Toxocariasis and food safety
Food safety is a major public health issue worldwide, and it is vitally important that any risks to consumers are managed both to protect the population and maintain consumer confidence. One such risk is the food-borne transmission of parasites to humans, which can have severe health implications for the global population. The most recently published assessment of the burden of food-borne parasites to humans estimated that 6.64 million disability-adjusted life years were lost due to the consumption of contaminated food in 2010 (Torgerson et al., Reference Torgerson, Devleesschauwer, Praet, Speybroeck, Willingham, Kasuga, Rokni, Zhou, Fèvre, Sripa, Gargouri, Fürst, Budke, Carabin, Kirk, Angulo, Havelaar, de Silva and Sripa2015). Human toxocariasis affects an estimated 1.4 billion people worldwide (Ma et al., Reference Ma, Rostami, Wang, Hofmann, Hotez and Gasser2020). The disease is caused by Toxocara canis and T. cati, common roundworm parasites of canines and felines, respectively. Toxocara vitulorum affects bovine species and is generally believed to have less zoonotic significance, although knowledge of its transmission biology is currently lacking (Dewair and Bessat, Reference Dewair and Bessat2020).
Human toxocariasis is recognized as one of the most commonly reported zoonotic helminth diseases worldwide (Magnaval et al., Reference Magnaval, Glickman, Dorchies and Morassin2001; Nicoletti, Reference Nicoletti2013), having a significant impact on global public health (Zibaei and Sadjjadi, Reference Zibaei and Sadjjadi2017). Toxocariasis can lead to a number of different clinical manifestations in humans, including allergic, ophthalmic and neurological disorders (Ma et al., Reference Ma, Holland, Wang, Hofmann, Fan, Maizels, Hotez and Gasser2018). Recent epidemiological studies and meta-analyses on cognitive impairment, psychosis and epilepsy have associated Toxocara infection with these debilitating neurological diseases (Walsh and Haseeb, Reference Walsh and Haseeb2012; Luna et al., Reference Luna, Cicero, Rateau, Quattrocchi, Marin, Bruno, Dalmay, Druet-Cabanac, Nicoletti and Preux2018; Taghipour et al., Reference Taghipour, Habibpour, Mirzapour and Rostami2021), and a potential link to the development of degenerative conditions such as Alzheimer's disease has been hypothesized (Fan, Reference Fan2020). Despite recognition of its clinical impact, toxocariasis remains a neglected disease and major gaps in our understanding of the epidemiology of this parasite remain.
Large numbers of Toxocara eggs are excreted in the feces of infected dogs, cats and foxes (Morgan et al., Reference Morgan, Azam and Pegler2013). Once present in the environment, these eggs can develop to the infective stage and persist in the soil for long periods (Fan et al., Reference Fan, Liao and Cheng2013). If infective eggs are consumed by accidental or paratenic host species, including humans, the larvae that hatch out in the gut subsequently migrate to several different organs in the body, becoming encapsulated in the tissues where larval development ceases. Studies have suggested it is primarily the host's immune response to Toxocara which is responsible for much of the pathology seen in cases of toxocariasis, rather than mechanical damage caused by migrating larvae (Epe et al., Reference Epe, Sabel, Schnieder and Stoye1994). In cases of ocular infection, the response to a single larva can lead to vision loss (Neafie and Connor, Reference Neafie, Connor, Binford and Connor1976).
Whilst it is widely accepted that contaminated soil acts as a source of Toxocara eggs for human infections with potentially severe clinical consequences, little is known about food-borne transmission of this parasite. With eggs contaminating grazing pastures and growing crops, the pathway to food contamination appears wide open. However, this transmission route has received relatively little attention in the literature. Toxocara larvae are able to persist in the tissues of paratenic hosts, thus infected meat can act as a source of infection to humans (Strube et al., Reference Strube, Heuer and Janecek2013; Wu and Bowman, Reference Wu and Bowman2020), particularly if consumed undercooked (Fan et al., Reference Fan, Holland, Loxton and Barghouth2015). A recently published review by Bowman (Reference Bowman2021) discusses vegetable contamination with both Ascaris spp. and Toxocara spp. eggs (Bowman, Reference Bowman2021), but given that Toxocara, unlike Ascaris, cannot be transmitted by humans and has the potential to contaminate meat as well as vegetable produce, the epidemiology of this parasite warrants individual attention.
To establish the public health risks posed by Toxocara in food, a new research focus is now required. This review highlights the key knowledge gaps in our understanding of food-borne toxocariasis, assessing the evidence for this route of transmission by means of published human cases, serological studies and recovery of Toxocara spp. eggs and larvae from foods. The ability of current food safety measures to prevent toxocariasis is addressed, alongside discussion into future research avenues to determine appropriate prevention strategies for this overlooked, but potentially significant issue.
