Entamoeba histolytica and amoebiasis
The parasitic amoeba E. histolytica is a human pathogen that lives as commensal in the host's colon. This single-celled eukaryote exists in two forms during its life cycle: as a dormant cyst that contaminates food and water, and (following cyst germination) as a trophozoite (Marie and Petri Jr, Reference Marie and Petri2014). Trophozoites feed on bacteria of the intestinal flora, divide and encyst. Hence, newly formed cysts are released into the environment with the stools. Unlike many protozoan parasite species, E. histolytica does not depend on a vector for the transmission because the cysts themselves perpetuate the life cycle by fecal–oral spreading. Amoebiasis has been classified by the US Centers for Disease Control and Prevention as a disease associated with critical bioterrorism-associated agents (i.e. Category 3 agents). Similarly, E. histolytica’s relatively easy transmission and its associated mortality and morbidity has prompted the US National Institute of Allergy and Infectious Diseases to classify it as a Category B agent (i.e. a pathogen for which diagnostic capacity and disease surveillance should be enhanced).
To a great extent, commensal microorganisms condition their environment and produce minimal damage to the host; this is important because they must regulate their numbers and immunomodulate the host to promote survival.
The majority of E. histolytica infections are asymptomatic. In rural populations in India, Africa and Latin America, the prevalence of E. histolytica infection ranges from 4 to 10%. The fact that symptoms of invasive amoebiasis develop in only one in five infected persons (Watanabe and Petri Jr, Reference Watanabe and Petri2015) suggests that 95% of individuals carry commensal trophozoites. Invasive trophozoites convert from a commensal phenotype to a pathogenic phenotype; they invade the intestinal mucosal barrier, exhaust nutrients in the mucus layer, and cause intestinal disorders by manipulating the host's immune system more intensively. The resulting intestinal symptoms range from frequent, mild diarrhoea to dysentery with blood and mucus in the stools. Although the stimuli that trigger the switch from the commensal stage to the pathogenic stage have yet to be identified, description of the early steps in amoebiasis provides a better understanding of the concept of virulence. The amoeba must adapt rapidly to the different environments encountered, in order to attack the host and avoid being killed. In contrast, excessive host destruction must also be avoided, since it would inevitably lead to the parasite's own death; E. histolytica cannot form cysts outside the intestinal lumen. The establishment of invasive amoebiasis is reportedly associated with human factors (Duggal et al. Reference Duggal, Guo, Haque, Peterson, Ricklefs, Mondal, Alam, Noor, Verkerke, Marie, Leduc, Streamson, Martin, Leibel, Houpt, Gilchrist, Sher, Porcella and Petri2011), environmental conditions (Petri Jr et al. Reference Petri, Mondal, Peterson, Duggal and Haque2009; Mondal et al. Reference Mondal, Minak, Alam, Liu, Dai, Korpe, Liu, Haque and Petri2012) and the parasite's virulence state (Olivos et al. Reference Olivos, Ramos, Nequiz, Barba, Tello, Castañón, González, Martínez, Montfort and Pérez-tamayo2005; Faust and Guillen, Reference Faust and Guillen2012). Trophozoites can also spread through the systemic circulation and reach the liver, where they form abscesses. Hence, dysentery and liver abscesses are the hallmarks of E. histolytica amoebiasis.
Intestinal invasion by E. histolytica
The versatile nature of E. histolytica’s virulence in humans suggests that intestinal invasion by commensal trophozoites is triggered on the luminal side of the colon. Nothing is known about the contribution of luminal resident bacterial flora to amoebic survival and pathogenesis. Nevertheless, the importance of the human microbiota in E. histolytica infection is highlighted by the fact that germ-free animals inoculated with E. histolytica do not develop amoebic ulcers (Phillips et al. Reference Phillips, Wolfe, Rees, Gordon, Wright and Reyniers1955). Hence, the presence of gut microbiota promotes infection by the parasite. Curiously, trophozoites isolated from patients suffering from amoebiasis progressively lose their virulence in axenic cultures (Olivos et al. Reference Olivos, Ramos, Nequiz, Barba, Tello, Castañón, González, Martínez, Montfort and Pérez-tamayo2005). Furthermore, recent studies have highlighted significant changes in the predominant gut bacteria in individuals infected with E. histolytica (Verma et al. Reference Verma, Verma, Ahuja and Paul2012), with a decrease in the numbers of beneficial bacteria (e.g. Bacteroides, Clostridiae, Lactobacillus, Campylobacter and Eubacterium spp.). Intestinal dysbiosis might influence the disease outcome. Hence, there is a need to characterize the complex parasite–bacterium relationships in the context of amoebic infection.
The trophozoites leave the intestinal lumen and invade the mucosa. Invasive amoebiasis results from the parasite- and tissue-driven processes in which physical, cellular and molecular determinants change as the motile trophozoites progress through the intestinal parenchyma. Acute amoebiasis can be viewed as resulting from the balance between the invasive trophozoites’ ‘fitness’ [i.e. their ability to: (i) express the phenotype required to resist host defences, such as oxidative and nitrosative stresses and (ii) rapidly adapt to changes in the environment] and the defensive response by the host's tissue (which controls the parasite burden, the degree of inflammation, the immune responses and the type of cell death). Moreover, combined activation of parasite and host lytic enzymes leads to the destruction of the intestinal parenchyma during the invasive process. The lytic enzymes degrade the mucus layer, the extracellular matrix (ECM) and the cell–cell junctions. The host's initial response is pro-inflammatory; the cellular component consists of neutrophil infiltration towards the sites of parasite invasion, followed by the activation of mast cells, macrophages and NK (natural killer) T cells. Inflammation is the main factor in tissue damage, and thus favours E. histolytica’s invasive progression. The major physiopathology features of interactions between E. histolytica and human cells (as studied with cell-based models in vitro and in mice in vivo) have been reviewed recently (Marie and Petri Jr, Reference Marie and Petri2014; Nakada-Tsukui and Nozaki, Reference Nakada-Tsukui and Nozaki2016).
In the present review, we describe the interactions between amoebic transcriptome responses and intestinal tissue responses. Most of the data are derived from our experiments on human colon explants. We shall focus on: (i) transcriptomic changes and genomic differences between commensal and pathogenic trophozoites; (ii) changes in phenotype in pathogenic amoebae during their interactions with the human colon; (iii) interplay between the local intestinal cells and the parasite during tissue invasion; and (iv) E. histolytica’s motility during invasion of the human colon.
