Statistical analysis
All statistical analyses were performed using R software version 3.4 (R Development Core Team, 2015). To investigate factors that influenced an individual sheep's probability of being infected with Eimeria and/or Campylobacter species, generalized linear models (GLMs) were used that assumed a binomial error term and a logit link. Factors considered included age, season (spring = months March–May, summer = June–August, autumn = September–November, winter = December–February), farm, weather (rainfall/temperature) and co-infection. In addition to considering infection at the genus level, this method was also employed for the two most common Eimeria species, E. ovinoidalis and E. bakuensis. To investigate whether the potential non-independence of samples from the same farm was important, generalized linear mixed models with a binomial error term and a logit link were employed where farm was included as a random effect
To investigate factors that influenced the intensity of Eimeria infections, GLMs with a negative binomial error term and a log link were employed. The same factors as listed above were considered. Model selection was based on a backward stepwise model selection with variables dropped according to P value, with only those variables significant at the P < 0.05 level being retained in the final model.
Results
Epidemiological patterns
A total of 360 fecal samples were collected from sheep on Farm A. Of these, 350 were tested for the presence of Eimeria oocysts and 353 were tested for the presence of Campylobacter (Table 2). In total, Eimeria oocysts were present in 281 (80.0%) samples, with the prevalence of fecal shedding ranging from 66.7 to 97.6% between surveys and a mean intensity ranging from 61 to 2672 oocysts per gram of feces between surveys. The overall prevalence of Campylobacter infections, as determined by PCR, was 32.6% (115/353), with a range of 21.9–63.2% between surveys (Table 2). Sanger sequencing of 20 PCR amplicons confirmed them all to be derived from Campylobacter species.
Table 2. Prevalence and intensity of Eimeria infections, and prevalence of Campylobacter infections on Farm A

s.e., standard error.
A total of 440 fecal samples were collected from Farm B. Of these, 433 were tested for the presence of Eimeria oocysts and 430 were tested for the presence of Campylobacter (Table 3). In total, Eimeria oocysts were present in 327 (75.5%) samples, with a prevalence of infection ranging from 53.3 to 100% between surveys and a mean intensity ranging from 62 to 460 oocysts per gram of feces between surveys. The overall prevalence of Campylobacter infections, as determined by genus and a combination of species-specific PCRs, was 29.1% (125/430), with a range of 10.8–62.5% between surveys (Table 3).
Table 3. Prevalence and intensity of Eimeria infections, and prevalence of Campylobacter infections on Farm B.

s.e., standard error.
The two most commonly encountered Eimeria species in this study were E. ovinoidalis and E. bakuensis (Table 4). Both species were frequently encountered in samples collected from both study farms. In total, E. ovinoidalis oocysts were present in 179 (51.1%) of samples collected on Farm A and 192 (44.3%) of samples collected on Farm B. Eimeria bakuensis oocysts were present in 136 (38.9%) of samples collected on Farm A and 198 (45.7%) of samples collected on Farm B (Table 4).
Table 4. Prevalence of E. ovinoidalis and E. bakuensis infections on Farms A and B
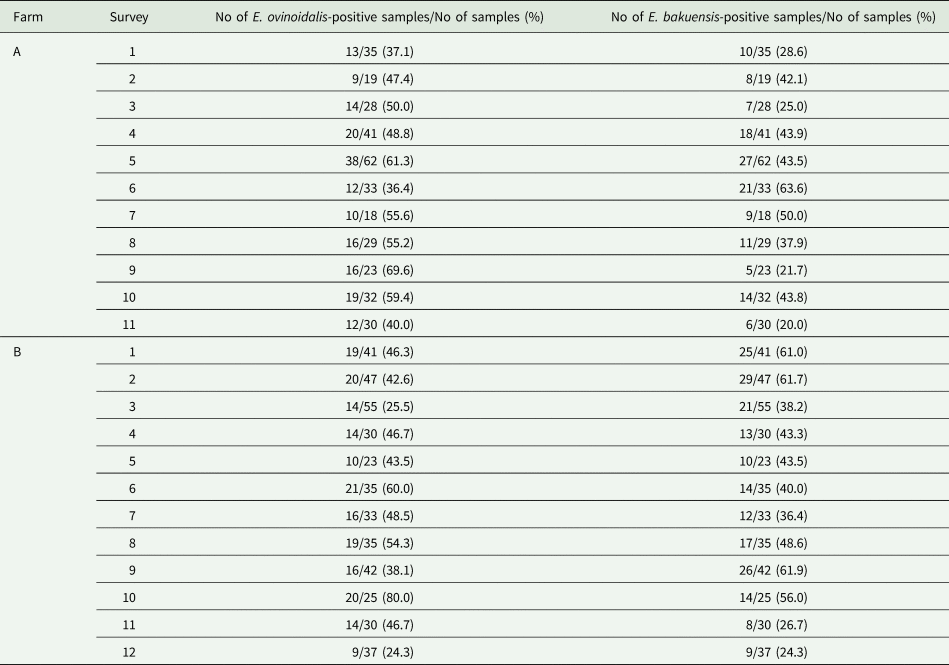
Statistical modelling
Infection with Eimeria spp. or Campylobacter spp.