Evidence of food-borne toxocariasis
Human case studies
The reported cases of human toxocariasis attributed to food consumption identified in the literature are shown in Table 1. In all cases, the food product was reported to have been consumed raw or only partially cooked. Some had missing information about the patients’ age or the species of animal or type of tissue consumed. In all studies listed, the diagnosis of human toxocariasis was made by patient serology.
Table 1. Reported food-borne human toxocariasis cases in the literature
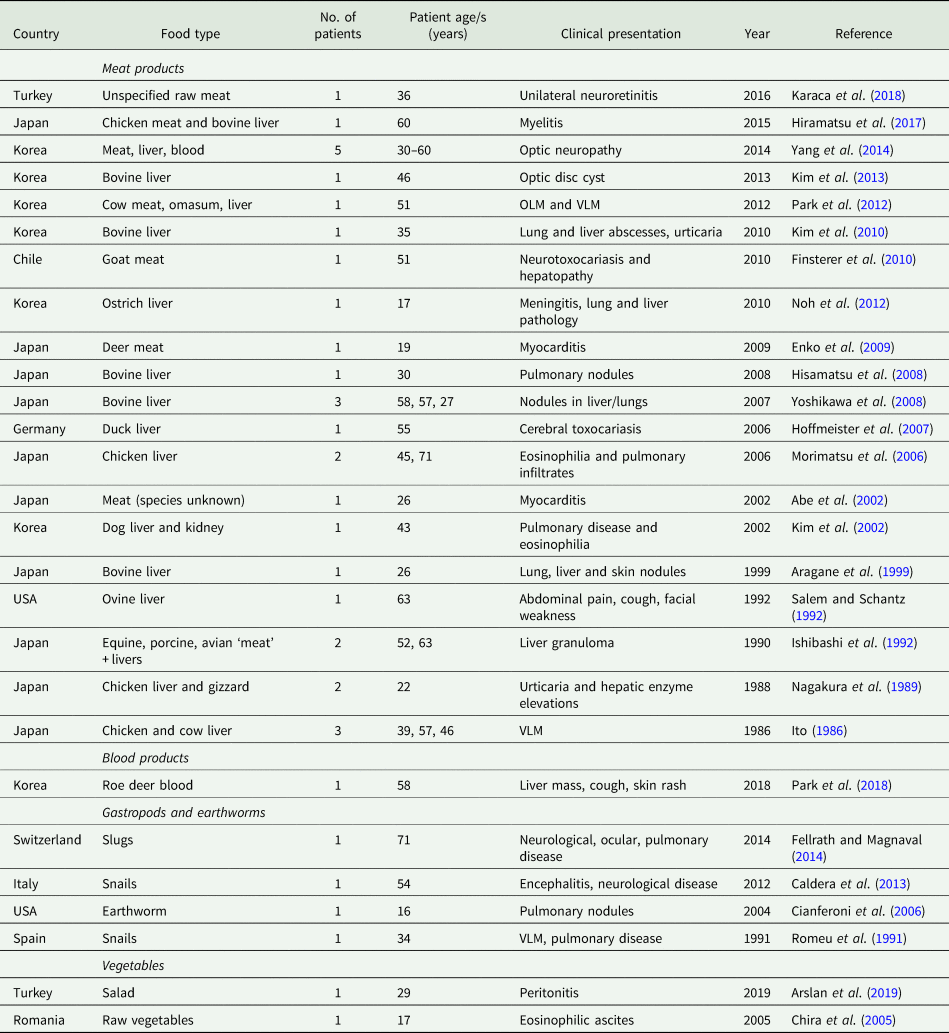
Abbreviations: OLM = Ocular larva migrans, VLM = Visceral larva migrans.
When reviewing published toxocariasis case reports, one must remain open to the possibility that we are only seeing the tip of the iceberg. Toxocariasis can have non-specific symptoms, which may be attributed to other aetiologies and not investigated, leading to under diagnosis (Magnaval et al., Reference Magnaval, Glickman, Dorchies and Morassin2001; Carlin and Tyungu, Reference Carlin and Tyungu2020). In many of the published case reports, details of the patients’ history were lacking: either no exposure history was taken by the clinician, the patient was not questioned on their dietary habits, or enquiries were only made regarding contact with animals. Cases were more commonly reported in countries where consumption of raw meat products is more widespread, an association noted elsewhere in the literature (Morimatsu et al., Reference Morimatsu, Akao, Akiyoshi, Kawazu, Okabe and Aizawa2006).