The plasticity of E. histolytica's phenotype is involved in pathogenesis: genomic differences and transcriptomic changes
It is difficult to study the time course of tissue penetration, host-parasite interactions and the molecular mechanisms underlying human colon invasion by E. histolytica because the parasite is obligate in humans. This feature precludes laboratory experiments on the best model of infection. To circumvent this obstacle, we have established an ex vivo model of the adult human colon that mimics the early steps in intestinal amoebiasis (see below). By using human colon explants as an experimental system, we have addressed the key question of how commensal and pathogenic amoebae react in the human colon by comparing two strains of E. histolytica. The first strain is the pathogenic E. histolytica HM1:IMSS; it was isolated in Mexico from a patient suffering from amoebic dysentery, and is the strain most commonly used to reproduce the main features of intestinal and hepatic amoebiasis in disease models. The second strain is E. histolytica Rahman; this was isolated in the UK from an asymptomatic carrier and is conventionally referred to as a non-virulent strain. rRNA sequencing (Som et al. Reference Som, Azam, Bhattacharya and Bhattacharya2000) and genomic diversity studies (Shah et al. Reference Shah, MacFarlane, Bhattacharya, Matese, Demeter, Stroup and Singh2005) have confirmed that both strains belong to the E. histolytica species. In contrast to E. histolytica HM1:IMSS, the Rahman strain is attenuated in virulence. Its trophozoites are unable to: (i) exert significant levels of cytotoxic activity with epithelial cells in vitro (Ankri et al. Reference Ankri, Padilla-Vaca, Stolarsky, Koole, Katz and Mirelman1999); (ii) form liver abscesses in animal models (Dvoraka et al. Reference Dvoraka, Kobayashi, Nozaki, Takeuchi and Matsubara2003); (iii) invade a human intestinal xenograft model of amoebic colitis (Davis et al. Reference Davis, Zhang, Guo, Townsend and Stanley2006); and (iv) invade and destroy tissue in colon explants (Thibeaux et al. Reference Thibeaux, Weber, Hon, Dillies, Avé, Coppée, Labruyère and Guillén2013). In molecular terms, the Rahman strain also differs from the HM1:IMSS strain with regard to: (i) a truncated glycan chain in the proteophosphoglycan coating the surface (Moody et al. Reference Moody, Becker, Nuchamowitz and Mirelman1997), (ii) a lower level of peroxiredoxin (Davis et al. Reference Davis, Zhang, Guo, Townsend and Stanley2006); and (iii) a lower level of the light subunit of the galactose/N-acetyl-D-galactosamine (Gal-GalNAc) lectin (Ankri et al. Reference Ankri, Padilla-Vaca, Stolarsky, Koole, Katz and Mirelman1999).
Recent data obtained by high-throughput DNA sequencing indicate that the degree of genomic divergence between the E. histolytica Rahman and HM1:IMSS strains (calculated in a homologous single-nucleotide polymorphism analysis) is 0·38 – a value considered to be low (Weedall et al. Reference Weedall, Clark, Koldkjaer, Kay, Bruchhaus, Tannich, Paterson and Hall2012). Nevertheless, the presence of DNA fragment duplication in the Rahman strain's genome indicates that DNA recombination/reassortment may be important in Entamoeba species. The genes within the duplicated DNA fragment do not have an identified role in the loss of virulence in the Rahman strain. Gene expression analysis has provided insight into the molecular factors involved in the virulence of the HM1:IMSS strain, with major differences relative to the Rahman strain. To identify genes whose expression is correlated with a virulence-related phenotype, the strains’ transcriptomes were compared during in vitro culture and again during incubation with human colon explants (Thibeaux et al. Reference Thibeaux, Weber, Hon, Dillies, Avé, Coppée, Labruyère and Guillén2013). The differential analysis identified gene expressions that were either (i) specific to each strain in culture, (ii) common or (iii) specific to the two strains when exposed to human colon explants. A gene ontology enrichment analysis of each strain's gene-specific responses to contact with human colon explants highlighted a remarkable transcriptomic feature of the HM1:IMSS invasive phenotype: the upregulation of genes involved in carbohydrate metabolism and the processing of glycosylated residues (Thibeaux et al. Reference Thibeaux, Weber, Hon, Dillies, Avé, Coppée, Labruyère and Guillén2013). The expression levels of these genes do not change significantly in the Rahman strain when it comes into contact with human colon tissue. However, it can be argued that trophozoites from the two E. histolytica strains may harbour as-yet unknown genetic differences influencing virulence. Although the existence of epigenetic regulatory mechanisms in one or both strains cannot be ruled out, the genetic derivation is not the principal reason for pathogenic differences between these two Entamoeba isolates. Recently, we analyzed the HM1:IMSS strain after incubation in a range of environmental conditions influencing pathogenicity. This included long-term cultivation, during which the HM1:IMSS loses some virulence markers; the trophozoites have the same genetic background but have lost the ability to develop liver abscesses (Olivos et al. Reference Olivos, Ramos, Nequiz, Barba, Tello, Castañón, González, Martínez, Montfort and Pérez-tamayo2005). The cultured strain was compared with highly virulent HM1:IMSS amoeba in the presence and absence of human colon tissue (Weber et al. Reference Weber, Koutero, Dillies, Varet, Lopez-Camarillo, Coppée, Hon and Guillén2016). The transcriptome data were closely related to the gene responses identified when comparing the Rahman and HM1:IMSS strains in culture and during their interaction with the human colon. One can thus conclude that contact between virulent trophozoites and the human colon is associated with a unique gene transcription profile required for the development of an invasive phenotype. The ability to switch to a virulent phenotype has been lost in the Rahman strain. The genes found to be significantly modulated during the amoebic invasion of the human colon code for glycosylases, together with proteins involved in the cytoskeleton and DNA repair activities. Overall, these data indicate that a rapid shift in gene expression is the most important driver of pathogenicity during E. histolytica’s interaction with the human colon.
Interplay between the human colon and the parasite during tissue invasion
The colon is formed by four distinct tissue structures: the mucosa, the submucosa, the muscularis externa, and the adventitia (Fig. 1A). The mucosal epithelium displays deep invaginations, forming the crypts of Lieberkühn (Fig. 1B). Between the crypts, a layer of connective tissue (the lamina propria) is enriched in ECM components, fibroblasts, and immune cells. The submucosa is a highly vascularized, innervated connective tissue that links the mucosa to the muscularis mucosa. It comprises a vast network of neurons (forming Meissner's plexus), and lymphoid follicles containing T cells, B cells and IgA-secreting plasma cells. Entamoeba histolytica destroys the intestinal parenchyma (the first physical barrier) during its migration towards the blood vessels. By using wild-type E. histolytica or strains with various impairments in pathogenic functions, we have studied the trophozoite's invasion of the mucosa in a fresh human colon explant model that includes the tissue's key defensive components (mucus, epithelial cells, immune cells, fibroblasts and ECM elements).