An animal's age was a predictor of Eimeria infection, with infections being significantly more common in young animals (Table 5). There was a seasonal pattern in Eimeria infection rates, which were significantly higher in winter, spring and summer than in autumn. Campylobacter infection prevalence also varied significantly with season, being higher in winter and spring than in autumn.
Table 5. Parameter estimates and standard errors for GLM models
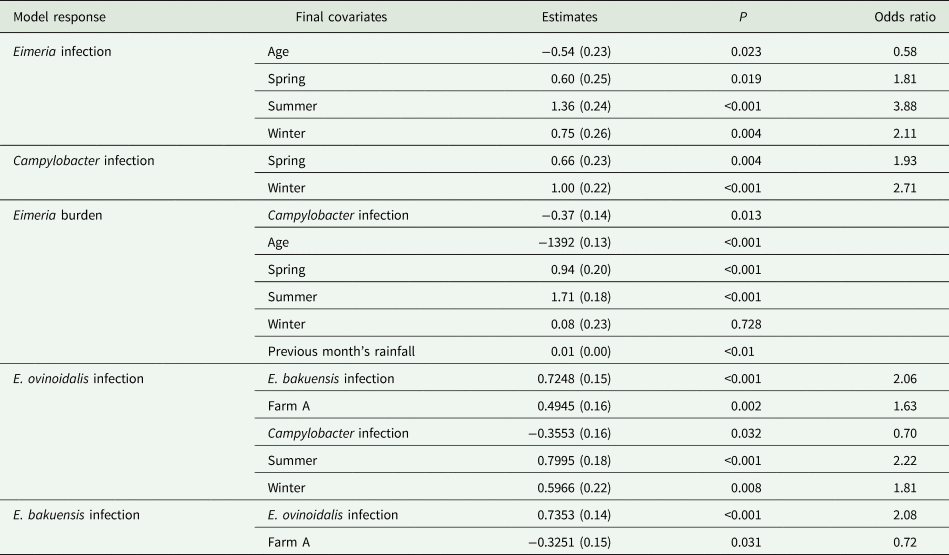
Only statistically significant results are shown.
Infection with E. ovinoidalis or E. bakuensis
Coinfection appears to be an important predictor of an individual's probability of infection, with animals infected with E. bakuensis being significantly more likely to also be infected with E. ovinoidalis. However, infection with Campylobacter reduced the probability of an individual having E. ovinoidalis (Table 5). Season also affected infection probability for E. ovinoidalis, with increased infection in summer and winter compared to autumn. Interestingly, E. ovinoidalis infections were more likely at Farm A, whilst E. bakuensis infections were more likely at Farm B (Table 5).
Eimeria spp. burdens
We also observed seasonal variation in the intensity of Eimeria infections, with infections being significantly more intense in spring and summer than in autumn. Eimeria infection intensity also correlated with the amount of rainfall during the month prior to sampling. Interestingly, Eimeria infection intensity also correlated with Campylobacter infection; Eimeria was present at significantly lower intensities in co-infected animals than in those in which only Eimeria was detected (Table 5).
Discussion
This study explored the epidemiology of Eimeria and Campylobacter infections in flocks of naturally infected sheep in Cumbria, UK, cataloguing their diversity and charting their temporal dynamics over a 14-month period. Overall, the epidemiology of Eimeria infections in our study population appeared to be ‘typical’ in that, in keeping with previous studies, we observed that (i) the majority of animals shed Eimeria oocysts throughout the duration of the survey, (ii) E. ovinoidalis was the most prevalent (of 10) ovine-associated Eimeria species encountered, (iii) infection rates and intensities were more common in younger animals and (iv) infection rates and intensities varied with season (e.g. Joyner et al., Reference Joyner, Norton, Davies and Watkins1966; O'Callaghan et al., Reference O'Callaghan, O'Donoghue and Moore1987; Dittmar et al., Reference Dittmar, Mundt, Grzonka, Daugschies and Bangoura2010; de Souza et al., Reference de Souza, da Cruz, Neto, Albuquerque, Melo and Tapia2015; Carrau et al., Reference Carrau, Silva, Pérez, Failing, Martínez-Carrasco, Macías, Taubert, Hermosilla and de Ybáñez2018). The relative high frequency with which we encountered E. bakuensis was noteworthy, although geographical variation in the relative abundance of ovine-associated Eimeria species is recognized while far from fully explored (de Souza et al., Reference de Souza, da Cruz, Neto, Albuquerque, Melo and Tapia2015; Carrau et al., Reference Carrau, Silva, Pérez, Failing, Martínez-Carrasco, Macías, Taubert, Hermosilla and de Ybáñez2018). The basis of such variation is unclear, although adaptation of different species to climatic conditions has been proposed (de Souza et al., Reference de Souza, da Cruz, Neto, Albuquerque, Melo and Tapia2015; Carrau et al., Reference Carrau, Silva, Pérez, Failing, Martínez-Carrasco, Macías, Taubert, Hermosilla and de Ybáñez2018). However, this proposal included the association of E. ovinoidalis to dry arid climates, which is at odds with our observation of this species at high prevalence in North West England, where precipitation levels are amongst the highest in Europe (https://www.eea.europa.eu/data-and-maps/figures/average-annual-precipitation). Other possibilities include variation in the rate and efficiency of sporulation, as has been described for some avian infecting eimerians (Norton and Chard, Reference Norton and Chard1983), possibly biasing efficient replication by different Eimeria species under different environmental conditions.