Larval distribution and persistence in animal tissues
Previous experimental studies have investigated the larval migration patterns for Toxocara spp. in various paratenic host species. In mice and rats, both T. canis and T. cati are initially detected in the liver and lungs during the so-called ‘hepato-pulmonary’ phase and are later found to undergo a ‘myotropic-neurotropic phase’, migrating to the skeletal tissues and brain (Abo-Shehada et al., Reference Abo-Shehada, Al-Zubaidy and Herbert1984). In mice, Toxocara cati is reported to be less likely than T. canis to infect brain tissue, and more likely to be found in the musculature (Havasiová-Reiterová et al., Reference Havasiová-Reiterová, Tomašovicová and Dubinský1995). Once present in these tissues, larvae have been found to persist for up to one year in mice (Bardón et al., Reference Bardón, Cuéllar and Guillén1994).
In rabbits experimentally infected with T. canis and T. cati, larvae could be recovered from the liver, lungs, kidneys and brain, with those in the liver being detected alive over 7 months post-infection; the musculature was not examined in this study (Pankavich, Reference Pankavich1966). In piglets fed embryonated T. canis eggs, larvae were recoverable from the lungs and the brain, as well as in tissues more commonly consumed by humans including the kidneys, liver and musculature. Larvae collected from the livers 30 days post-infection and subsequently fed to recipient mice were able to induce infection, confirming larval viability (Sasmal et al., Reference Sasmal, Acharya and Laha2008). However, in a separate study, significant decreases in larval numbers were observed up to 3 weeks post-infection, suggesting that larvae seem to be less able to persist for long periods in the tissues of pigs (Helwigh et al., Reference Helwigh, Lind and Nansen1999). In lambs fed infective T. canis eggs, larvae were detected in the liver and lungs but muscles were not examined (Aldawek et al., Reference Aldawek, Levkut, Revajová, Kolodzieyski, Ševěiková and Dubinský2002). An earlier study detected larvae in the muscles of lambs in addition to the pancreas, heart, kidney and brain, while larvae appeared to remain in the liver in year-old animals, not migrating to other tissues but remaining alive for the duration of the 12-week study (Schaeffler, Reference Schaeffler1960). Toxocara larvae within chicken tissues have been found to migrate to the lungs, liver, brain and muscles, with an ability to remain infective for prolonged periods of time. Toxocara canis larvae were found predominantly in chicken liver for up to 3.5 years (Tsvetaeva et al., Reference Tsvetaeva, Sosipatrova and Smirnov1979), whereas T. cati larvae appear to favour migration through the liver and lungs to the musculature, remaining infective to mice 176 days post-infection (Taira et al., Reference Taira, Saitoh and Kapel2011). Published studies in cattle are scarce, but a study reported the usual larval migration sites: liver, lungs, kidney and brain, in calves experimentally infected with T. canis. Muscle tissue was not examined for larvae in this study (Fitzgerald and Mansfield, Reference Fitzgerald and Mansfield1970).
Given that larval migration to the liver is a common finding in the published literature, it is easy to see why so many of the human cases reported have been attributed to the consumption of this particular tissue. Moreover, experimental studies provide evidence of larval migration to other tissues consumed by humans, including the musculature. Thus, the consumption of raw or undercooked meat products, in particular liver tissue, is best avoided to reduce the risk of developing toxocariasis.
Natural infections in food-producing animals
In addition to experimental studies, there are a limited number of reports where larvae have been detected in naturally infected animals. For example, T. cati has been detected in pig tissues intended for human consumption (Davidson et al., Reference Davidson, Mermer and Øines2012) and both T. canis and T. cati have been isolated from naturally infected chickens (Zibaei et al., Reference Zibaei, Sadjjadi and Maraghi2017; Okada et al., Reference Okada, Ooi and Taira2021). Indirect evidence of Toxocara infection in the form of serological data has also been published, with anti-Toxocara antibodies detected in several food-producing animals on farm and at slaughter. Lloyd (Reference Lloyd2006) found that up to 47% of sheep in a Welsh study had anti-Toxocara antibodies present in their blood, with levels directly proportional to animals’ age. This finding was supported by a 2011 Brazilian study, with 52.9% of female sheep between 11 and 15 months reported to have anti-Toxocara antibodies, compared with 5% sero-prevalence in lambs aged 0–6 months (Santarém et al., Reference Santarém, Chesine, Lamers, Rubinsky-Elefant and Giuffrida2011). Serological investigations have also been undertaken for chickens, with 58.5% (Campos-da-Silva et al., Reference Campos-da-Silva, da Paz, Fortunato, Beltrame, Valli and Pereira2015) and 67.7% (Oliveira et al., Reference Oliveira, Rubinsky-Elefant, Merigueti, Batista and Santarém2018) of birds in Brazil confirmed to have anti-Toxocara antibodies. Whilst circulating antibodies do not prove the presence of infective larvae in the tissues, they confirm exposure of animals to Toxocara spp. and indicate the presence of this parasite in farm environments and common exposure of food-producing animals, presumably by ingestion of infective eggs.