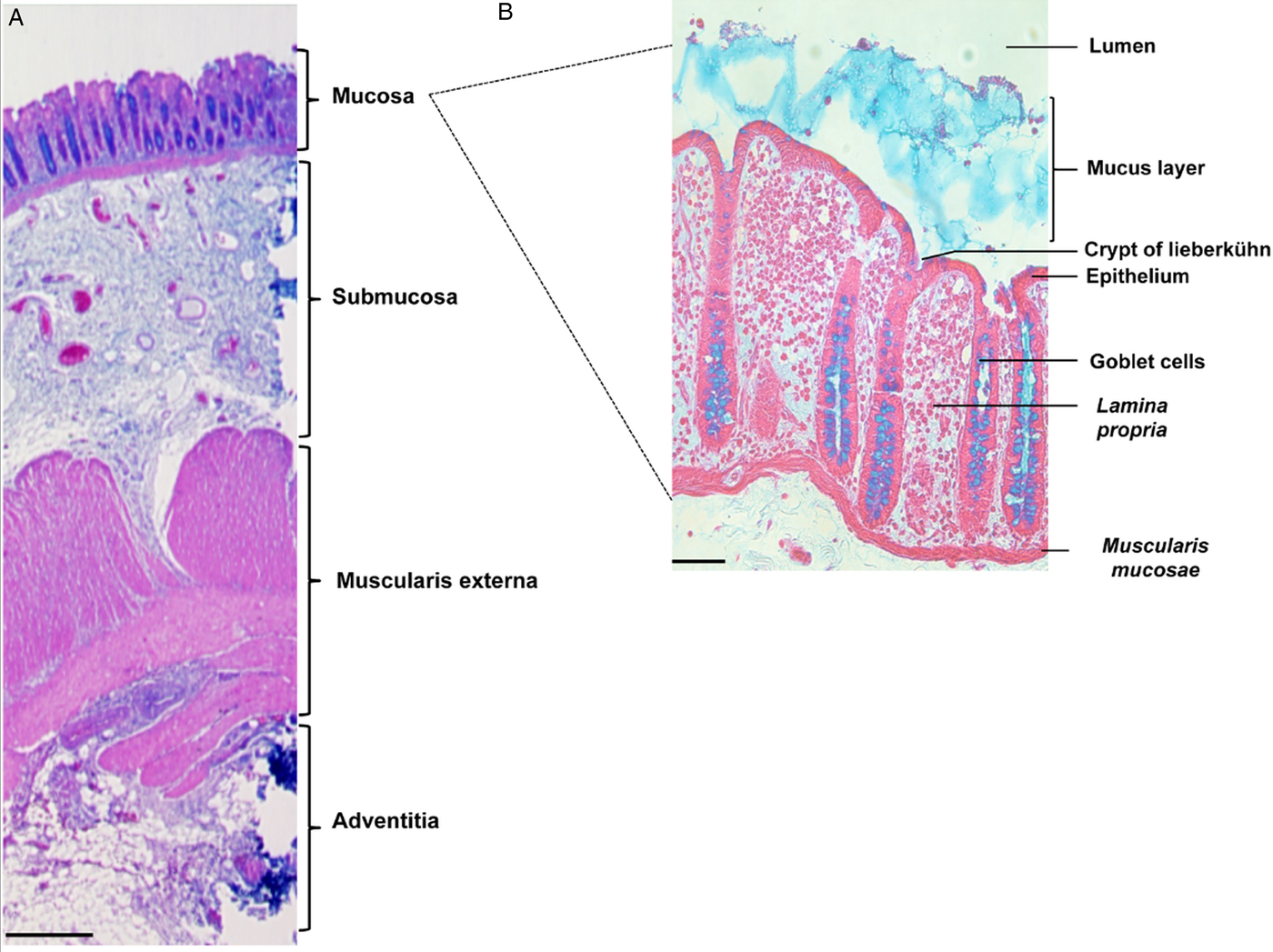
Fig. 1. Organization of tissue in the human colon. Histological staining of longitudinal sections of (A) the human colonic wall (scale bar: 500 µm) and (B) the human colonic mucosa (scale bar: 50 µm). The colonic mucosa is characterized by a monostratified epithelium composed of enterocytes and abundant goblet cells. It invaginates deep into the lamina propria, forming the crypts of Lieberkühn. Histological staining: haematoxylin (nucleus/purple), eosin (cytoplasm/pink) and Alcyan blue (intestinal mucus/blue).
Mucus gel layer adhesion and depletion
Upon activation of virulent trophozoites in the human colon, the dense, thick (up to 800 µm) mucus layer constitutes the first three-dimensional protective barrier encountered by E. histolytica. Mucus is a complex gel of glycolipids and mucins (notably MUC2, MUC5AC, MUC5B and MUC6). The mucins are high-molecular-mass glycoproteins decorated with sugar residues, such as N-acetylglucosamine, GalNAc, Gal, fucose, hexose and sialic acid. The mucus gel's polymer structure and viscoelastic properties are due to intermolecular and intramolecular interactions between secreted mucins (Johansson and Hansson, Reference Johansson and Hansson2016). In the first stage of colonization, E. histolytica adheres to the mucus layer. In asymptomatic infections, it is not known whether the trophozoites live and multiply at the surface of the mucus layer or whether they are embedded in loose mucus colonized by the microbiota. In the human colon explant model, both the Rahman and HM1:IMSS Entamoeba strains are able to adhere to the mucus layer (Thibeaux et al. Reference Thibeaux, Weber, Hon, Dillies, Avé, Coppée, Labruyère and Guillén2013). It is often stated that trophozoite adhesion to mucus is mediated by E. histolytica’s Gal/GalNAc lectin, which binds to the Gal and GalNAc residues present in the mucins’ glycosylated side chains. However, the experimental data mitigate this assumption, and suggest that other adhesive molecules are involved in amoeba-mucus adhesion. For instance, inhibition of the Gal/GalNAc lectin [in the RBV strain (Bracha et al. Reference Bracha, Nuchamowitz, Anbar and Mirelman2006; Reference Bracha, Nuchamowitz, Wender and Mirelman2007)] or the blockade of lectin signalling [in the HGL-2 strain (Tavares et al. Reference Tavares, Rigothier, Khun, Roux, Huerre and Guillen2005)] does not abolish adhesion (Bansal et al. Reference Bansal, Ave, Kerneis, Frileux, Boché, Baglin, Dubost, Leguern, Prevost, Bracha, Mirelman, Guillèn and Labruyère2009) or destruction of the mucus layer. Depletion of the mucus layer in the human colon explant model is only observed in the presence of the virulent E. histolytica HM1:IMSS strain (Fig. 2A–C), which depletes both the loose mucus sublayer and dense mucus sublayer that firmly adheres to the epithelium and prevents bacterial colonization. Entamoeba histolytica has at least two strategies for removing the mucus layer: its secretagogue activity and its ability to cleave the mucin polymer network. It has long been known that E. histolytica exacerbates mucus secretion (Chadee et al. Reference Chadee, Keller, Forstner, Innes and Ravdin1991), which might exhaust the mucin-producing goblet cells. It was recently shown that the E. histolytica cysteine proteinase A5 (CP-A5) binds to goblet cell αvβ3 receptors, initiates a signal cascade, and thus induces mucin secretion by goblet cells (Cornick et al. Reference Cornick, Moreau and Chadee2016). Entamoeba histolytica’s glycosidase and cysteine protease (CP) activities are essential for destabilization of the mucin gel. The non-virulent E. histolytica Rahman strain is unable to deplete the protective mucus layer (Bansal et al. Reference