Similarly, the epidemiology of Campylobacter infections was also akin to that described in previous surveys; our overall infection prevalence estimates of 32.6% on Farm A and 29.1% on Farm B are in close agreement with the findings of other surveys of sheep in the UK (Stanley and Jones, Reference Stanley and Jones2003; Grove-White et al., Reference Grove-White, Leatherbarrow, Cripps, Diggle and French2010; Sproston et al., Reference Sproston, Ogden, MacRae, Dallas, Sheppard, Cody, Colles, Wilson, Forbes and Strachan2011), as is our observation of a clear seasonal variation in infection prevalence. However, whereas others have reported higher infection prevalence in spring and summer (Stanley and Jones, Reference Stanley and Jones2003; Grove-White et al., Reference Grove-White, Leatherbarrow, Cripps, Diggle and French2010), in our survey, animals were more likely to be infected in winter and spring. This discrepancy may reflect differences in husbandry/farm management practices, which have been shown to influence Campylobacter shedding rates (Grove-White et al., Reference Grove-White, Leatherbarrow, Cripps, Diggle and French2010).
Interestingly, we observed that the intensity of Eimeria oocyst shedding (but not their prevalence) was significantly lower in animals co-infected with Campylobacter than in animals not co-infected with Campylobacter. This observation suggests that either the presence of Campylobacter leads to a reduction in Eimeria infection intensity or that higher Eimeria infection intensities impede Campylobacter colonization of the intestine. Previous work suggests both alternatives are feasible. Bacterial interference of Eimeria activity has been observed in vitro, with secretions from Lactobacillus species being shown to impede Eimeria tenella invasion of cultures of Madin-Darby bovine kidney cells (Tierney et al., Reference Tierney, Gowing, Van Sinderen, Flynn, Stanley, McHardy, Hallahan and Mulcahy2004). Furthermore, the ability of bacteria to interfere with the development of apicomplexan oocyst development (in vitro) has been demonstrated for Cryptosporidium (Deng et al., Reference Deng, Nuanualsuwan and Cliver2001). The impact of parasite infections on the composition and activity of the microbiome is now well accepted based on studies of experimental (Hayes et al., Reference Hayes, Bancroft, Goldrick, Portsmouth, Roberts and Grencis2010; Cantacessi et al., Reference Cantacessi, Giacomin, Croese, Zakrzewski, Sotillo, McCann, Nolan, Mitreva, Krause and Loukas2014) and naturally acquired (Kreisinger et al., Reference Kreisinger, Bastien, Hauffe, Marchesi and Perkins2015) infections. These studies include several that have explored the interaction between coccidian parasites and the microbiome; evidence of intestinal coccidial infection-induced perturbation of the microbiome has been reported in BALB/c mice (Huang et al., Reference Huang, Tang, Bi, Hao, Han, Suo, Zhang, Wang, Duan, Yu, Yu, Yu, Lv, Suo and Liu2018a) and chickens (Macdonald et al., Reference Macdonald, Nolan, Harman, Boulton, Hume, Tomley, Stabler and Blake2017; Huang et al., Reference Huang, Zhang, Zhou, Tang, Li, Wang, Tang, Suo, Jia, El-Ashram, Yu, Cai, Gupta, Suo and Liu2018b). Perhaps of most relevance to our study are observations drawn from experimental co-infection of poultry with Eimeria parasites and various enteric bacterial pathogens. The presence of Eimeria was found to enhance the growth of Clostridium perfringens and Salmonella Typhimurium in experimentally infected chickens (Arakawa et al., Reference Arakawa, Baba and Fukata1981; Collier et al., Reference Collier, Hofacre, Payne, Anderson, Kaiser, Mackie and Gaskins2008) and, very recently, Macdonald et al. (Reference Macdonald, van Diemen, Martineau, Stevens, Tomley, Stabler and Blake2019) observed that increased fecal shedding of C. jejuni was associated with concomitant E. tenella infection in chickens. The results of one trial in this study indicated that fecal shedding rates of E. tenella oocysts were not affected by the presence of C. jejuni, whereas in another trial, C. jejuni infection intensities in the caeca and cloaca were significantly higher in birds co-infected with a higher dose of E. tenella than birds which received 10 times fewer parasites. The disparity between these observations and our results is noteworthy, since the association between E. tenella infection and increased C. jejuni load was detected during pathogenic parasite challenge that incurred notable haemorrhagic pathology. The increased mucus secretion and haemorrhage caused by concurrent pathogenic E. tenella challenge of chickens likely served to provide a source of nutrients and iron in support of C. jejuni proliferation (Palyada et al., Reference Palyada, Threadgill and Stintzi2004; Van Deun et al., Reference Van Deun, Pasmans, Ducatelle, Flahou, Vissenberg, Martel, Van den Broeck, Van Immerseel and Haesebrouck2008). The lower levels of Eimeria oocyst shedding described herein suggest a parasite–host system in enzootic stability, lacking such pathologies. Exploration of our findings in an aptly designed experimental model of sub-clinical and clinical disease would be worthwhile.