Contamination of Toxocara eggs on farms
Several published studies have investigated the contamination of agricultural environments with Toxocara eggs. In Poland, 34.6% of soil samples from conventional farms and 21.3% from organic farms were positive for Toxocara spp. eggs (Klapec and Borecka, Reference Klapec and Borecka2012). In the Philippines, the overall prevalence of Toxocara in soil samples was much lower at 4%, with no statistically significant difference reported between conventional and organic farms (Paller and Babia-Abion, Reference Paller and Babia-Abion2019). Eggs may be transferred in contaminated soil to vegetable crops destined for human consumption. In their study, Klapec and Borecka (Reference Klapec and Borecka2012) analysed various vegetable produce harvested with the surrounding soil, detecting eggs on 10.8% of the sampled produce from organic farms, and 19.2% from conventional farms (Klapec and Borecka, Reference Klapec and Borecka2012). An Iranian study found that overall, 3.97% of sampled vegetables were contaminated with Toxocara eggs (Fallah et al., Reference Fallah, Makhtumi and Pirali-Kheirabadi2016), slightly higher than the 1.68% prevalence reported in another study from the same country (Rostami et al., Reference Rostami, Ebrahimi, Mehravar, Fallah Omrani, Fallahi and Behniafar2016) and the 1.5% prevalence reported in Turkey (Kozan et al., Reference Kozan, Gonenc, Sarimehmetoglu and Aycicek2005). It is difficult to compare the results of these studies due to variations in sampling strategies and laboratory techniques, but these studies confirm the link between Toxocara eggs in the soil and contamination of vegetable produce.
Toxocara eggs can also reach people from soil or feces via invertebrates. There are reported human cases arising from gastropod ingestion, and one case from earthworm ingestion (Table 1) (Romeu et al., Reference Romeu, Roig, Bada, Riera and Muñoz1991; Cianferoni et al., Reference Cianferoni, Schneider, Schantz, Brown and Fox2006; Fellrath and Magnaval, Reference Fellrath and Magnaval2014). Whilst this situation is probably extremely rare, one must bear in mind that contamination of vegetables with common invertebrates such as these does occur and could pose a risk to consumers if produce is consumed unwashed. Cases of zoonotic disease from accidental ingestion of molluscs infected with nematodes such as Angiostrongylus cantonensis (Slom et al., Reference Slom, Cortese, Gerber, Jones, Holtz, Lopez, Zambrano, Sufit, Sakolvaree, Chaicumpa, Herwaldt and Johnson2002) attest to this possibility, while the possible growth of insects as human food in future (Babarinde et al., Reference Babarinde, Mvumi, Babarinde, Manditsera, Akande and Adepoju2020; Hawkey et al., Reference Hawkey, Lopez-Viso, Brameld, Parr and Salter2021) demands consideration of their potential as sources of zoonoses, including Toxocara. Insects are known to transfer taeniid cestode eggs from feces to food (Benelli et al., Reference Benelli, Wassermann and Brattig2021), although this route has not yet been demonstrated for Toxocara spp.
Sampling techniques and diagnostics for Toxocara spp.
Toxocara eggs in soil and vegetable produce
Most of the published methods for the recovery of Toxocara sp. eggs from soil and vegetables are based on conventional techniques, involving steps such as washing, sieving, sedimentation, filtration and flotation prior to microscopic examination. In some cases, egg viability determination is also undertaken, usually by means of dye uptake differentiation (Dabrowska et al., Reference Dabrowska, Zdybel, Karamon, Kochanowski, Stojecki, Cencek and Klapec2014). Sample sizes vary widely between studies. In the case of soil, from 3 g up to 200 g of dried sample has been used, which is typically extracted up to 5 cm from the soil surface (Paller and Babia-Abion, Reference Paller and Babia-Abion2019; Tyungu et al., Reference Tyungu, McCormick, Lau, Chang, Murphy, Hotez, Mejia and Pollack2020). For vegetable testing, preferred weights of samples usually range from 100 to 250 g, with the processing of sedimented washing solution and subsequent residue concentration commonly undertaken prior to parasitological analysis via microscopy (Abougrain et al., Reference Abougrain, Nahaisi, Madi, Saied and Ghenghesh2010; Fallah et al., Reference Fallah, Makhtumi and Pirali-Kheirabadi2016; Hajipour et al., Reference Hajipour, Soltani, Ketzis and Hassanzadeh2021). Some studies have additionally utilized PCR-based molecular assays, to differentiate between T. canis and T. cati eggs in soil samples (Choobineh et al., Reference Choobineh, Mikaeili, Sadjjadi, Ebrahimi and Iranmanesh2019; Tyungu et al., Reference Tyungu, McCormick, Lau, Chang, Murphy, Hotez, Mejia and Pollack2020) and vegetable produce (Guggisberg et al., Reference Guggisberg, Alvarez Rojas, Kronenberg, Miranda and Deplazes2020).