Bansal, Ave, Kerneis, Frileux, Boché, Baglin, Dubost, Leguern, Prevost, Bracha, Mirelman, Guillèn and Labruyère2009; Thibeaux et al. Reference Thibeaux, Dufour, Roux, Bernier, Baglin, Frileux, Olivo-marin, Guillén and Labruyère2012). Given that the HM1:IMSS and Rahman strains differ markedly in their expression of CPs (Davis et al. Reference Davis, Schulze and Stanley2007), it has been suggested that the CPs are involved in degradation of the mucus layer. Consistently, in vitro studies have demonstrated that E. histolytica CPs can cleave the C-terminal end of MUC2 (Lidell et al. Reference Lidell, Moncada, Chadee and Hansson2006). In the ex vivo colon explant model, inhibition of CP activities (using the irreversible inhibitor E-64) abolishes mucus layer degradation (Thibeaux et al. Reference Thibeaux, Dufour, Roux, Bernier, Baglin, Frileux, Olivo-marin, Guillén and Labruyère2012). However, CP-A5's activity is not essential because trophozoites lacking this protease (the RB8 strain (Bracha et al. Reference Bracha, Nuchamowitz, Anbar and Mirelman2006)) are able to deplete the mucus layer. Hence, other CPs are probably involved in mucus depletion (Bansal et al. Reference Bansal, Ave, Kerneis, Frileux, Boché, Baglin, Dubost, Leguern, Prevost, Bracha, Mirelman, Guillèn and Labruyère2009).

Fig. 2. Invasion and depletion of the protective mucus layer. (A) Entamoeba histolytica adheres to and invades the mucus layer. Histological staining of a longitudinal section of the human colonic mucosa after an hour of incubation with E. histolytica. Histological staining: haematoxylin (nucleus/purple), eosin (cytoplasm/pink) and Alcyan blue (intestinal mucus/blue). Scale bar: 20 µm. (B–D) Scanning electron micrographs of the luminal surface of human colon explants incubated with E. histolytica. (B) Trophozoites adhering to the mucus layer (time 0); (C) A breach in the mucus layer by E. histolytica reveals the colonic epithelium (after 1 h of incubation); (D) The trophozoites have removed the mucus layer, and the normal mucosal architecture of the colonic epithelium (with the mucus-secreting crypts of Lieberkühn) are visible after 2 h of incubation. Note the specific localization of cell aggregates composed of E. histolytica and (presumably) dead human epithelial cells and immune cells in the interglandular regions. Scale bar: 20 µm.
As mentioned above, MUC2's extensively glycosylation enables it to resists some proteases. Thus, cleavage of the MUC2 protein backbone may require prior glycosidase activity and removal of the carbohydrate side chains. In vitro, it has been shown that E. histolytica possesses glycosidase activities that might degrade oligosaccharides on colonic mucins (Moncada et al. Reference Moncada, Keller and Chadee2005). This ability to cleave MUC2 is in line with the results of transcriptomic comparisons (Thibeaux et al. Reference Thibeaux, Weber, Hon, Dillies, Avé, Coppée, Labruyère and Guillén2013) of HM1:IMSS and Rahman strains during incubation on human colon explants. For instance, the genes encoding a glycoside hydrolase (a β-amylase absent in humans) is strongly expressed by the HM1:IMSS strain but not by the Rahman strain (Fig. 3) Consequently, inhibition gene expression (using RNA interference) in the virulent HM1:IMSS strain abolishes mucus depletion and tissue invasion (Thibeaux et al. Reference Thibeaux, Weber, Hon, Dillies, Avé, Coppée, Labruyère and Guillén2013). Therefore, the pathogenicity of E. histolytica appears to be regulated by the ability to cleave and use sugar moieties from colon components like the MUC2 oligosaccharide chains. These results indicate that E. histolytica HM1:IMSS may use glycosidase activities to remove mucin oligosaccharides and expose the protein backbone to CPs.