The analyses of Eimeria species level interactions provide another possible explanation for the observed lower intensity of Eimeria (genus) infections in animals co-infected with Campylobacter. These analyses indicated that infection with Campylobacter reduced the probability of an individual having E. ovinoidalis but did not significantly affect the probability of an individual having E. bakuensis. Thus, it may be that Campylobacter interacts with some but not all Eimeria species and that the observed loss of Eimeria infection intensity resulted from a reduction in the diversity of co-infecting Eimeria species.
Coinfection by different Eimeria species was also an important predictor of an individual's probability of infection. A sheep infected with E. bakuensis was significantly more likely to also be infected with E. ovinoidalis and vice-versa. Mixed infections with two or more Eimeria species are the norm for many reservoir hosts, including sheep, but as yet little work has been reported exploring competitive or facilitative interactions between species. Seville et al. (Reference Seville, Stanton and Gerow1996) surveyed infections by six Eimeria species in Wyoming ground squirrels (Spermophilus elegans) and reported several positive interactions between species but found no evidence for inter-species competition. Similarly, positive interaction was reported between co-infecting Eimeria species in red squirrels (Sciurus vulgaris) (Bertolino et al., Reference Bertolino, Wauters, De Bruyn and Canestri-Trotti2003) and guanacos (Lama guanicoe) (Moreno et al., Reference Moreno, Eberhardt, Lamattina, Previtali and Beldomenico2013). All authors proposed that these positive correlations reflected shared transmission pathways for different species, an explanation that may well also be valid for our observations. Conversely, other groups have reported apparent competition between co-infecting Eimeria species, based on surveys of co-infections in lizards (Phelsuma ornate) (Leinwand et al., Reference Leinwand, Kilpatrick, Cole, Jones and Daszak2005) and rabbits (Sylvilagus floridanus) (Bertolino et al., Reference Bertolino, Hofmannova, Girardello and Modry2010).
In conclusion, the epidemiologies we quantified for Eimeria and Campylobacter infections in sheep align well with those described previously, and we confirm the influence of previously established epidemiological determinants. However, the concurrent survey of both taxa has revealed interactions between co-infectors that potentially affect the transmission dynamics of both. The importance of such interactions is well recognized among parasite ecologists (Telfer et al., Reference Telfer, Lambin, Birtles, Beldomenico, Burthe, Paterson and Begon2010), but they are also clearly relevant to applied veterinary science given the strong correlation between intestinal microbiome structure, feed efficiency and thus livestock productivity (Stanley et al., Reference Stanley, Denman, Hughes, Geier, Crowley, Chen, Haring and Moore2012; Patil et al., Reference Patil, Ellison, Wolff, Shearer, Wright, Cockrum, Austin, Lamberson, Cammack and Conant2018). Finally, our work underlines the value of livestock as models for studying the dynamics of natural parasite communities, particularly animals, such as the sheep we worked with, that graze all year round on rough pasture. Each sheep can be easily identified, and losses are low, so long-term repeated sampling of individuals is straight-forward. Furthermore, reagents for quantification of numerous biomarkers are commercially produced, indicators of fitness (productivity) are well established, and medicines of proven efficacy against specific parasites are available for intervention studies.
Acknowledgements
We thank the farmers who not only allowed us onto the farms but also provided huge assistance in the collection of samples and data for this study.
Financial support
The work reported herein forms part of RTAN's PhD studies, funded by The Iraqi Ministry of Higher Education.
Conflict of interests
None.
Ethical standards
Ethical approval for this study was granted by the Ethics Committee of The University of Salford, UK.