Toxocara spp. larvae in meat tissues and animal serology
In order to recover Toxocara larvae from meat products, the tissue is usually subjected to chemical digestion treatment using an HCl-pepsin solution with simultaneous incubation and mechanical stirring, followed by sedimentation, filtration and identification of larvae by microscopy. Subsequent molecular analysis of the larvae obtained using PCR-based techniques is commonly undertaken following isolation (Zibaei et al., Reference Zibaei, Sadjjadi and Maraghi2017; Okada et al., Reference Okada, Ooi and Taira2021).
For the purpose of identifying animals with circulating anti-Toxocara antibodies in their bloodstream, studies have utilized enzyme-linked immunosorbent assay (ELISA) based detection using the excretory-secretory (TES) antigens of T. canis larvae. To reduce cross-reactivity with Ascaris spp. and improve test specificity, serum samples are typically pre-adsorbed with the extract of an adult Ascaris worm prior to ELISA testing (Santarém et al., Reference Santarém, Chesine, Lamers, Rubinsky-Elefant and Giuffrida2011; Rassier et al., Reference Rassier, Borsuk, Pappen, Scaini, Gallina, Villela, da Rosa Farias, Benavides and Berne2013; Campos-da-Silva et al., Reference Campos-da-Silva, da Paz, Fortunato, Beltrame, Valli and Pereira2015). No attempt is usually made to differentiate between T. canis and T. cati infections in these assays, and cross-reactivity between these species is likely (Santarém et al., Reference Santarém, Chesine, Lamers, Rubinsky-Elefant and Giuffrida2011).
Whilst the presence of circulating anti-Toxocara antibodies does not definitively diagnose an active Toxocara infection, it is suggestive of exposure to this parasite. In contrast, identification of Toxocara spp. eggs in the feces of infected cats, dogs and foxes is more suggestive of a patent infection, with adult worms residing within the intestine of these definitive hosts (Fan, Reference Fan2020). The risk of a false-positive diagnosis due to the ingestion of faeces containing Toxocara spp. eggs can be reduced with the addition of ELISA-based coproantigen detection (Elsemore, Reference Elsemore2020), or repeat sampling following the gut transit time.
Diagnosing human toxocariasis
In the case of human Toxocara infections, diagnosis is most commonly based on the results of clinical and serological findings. As is the case for animal serological testing, ELISA-based tests are utilized to detect TES antibodies in the blood of human patients. As a confirmatory measure, following a positive ELISA result, Western blotting techniques are recommended which improve the sensitivity and specificity of diagnosis (Mazur-Melewska et al., Reference Mazur-Melewska, Mania, Sluzewski and Figlerowicz2020). Research to develop improved serodiagnostic assays for Toxocara sp. is ongoing. The use of recombinant antigens and the detection of antibody subclasses are approaches currently being explored for their potential to improve the reliability of testing for toxocariasis in humans in the future (Nicoletti, Reference Nicoletti2020). Improvements in the diagnosis of Toxocara spp. infections in humans would help to elucidate dietary risk factors by enabling larger and more precise epidemiological studies.
Control measures to minimize transmission risk
The potential flow of Toxocara spp. from the farm to the consumer, and a summary of the possible control measures at each step of the production chain are summarized in Fig. 1. While many food systems have steps in place to protect consumers from other pathogens, their effectiveness against Toxocara spp. has not been systematically tested.

Fig. 1. The potential flow of Toxocara spp. from the farm to the consumer, and a summary of the possible control measures at each step of the production chain.
Farm biosecurity
Toxocara spp. eggs are deposited in the soil via the feces of infected dogs, cats and foxes, and once present in the soil can remain infective for years due to their resistance to environmental conditions (Parsons, Reference Parsons1987). Because organic fertilizer does not typically incorporate the feces of these definitive hosts, its agricultural use should not usually pose a risk of contaminating the environment with the eggs of this parasite. No practical methods exist to remove Toxocara eggs from the environment (Overgaauw and van Knapen, Reference Overgaauw and van Knapen2013); therefore, controlling access of these definitive hosts to agricultural land is a logical first intervention point to explore for both meat and vegetable production processes.