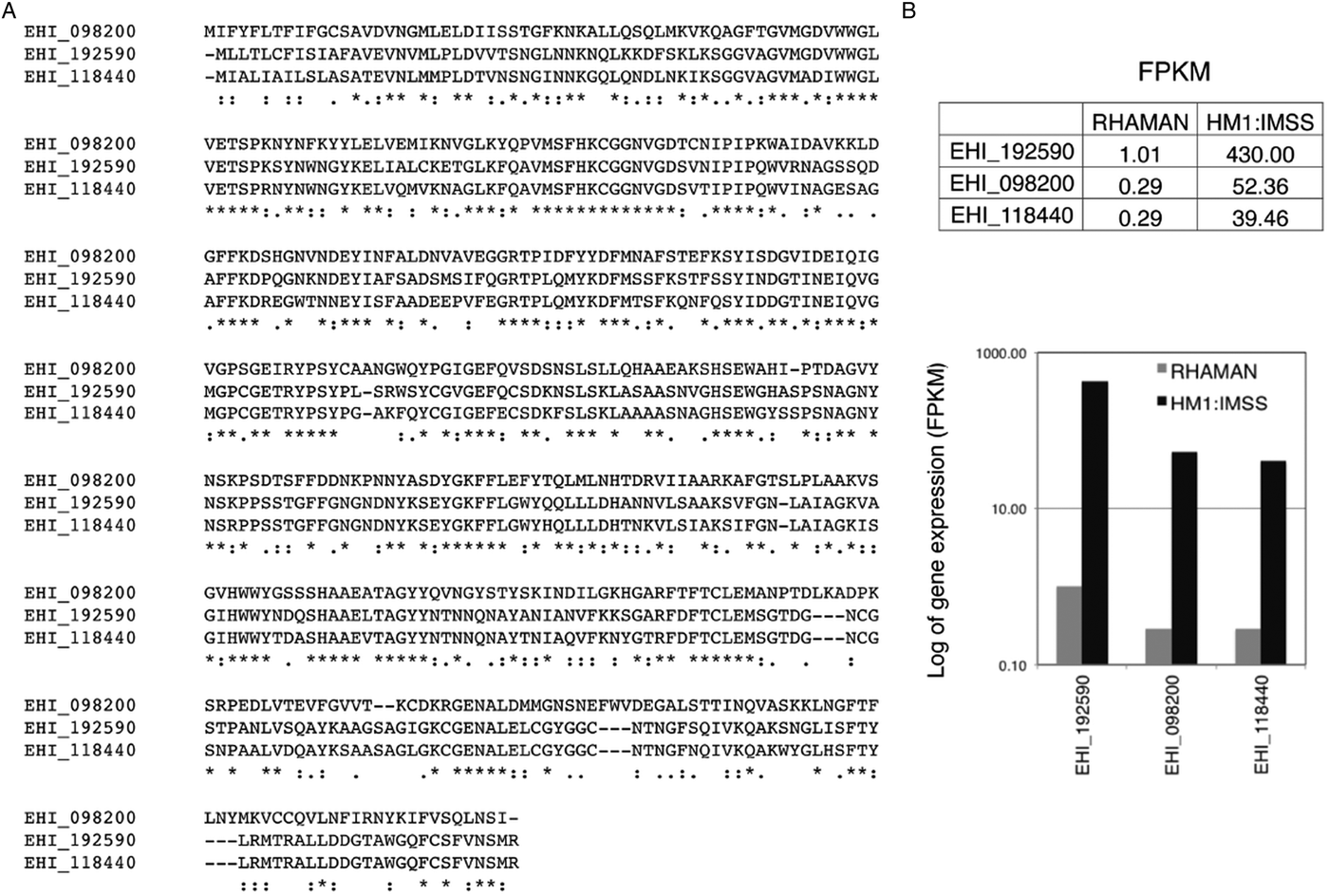
Fig. 3. Transcription of the gene coding for β-amylase in E. histolytica HM1:IMSS and Rahman strains. (A) A bioinformatics analysis of the amino acid sequences of β-amylase isoforms (accession numbers are taken from AmoebaDB) was performed using the CLUSTALW computer program. The results indicate identity (stars) and homology (double dots) between the proteins. (B) Counted reads for expression of the gene coding for β-amylase after RNA sequencing of HM1:IMSS or Rahman transcripts. Note the low number of reads in Rahman trophozoites and the high number in HM1:IMSS trophozoites. FPKM: fragments (reads) per kilobase of transcript per million fragments mapped.
Destruction of the colonic epithelium
Once E. histolytica has disrupted the protective mucus layer, trophozoites adhere to the colonic epithelium (mainly in the interglandular regions) (Fig. 2D). This specific location might be due to the presence of shed apoptotic cells. The latter might constitute an important source of nutrients for the amoeba, since it has been clearly shown that E. histolytica preferentially phagocytose apoptotic corpses in vitro (Huston et al. Reference Huston, Boettner, Miller-sims and Petri2003). The trophozoites’ location might also be explained by the decrease in mechanical tension between epithelial cells caused by shedding; this creates a weak point in the epithelium barrier, and might facilitate entry by E. histolytica. It was recently shown that after attaching to host cells, amoebae ‘chew off’ and ingest host cell fragments via trogocytosis (Ralston et al. Reference Ralston, Solga, Mackey-Lawrence, Somlata and Petri2014), which contributes to cell killing. After 4 h of incubation, virulent E. histolytica trophozoites lyse human cells and prompt a significant increase in local levels of lactate dehydrogenase (Bansal et al. Reference Bansal, Ave, Kerneis, Frileux, Boché, Baglin, Dubost, Leguern, Prevost, Bracha, Mirelman, Guillèn and Labruyère2009). The uptake of cells and nuclei from eukaryotic cells by E. histolytica can be seen under the microscope, highlighting active phagocytic activity during the removal of the epithelium (Figs 4 and 5A). Destruction of the epithelium architecture requires CP activity, since inhibition of these enzymes abolishes epithelial cell detachment; this observation is consistent with previous studies showing that CPs can alter tight junctions (Leroy et al. Reference Leroy, Lauwaet, De Bruyne, Cornelissen and Mareel2000; Betanzos et al. Reference Betanzos, Javier-Reyna, Garcia-Rivera, Banuelos, Gonzalez-Mariscal, Schnoor and Orozco2013). The mechanism of cell death is difficult to characterize in vivo; death may occur following parasite-cell contact, changes in homeostasis rupture and intestinal inflammation because K+ channels are host mediators of (i) amoebic cytotoxicity in many cell types and (ii) inflammasome activation in macrophages (Marie et al. Reference Marie, Verkerke, Theodorescu and Petri2015). Nevertheless, one can assume that epithelial cell apoptosis promotes intestinal barrier leakage and thus facilitates invasion. Amoebapores are pathogenic factors known to be involved in epithelial cell lysis (Horstmann et al. Reference Horstmann, Leippe and Egbert1992; Andra et al. Reference Andra, Herbst and Leippe2003). The pathogenicity of the G3 strain [which lacks amoebapore-A (Bracha et al. Reference Bracha, Nuchamowitz and Mirelman2003)] has been investigated in human colon explants. The results show that the G3 strain behaves in much the same manner as the parental HM1:IMSS strain: both strains induce cell lysis and cytokine secretion to the same extent, and both are able to invade the colonic mucosa. This finding suggests that (i) amoebapore-A is not necessary for cell lysis and (ii) other molecules may be involved (Bansal et al. Reference Bansal, Ave, Kerneis, Frileux, Boché, Baglin, Dubost, Leguern, Prevost, Bracha, Mirelman, Guillèn and Labruyère2009). Several surface molecules – including LPPG (lipopeptidophosphoglycan), lysine and glutamic acid-rich protein 1 (KERP1), and STIRP (serine-, threonine-, and isoleucine-rich protein) – are reportedly involved in parasite–cell interactions (Marinets et al. Reference Marinets, Zhang, Guillén, Gounon, Bohle, Vollmann, Scheiner, Wiedermann, Stanley and Duchêne1997; MacFarlane and Singh, Reference MacFarlane and Singh2007; Santi-Rocca et al. Reference Santi-Rocca, Weber, Guigon, Sismeiro, Coppée and Guillén2008). Although these proteins’ functions have not been yet examined in vivo, the cell lysis process is likely to be multifactorial and regulated by more than one parasite determinant.

Fig. 4. Destruction of the human colonic mucosa. (A) An orthogonal section of the human colonic mucosa, visualized by multiphoton microscopy (MPM) and second harmonic generation (SHG) signal. After 4 h of culture in the absence of E. histolytica, intact epithelial cells are still at the mucosal surface and along the crypt of Lieberkühn (1); in the presence of E. histolytica, however, the epithelial cells have been detached, and the trophozoites adhere to the dense subepithelial collagen structures (2). (B) Histological analysis of longitudinal sections of the human colonic mucosa; after 4 h of incubation, trophozoites adhere to and detach the epithelial cells, and thus destroy the epithelium (1); nuclei and fragments of human cells are engulfed by trophozoites, which show strong phagocytic activity at this stage (2). Histological staining (3) and MPM/SHG imaging (4) of a human colon explant fixed after 7 h of incubation. Trophozoites have migrated along the crypts of Lieberkühn and deep into the mucosa. Note the marked removal of epithelial cells during trophozoite migration. Scale bar: 20 µm.

Fig. 5. Invasion of the colonic mucosa, and remodelling of its collagen structures. (A) A crypt of Lieberkühn in the epithelium. After 7 h of incubation, the control tissue has intact, cohesive epithelial cells (1). However, in the presence of E. histolytica (2), the epithelial cells are detached and replaced by trophozoites adhering to the ECM. Transversal section of fixed tissues, stained with DAPI (blue nuclei) and a monoclonal antibody against actin (red) (1, 2). Using live imaging, we observed the formation of phagocytic cups – showing that phagocytic activity occurs when trophozoites migrate along the crypts (3) Scale bar: 10 µm. (B) Destruction of the loose collagen fibre network during E. histolytica invasion. After 7 h of incubation, human colon explants were observed in real-time using MPM/SHG. In the absence of E. histolytica, the architecture of the control tissue shows an intact meshwork of collagen fibres (1) and round crypts surrounded by a collagen ring (2). In the presence of E. histolytica, the architecture of the loose collagen meshwork is disrupted; the crypts have collapsed (3), and the collagen structures surrounding the crypt have been remodelled (4) Scale bar: 20 µm. (C) A three-dimensional reconstruction of a human colon explant invaded by E. histolytica. Note the presence of trophozoites along the crypts and in the lamina propria, and the drastic alteration of the collagen structures. Scale bar: 50 µm.
Chemokine release during intestinal invasion
The inflammatory response and cytokine release following host cell–parasite interaction have been observed in vitro with cultured cell monolayers, in vivo in several animal models and, most recently, in patients infected with E. histolytica [for a review, see (Nakada-Tsukui and Nozaki, Reference Nakada-Tsukui and Nozaki2016)]. There is a growing body of evidence to show that the parasite's presence evokes an immune response and the secretion of pro-inflammatory mediators by intestinal epithelial cells. Histological analysis of human colonic biopsies has revealed that neutrophils, macrophages and dendritic cells infiltrate the mucosa to some extent at the start of the ulceration process. The neutrophil, plasma cell, eosinophil, macrophage and T cell counts rise as the infection progresses (Ventura-Juarez et al. Reference Ventura-Juarez, Barba-Gallardo, Munoz-Fernandez, Martinez-Medina, Marquez-Diaz, Sosa-Diaz, Gerardo-Rodriguez, Gonzalez-Romo and Campos-Rodriguez2007). Human colon tissue is known to contain resident immune cells. As expected, colon explants contain monocytes and T-lymphocytes, which contribute to tissue inflammatory responses during intestinal amoebiasis. Measurements of the interleukins IL-1β, IL-6, IL-8, interferon gamma (IFN-γ) and tumour necrosis factor (TNF) concentrations in the extracellular medium indicate that significant amounts of these chemokines are specifically secreted by human cells in the presence of E. histolytica (Bansal et al. Reference Bansal, Ave, Kerneis, Frileux, Boché, Baglin, Dubost, Leguern, Prevost, Bracha, Mirelman, Guillèn and Labruyère2009). These results are in agreement with previous findings in the SCID mouse-human intestinal xenograft (SCID-HU-INT) model, in which IL-1β, TNF and IL8 are produced in response to the introduction of E. histolytica trophozoites into the engrafted human intestinal segments (Zhang et al. Reference Zhang, Mahajan, Zhang and Stanley2003). Other cytokines (such as IL-2, IL-4 and IL-10) are not detected in the environment of human colon explants, regardless of the presence or absence of parasites. The high levels of pro-inflammatory cytokine release induced by E. histolytica demonstrate that our ex vivo human colon model reproduces the inflammatory responses to early-stage amoebiasis. The immune explants’ responses are initially induced by amoebic virulence factors (notably CPs). For instance, a strain lacking CP-A5 is able to deplete the mucus barrier but not to migrate within the mucosa or evoke an inflammatory response after 7 h of incubation (Bansal et al. Reference Bansal, Ave, Kerneis, Frileux, Boché, Baglin, Dubost, Leguern, Prevost, Bracha, Mirelman, Guillèn and Labruyère2009). In vitro experiments have shown that the binding of the pro-mature CP-A5 RGD motif to the α Vβ 3 integrin triggers integrin/NF-κB signalling, and elicits the production of pro-inflammatory cytokines (Hou et al. Reference Hou, Mortimer and Chadee2010). Taken as a whole, these results suggest that CP-A5 has an essential role in the inflammatory responses to trophozoite invasion. The molecular mechanism underlies this activity being investigated intensively.
Remodelling of the interstitial ECM, and invasion of the lamina propria
Thanks to technical progress in imaging living tissues and cells, combined multiphoton microscopy (MPM) and second harmonic generation (SHG) signal detection has become a powerful method for the real-time visualization of endogenous fluorescence and fibrillar collagen organization in living tissues (Schenke-Layland, Reference Schenke-Layland2008). By applying MPM/SHG to human explants, we characterized the three-dimensional architecture of the fibrillar collagen present in the human colon. We placed particular emphasis on the remodelling of the collagen scaffold that occurs during the intestinal invasion by the HM1:IMSS strain (Figs 4 and 5). Importantly, we found that the human colonic mucosa exhibits two different collagen fibre structures (Thibeaux et al. Reference Thibeaux, Dufour, Roux, Bernier, Baglin, Frileux, Olivo-marin, Guillén and Labruyère2012). Firstly, the subepithelial collagen layer (5 µm thick, located underneath the epithelium's basal membrane) is dense, and shows small apertures (of around 4 to 10 µm in diameter). Secondly, a loose meshwork of collagen fibres deeper within the lamina propria has large pores (up to 500 µm in diameter) between the bundles. Most of these large pores are full of cells (Figs 4 and 5). Entamoeba histolytica migrates along the dense collagen layer (without degrading it) and then along the crypt, where it is able to degrade the loose collagen meshwork and thus reach the lamina propria. Upon invasion by virulent trophozoites, the loose meshwork of collagen fibres is dispersed, and wavy bundles of collagen that have collapsed are seen. The collagen bundles are also altered in the lamina propria, and a structured collagen network is no longer apparent (Fig. 5B and C). These results indicate that collagen fibres are cleaved during E. histolytica’s invasion of human colonic tissue.