An Italian study which assessed the level of Toxocara eggs in the soil of farms in the Marche region found that out of 60 farms sampled, around 50% had soil that tested positive for Toxocara. In addition, the proportion of positive farms was almost twice as high in the group which had more than three dogs living on-site, compared to farms that had three dogs or fewer (Habluetzel et al., Reference Habluetzel, Traldi, Ruggieri, Attili, Scuppa, Marchetti, Menghini and Esposito2003). Although scarce, other studies have also found Toxocara spp. on livestock farms. These include a 2002 study, which assessed a large-scale poultry unit and its surrounding environment in Poland, detecting Toxocara spp. eggs in soil within close proximity to the farm (Trawinska et al., Reference Trawinska, Tymczyna, Polonis, Pijarska and Saba2002), and a study in 2003 which assessed the environmental microbial composition of two pig farms in Poland, detecting Toxocara spp. eggs in the soil of both units sampled (Szostak and Bekier-Jaworska, Reference Szostak and Bekier-Jaworska2003).
There are other studies in the literature which have focused on determining the prevalence of Toxocara spp. in definitive hosts that could access agricultural land. For example, some authors have investigated Toxocara prevalence in free-roaming farm cats by faecal analysis: T. cati was detected in 91% of sampled cats in the UK (Yamaguchi et al., Reference Yamaguchi, Macdonald, Passanisi, Harbour and Hopper1996), whereas an earlier study obtained positive results in 63% of cats (Gethings et al., Reference Gethings, Stephens, Wills, Howard, Balfour, Wright and Morgan1987). In farm dogs, a Portuguese study sampling canine feces on 165 small-ruminant farms detected a Toxocara prevalence of 8% using microscopic evaluation (Cardoso et al., Reference Cardoso, Costa, Figueiredo, Castro and Conceição2014), and studies assessing T. canis infection in foxes found prevalence levels of 47.4% in Zurich, Switzerland (Hofer et al., Reference Hofer, Gloor, Muller, Mathis, Hegglin and Deplazes2000); and 55.9% in the UK (Richards et al., Reference Richards, Harris and Lewis1995). It is always difficult to compare the results of studies such as this directly, because of variations in sampling techniques and laboratory methods. In these studies, the soil was not analysed, but infected animals potentially had access to farmland, in particular land utilized for arable production or livestock grazing. In the previously referenced study reporting a 47% sero-prevalence of T. canis in Welsh sheep, all participating farms had dogs resident on-site, and foxes were also present (Lloyd, Reference Lloyd2006). In addition, stray cats of unknown health status were present on farms from which T. cati larvae were isolated from pig meat destined for human consumption in Norway (Davidson et al., Reference Davidson, Mermer and Øines2012), and chicken meat from a commercial unit in Japan (Okada et al., Reference Okada, Ooi and Taira2021). As well as defecating onto agricultural land, there is the potential for definitive hosts, mainly cats, to contaminate animal feed stores. This route of infection has been seen in cases of toxoplasmosis in pigs, a parasitic infection which shares several features with toxocariasis including a common definitive host (Li et al., Reference Li, Wang, Yu, Li and Zhang2010).
Regular treatment of farm cats and dogs with appropriate anthelmintic drugs in accordance with guidelines such as those of the European Counsel for Companion Animal Parasites (ESCCAP) is one approach to reducing the contamination of the agricultural environment with Toxocara eggs, particularly for kittens, pups and nursing queens and bitches (Overgaauw and van Knapen, Reference Overgaauw and van Knapen2013). A 2016 study by Nijsse reported that fewer than a quarter of cat owners questioned were treating animals with an anthelmintic at the recommended frequency, with a higher risk of Toxocara shedding in free roaming cats (Nijsse et al., Reference Nijsse, Ploeger, Wagenaar and Mughini-Gras2016). Treating owned animals is just one part of the picture. A meta-analysis showed the prevalence of Toxocara in stray cats, working and rural dogs to be significantly higher than that of pet cats and dogs (Rostami et al., Reference Rostami, Riahi, Hofmann, Ma, Wang, Behniafar, Taghipour, Fakhri, Spotin, Chang, Macpherson, Hotez and Gasser2020), and parasites in foxes should also be considered (Deplazes et al., Reference Deplazes, Hegglin, Gloor and Romig2004).
In a recent study, Hajipour et al. (Reference Hajipour, Soltani, Ketzis and Hassanzadeh2021) assessed the impact of fencing-off farmland on the contamination of vegetables with T. canis and T. cati in Iran and found unfenced cropping areas had a vegetable contamination rate of 55.3% compared to 9.2% in fenced areas (Hajipour et al., Reference Hajipour, Soltani, Ketzis and Hassanzadeh2021). Whilst this control step seems logical, the size of the farms is important to consider: in this study, the largest site was 20 hectares, considerably smaller than the average farm size of 87 hectares in England, UK (Defra, 2021). Farm size could impact the feasibility and economic viability of biosecurity measures such as fencing.
Meat inspection at slaughter
In terms of reducing the risk of parasitic transmission to humans, meat inspection is currently the principal means of controlling Trichinella spp. and Taenia spp. infections in the food chain (Dorny et al., Reference Dorny, Praet, Deckers and Gabriel2009). At this time, the presence of Toxocara spp. larvae in the tissues of food animals is not assessed at slaughter in any jurisdiction. Indeed, Toxocara larvae are frequently seen as a ‘contaminant’ when isolated during routine porcine Trichinella testing by artificial tissue digestion (Marucci et al., Reference Marucci, Interisano, La Rosa and Pozio2013), and their presence is often not reported. Some recent studies have advocated the use of molecular methods to improve the detection of helminth contamination of meat and offal (Nguyen et al., Reference Nguyen, Wang, Maruyama, Horii, Nonaka and Yoshida2017; Wang et al., Reference Wang, Shibata, Nguyen, Hayata, Nonaka, Maruyama and Yoshida2018; Karadjian et al., Reference Karadjian, Kaestner, Laboutière, Adicéam, Wagner, Johne, Thomas, Polack, Mayer-Scholl and Vallée2020). This is an area which has the potential to greatly improve the detection of Toxocara during the meat inspection process and warrants further investigation.
Washing vegetable produce
The contamination of vegetable produce at source and human consumption without washing is a potential transmission route for several parasites, including Toxocara spp. (Slifko et al., Reference Slifko, Smith and Rose2000; Mosayebi et al., Reference Mosayebi, Hajihossein, Didehdar, Eslamirad, Ejtehadifar and Hamzelo2014). Several published studies have assessed the degree of contamination of vegetable produce with the eggs of Toxocara spp. worldwide, with leafy vegetables such as salad leaves seemingly more susceptible compared to other vegetable types. For example, in Iran, Hajipour et al. (Reference Hajipour, Soltani, Ketzis and Hassanzadeh2021) reported 40.2 and 33.0% of lettuce samples tested to contain the eggs of T. cati and T. canis respectively, compared with radishes from which only T. cati was isolated in 6.1% of samples (Hajipour et al., Reference Hajipour, Soltani, Ketzis and Hassanzadeh2021). In Libya, T. canis and T. cati eggs were detected in 37 and 48% of lettuces compared to 14 and 8% of cucumbers, respectively (Abougrain et al., Reference Abougrain, Nahaisi, Madi, Saied and Ghenghesh2010). The higher levels of contamination reported on leafy vegetables could be due to an increased surface area in contact with the contaminated soil surface (Gupta et al., Reference Gupta, Khan and Santra2009; Maikai et al., Reference Maikai, Elisha and Baba-Onoja2012). Studies have assessed the effectiveness of washing different vegetables in reducing parasitic contamination. Avcioglu et al. (Reference Avcioglu, Soykan and Tarakci2011) did not detect any helminth eggs in vegetable samples following a washing step with clean water alone (Avcioglu et al., Reference Avcioglu, Soykan and Tarakci2011), a finding supported by other studies in the literature (Fallah et al., Reference Fallah, Pirali-Kheirabadi, Shirvani and Saei-Dehkordi2012; Rostami et al., Reference Rostami, Ebrahimi, Mehravar, Fallah Omrani, Fallahi and Behniafar2016). Alternative washing methods for the removal of parasitic species from vegetables have been investigated, with 0.95% calcium hypochlorite solution found to be more effective at reducing parasitic contamination than 1% lemon juice, 1% vinegar or a diluted dishwashing detergent (Hajipour et al., Reference Hajipour, Soltani, Ketzis and Hassanzadeh2021). However, consumer safety and public acceptance of chemical food treatments need to be considered. In some countries, for example, the use of chlorine-based disinfectants for food produce is highly restricted or not authorized (De Corato, Reference De Corato2020).