In contrast, a CP-A5-deficient strain does not alter the collagen structure or penetrate into the crypts. Importantly, dissemination of trophozoites into the lamina propria is only observed when the collagen network is altered -demonstrating that intestinal invasion requires disruption of the physical constraints exerted by the ECM. In addition to CP-A5's role in inflammation, previous research results suggest that CP-A5 is also involved in ECM degradation during tissue invasion (Bansal et al. Reference Bansal, Ave, Kerneis, Frileux, Boché, Baglin, Dubost, Leguern, Prevost, Bracha, Mirelman, Guillèn and Labruyère2009). In vitro, CP-A5 is seen to possess gelatinase activity (i.e. cleavage of non-fibrillar collagen) (Hellberg et al. Reference Hellberg, Nowak, Leippe, Tannich and Bruchhaus2002). Hence, it has been suggested that the CP-A5-deficient strain's failure to invade the tissue is due to its inability to overcome the physical constraints imposed by the ECM (Thibeaux et al. Reference Thibeaux, Dufour, Roux, Bernier, Baglin, Frileux, Olivo-marin, Guillén and Labruyère2012). In vitro experiments have investigated the level of collagenase activity (i.e. cleavage of fibrillar collagen) in the CP-A5 deficient strain; it was found to cleave collagen fibres to the same extent as the wild-type strain (Thibeaux et al. Reference Thibeaux, Dufour, Roux, Bernier, Baglin, Frileux, Olivo-marin, Guillén and Labruyère2012). Thus, the absence of collagen remodelling in situ appears to be due to the absence of the protein (including the pro-form), rather than a lack of collagenase activity for the expressed protein. These findings suggest that the absence of collagen remodelling upon tissue invasion by the CP-A5 deficient strain is probably due to the absence of inflammation; hence, the interplay between CP-A5 and human components is important for tissue destruction. The matrix metalloproteases (MMPs) are key human components in ECM degradation. Several studies have shown that inflammatory stimuli caused enterocytes to express higher levels of MMP-9 (Gan et al. Reference Gan, Wong, Wright, Cai and Al2001), which contributed to intestinal damage in a mouse model (Castaneda et al. Reference Castaneda, Walia, Vijay-Kumar, Patel, Roser, Kolachala, Rojas, Wang, Oprea, Garg, Gewirtz, Roman, Merlin and Sitaraman2005). Furthermore, the genes encoding MMP-1 and -3 are overexpressed in colon biopsies from patients with acute E. histolytica colitis (Peterson et al. Reference Peterson, Guo, Elkahloun, Mondal, Bardhan, Sugawara, Duggal, Haque and Petri2011). The role of MMP-1 and MMP-3 in the invasive process and CP-A5's role in the activation of human MMPs have been studied using ex vivo colon models and in vitro experiments. Briefly, in the presence of E. histolytica trophozoites: (i) MMPs-1 and -3 are overexpressed, (ii) inhibition of the MMPs’ proteolytic activity prevents trophozoite invasion and ECM remodelling, and (iii) CP-A5 cleaves pro-MMP-3, which in turn activates pro-MMP-1 (Thibeaux et al. Reference Thibeaux, Avé, Bernier, Morcelet, Frileux, Guillén and Labruyère2014). CP5's dual function contributes to remodelling of the ECM by activating the host's early-stage inflammatory response and by stimulating human MMPs.
Entamoeba histolytica's motility, cytokine chemotaxis and tissue invasion
For successful intestinal invasion, E. histolytica has to be motile. This motility seems to be activated by compounds involved in the inflammatory response. Cell migration within a tissue is usually triggered and/or guided by environmental stimuli (concentration gradient of chemokines, nutrients or growth factors), and the resulting changes in cell morphology depend on the forces on the cell and within the cell (Doyle et al. Reference Doyle, Carvajal, Jin, Matsumoto and Yamada2015). The composition of a three-dimensional environment (i.e. specific signalling molecules and mechanical forces) critically affects cell behaviour, and leads to phenotypic diversity by stimulating variations in gene expression and responsiveness to chemical perturbations and changes in the microenvironment. The establishment of amoebiasis is overwhelmingly dependant on E. histolytica’s behaviour in the human colon, where environmental factors trigger changes in the amoebic phenotype, migration, tissue remodelling and tissue destruction. In the human colon, the components that trigger E. histolytica’s switch from a non-pathogenic commensal to an invasive pathogen have not yet been identified. Nevertheless, several molecules capable of attracting trophozoites in vitro have been described: complement component 5a, fibronectin, IL8, TNF and unidentified chemoattractants from E. coli or erythrocyte extracts (Urban et al. Reference Urban, Jarstrand and Aust-Kettis1983; Franco et al. Reference Franco, Vazquez-Prado and Meza1997; Blazquez et al. Reference Blazquez, Zimmer, Guigon, Olivo-Marin, Guillén and Labruyère2006; Diaz-valencia et al. Reference Diaz-valencia, Pérez-yépez, Ayala-sumuano, Franco and Meza2015). The random migration of E. histolytica follows a typical amoeboid mechanism. A large number of blebs nucleate, with one becoming the predominant pseudopod for forwarding movement based on actin/myosin II contraction (Dufour et al. Reference Dufour, Olivo-marin and Guillen2015; Aguilar-Rojas et al. Reference Aguilar-Rojas, Olivo-Marin and Guillen2016). During chemotactic migration towards TNF, E. histolytica’s velocity increases; this is accompanied by changes in actin cytoskeleton dynamics and in the trophozoite's shape (with little or no blebbing and a persistent, large migration front). The phosphatidylinositide 3-kinases (Pi3Ks) are enzymes involved in a large range of cellular functions including cell-motility. In E. histolytica, the phosphatidylinositol -3 -4,5- triphosphate (a product of Pi3K activity that localizes to rafts lipids domains) is expected to participate in the control amoeba motility (Koushik et al. Reference Koushik, Powell and Temesvari2013). As shown by experiments with the Pi3K inhibitor wortmannin, PI3 kinase is involved in directional sensing and persistent pseudopod extension in mechanotaxis (Rivière et al. Reference Rivière, Marion, Guillen, Bacri, Gazeau and Wilhelm2007), chemotaxis towards TNF (Blazquez et al. Reference Blazquez, Guigon, Weber, Syan, Sismeiro, Coppée, Labruyère and Guillén2008) and the presence of LPA (known to stimulate chemotaxis towards nutrients) (Franco-Barraza et al. Reference Franco-Barraza, Zamudio-Meza, Franco, Domínguez-Robles, Villegas-Sepulveda and Meza2006; Bharadwaj et al. Reference Bharadwaj, Arya, Mansuri, Bhattacharya and Bhattacharya2017). Interestingly, the presence of wortmannin does not modify cell polarization during random locomotion. During a chemotactic response to TNF and IL8, specific rearrangements of actin-myosin cytoskeleton components and regulatory elements are observed (Blazquez et al. Reference Blazquez, Guigon, Weber, Syan, Sismeiro, Coppée, Labruyère and Guillén2008; Diaz-valencia et al. Reference Diaz-valencia, Pérez-yépez, Ayala-sumuano, Franco and Meza2015). Furthermore, our transcriptomic study during TNF chemotaxis showed that genes involved in actin cytoskeleton dynamics are upregulated; these include genes encoding proteins attributed to microfilament dynamics, network and bundling (such as components of the Arp2-3 complex, formins, ABP-120, cortexillin and α-actinin) and proteins required for microfilament functions (such as myosin IB and II, and coronin and signalling proteins). The genes encoding the light subunit 1 (Lgl1) and a heavy subunit (Hgl) of the Gal/GalNAc lectin are also upregulated. Consequently, E. histolytica with defective Gal/GalNAc lectin signalling (HGL2) are able to migration randomly but have impaired chemotaxis in vitro towards TNF and C5a; this observation suggests that the Gal/GalNAc lectin has a role in transducing traction forces during chemotaxis (Blazquez et al. Reference Blazquez, Guigon, Weber, Syan, Sismeiro, Coppée, Labruyère and Guillén2008). The molecular mechanisms of E. histolytica chemotaxis and the chemoattractant molecules’ receptors have not been fully characterized. Nevertheless, experiments with specific antibodies and a BLAST analysis have shown that amoeba possesses a 29 kDa protein that shares epitopes with the human-IL8 receptor and a 140 kDa cell surface protein (CSP) from the Bsp-A family (related to the human TNF receptor). These proteins might bind IL8 (Diaz-valencia et al. Reference Diaz-valencia, Pérez-yépez, Ayala-sumuano, Franco and Meza2015) and TNF (Silvestre et al. Reference Silvestre, Plaze, Berthon, Thibeaux, Guillen and Labruyère2015), respectively. CSP colocalizes with TNF on the parasite surface, and is recruited to the uropode during TNF chemotaxis. Experiments on trophozoites lacking CSP (using antisense RNA) have highlighted the role of this protein in TNF chemotaxis. Indeed, these trophozoites are able to migrate at random but not chemotactically in a TNF gradient (Silvestre et al. Reference Silvestre, Plaze, Berthon, Thibeaux, Guillen and Labruyère2015). Taken as a whole, these data suggest that E. histolytica chemotaxis towards TNF is dependent on: (i) CSP (which senses a TNF gradient), (ii) PI3K (involved in persistent polarization), (iii) the Gal–Gal NAc lectin (for signal transduction) and (iv) actin–myosin cytoskeleton components (the rearrangement of which changes the amoeba's dynamics and shape).
However, one can question whether the inflammatory molecule TNF has a direct influence on parasite migration in vivo. In the human colon explant model, trophozoites lacking CSP at their surface are unable to invade the lamina propria but are still able to deplete the mucus, and detach and lyse endothelial cells. Therefore, E. histolytica’s sensing of TNF seems to be critical for the invasive process in the early-stage of amoebiasis – suggesting that a TNF gradient attracts amoeba and prompts them to penetrate into the interstitial ECM. Experiments in the SCID-HU-INT mouse model have shown that TNF blockage reduces intestinal damage in amoebic infection (Zhang et al. Reference Zhang, Mahajan, Zhang and Stanley2003), whereas a study in Bangladeshi children revealed an association between higher TNF production and E. histolytica diarrhoea (Peterson et al. Reference Peterson, Shu, Duggal, Haque, Mondal and Petri2010). Taken as a whole, these data suggest that the host's inflammatory response is essential for parasite migration through the lamina propria and thus the development of the disease.
Our knowledge of how amoebae move within the colonic tissue (i.e. in live three-dimensional models) is still very limited, and we now need to design and implement a realistic experimental model that is amenable to all the molecular and cellular approaches used to investigate E. histolytica’s strategy for migration within the human intestinal mucosa. The ability of the human ‘gut-on-a-chip’ to reproduce the three-dimensional structures, differentiated cell types, and multiple physiological functions of the normal human intestine (Kim et al. Reference Kim, Li, Collins and Ingber2015) may provide a powerful alternative to in vitro colon explants. This model may enable characterization of the dynamic spatial and temporal interplay between E. histolytica factors and the human ECM (cells, molecules, and physical constraints) during the invasive process.
Concluding remarks
In our working model, we have considered several factors that may explain how the commensal amoebic phenotype shifts towards virulence. The first factor may be a change in the composition of the bacterial flora; this might take place at any time. For instance, it is known that co-infection in the presence of certain Enterobacteriaceae increases the parasite's virulence. Another likely factor is the human immune response, since the E. histolytica amoebae are chemoattracted by pro-inflammatory molecules like TNF. Some intestinal cells behave like TNF reservoirs and may be activated in the early stages of infection. Binding of TNF at the amoebic surface induces motility through a chemotactic signalling mechanism. The differences in pathogenicity between commensal and virulent trophozoites appear to be closely related to the commensal parasites’ ability to: (i) rapidly switch gene expression and (ii) activate enzymes needed for mucus component degradation and lytic factors that in turn exacerbate the immune response. The MMPs and CP-A5 are key lytic enzymes and immunomodulators in both parasite invasion and tissue inflammation. Hence, MMP-3 and/or CP-A5 inhibitors may be of value in studies of how to treat intestinal amoebiasis.
Acknowledgements
We acknowledge the contribution of the Institut Pasteur's Transcriptome and EpiGenome Facility and Imagopole to data analysis and microscopy images. We thank the DIM Ile-de-France program for support us in the acquisition of the two-photon microscope.
Financial support
This work was funded by the Institut Pasteur (through grants PTR-178 and PTR 392), the French National Agency for Research (ANR, through grants ANR-MIE-08 Intestinalamibe and ANR-2010-GENM-0011-01, GENAMIBE), and the French Parasitology consortium Labex ParaFrap (through the grant ANR-11-LABX0024).