Chilling and freezing of foods
Refrigeration is used throughout the food chain, from the production stage through to the consumer, to maximize food quality and safety (Tassou et al., Reference Tassou, Lewis, Ge, Hadawey and Chaer2010). However, there are very few published studies assessing the effect of chilling and freezing on the viability of Toxocara spp. eggs and larvae, particularly in food products. A study by Taira et al. (Reference Taira, Saitoh and Kapel2011) demonstrated that the infectivity of T. cati larvae within chicken muscle tissue reduced substantially following chilling at 4°C for 14 and 28 days, but larvae were still viable and induced infections in recipient mice (Taira et al., Reference Taira, Saitoh and Kapel2011). In a similar study, chilling pig and poultry tissues at 4°C for 7 days had a significant effect on larval infectivity, but again infections could still be established in recipient pigs (Taira et al., Reference Taira, Saeed, Permin and Kapel2004). Chilling infected mouse liver samples to between 0 and 4°C for 10 days was found to significantly reduce the intensity of infection in recipient mice (Dutra et al., Reference Dutra, Pinto, da Costa de Avila, Telmo, Hora, Martins, Aires Berne and Scaini2013). These studies suggest the potential risk of Toxocara transmission by consumption of meat products is decreased by refrigeration, but the risk of human infection remains.
Freezing of meat tissues appears to have a more significant effect on the viability of Toxocara larvae compared to refrigeration. Storing infected muscle tissue at −21°C for 12–48 h was found to have a dramatic impact on larval viability, with no subsequent infections detected in recipient mice. Larval motility was reported as absent after 24 h freezing at −25°C, suggesting that the thermal death point for the larvae had been reached (Taira et al., Reference Taira, Saitoh and Kapel2011). This finding was supported by Dutra et al. (Reference Dutra, Pinto, da Costa de Avila, Telmo, Hora, Martins, Aires Berne and Scaini2013), with a 100% reduction in larval viability following freezing of mouse liver tissue at −20°C, with larvae showing detrimental morphological changes including ruptured cuticles and internal organ degeneration (Dutra et al., Reference Dutra, Pinto, da Costa de Avila, Telmo, Hora, Martins, Aires Berne and Scaini2013). In contrast, Sprent (Reference Sprent1953) reported finding motile larvae in mice carcasses subjected to −20°C for 4 weeks, although the viability of these larvae was not assessed (Sprent, Reference Sprent1953). Freezing meat products is a promising potential control point to investigate, and is utilized for some other food-borne parasites such as Trichinella spiralis (Noeckler et al., Reference Noeckler, Pozio, van der Giessen, Hill and Gamble2019). This would be especially important for meat intended to be served in raw dishes, such as steak tartare. However, effects on food quality and palatability brought about by the freezing process need to be considered if this control measure is implemented during processing (Zhang et al., Reference Zhang, Mao, Li, Luo and Hopkins2019).
The environmental resistance of Toxocara spp. eggs is widely reported (Mizgajska, Reference Mizgajska2001; Despommier, Reference Despommier2003), with studies demonstrating the tolerance and development of eggs at a range of ambient temperatures (Azam et al., Reference Azam, Ukpai, Said, Abd-Allah and Morgan2012). A study by Azam et al. (Reference Azam, Ukpai, Said, Abd-Allah and Morgan2012) assessed the impact of low temperatures on Toxocara eggs. Toxocara canis eggs were stored at +1 and −2°C for a 6-week period, during which time they did not embryonate to become infective, and their subsequent development was delayed once returned to higher temperatures (Azam et al., Reference Azam, Ukpai, Said, Abd-Allah and Morgan2012). Whilst consumers are unlikely to routinely store vegetables for this length of time in domestic refrigerators, storing vegetable produce at low temperatures could postpone the development of eggs such that any consumed are less likely to be fully larvated and thus infective. Freezing eggs was found to reduce the viability of T. canis and T. cati, with longer treatment times correlating with reduced viability (O'Lorcain, Reference O'Lorcain1995). As is the case for meat products, the process of freezing vegetable produce can lead to structural changes and a reduction in product quality, with some types of vegetable more negatively impacted by freezing than others (Jeremiah, Reference Jeremiah2019).
Cooking foods
Heat treatment remains one of the most reliable methods to inactivate food contaminated with parasites, such as Taenia spp., Toxoplasma gondii and some Trichinella spp., provided the internal temperature is high enough (Franssen et al., Reference Franssen, Gerard, Cozma-Petruţ, Vieira-Pinto, Jambrak, Rowan, Paulseng, Rozyckih, Tysnesi, Rodriguez-Lazaroj and Robertson2019). For example, in the case of Taenia solium infected pork, cooking for 10 min at a core temperature of 80°C, 20 min at 70°C or 30 min at 60°C has been found to kill cysticerci, but at core temperatures of 40°C, metacestodes were still viable after up to 1 h of cooking. This raises some concerns for faster cooking methods, such as deep frying (Møller et al., Reference Møller, Ngowi, Magnussen, Magne, Kabululu and Johansen2020). In comparison, T. gondii and T. spiralis appear to be more sensitive to heat treatment, with T. gondii tissue cysts killed following exposure to temperatures above 56°C for 10 min (Dubey et al., Reference Dubey, Kotula, Sharar, Andrews and Lindsay1990), and a 15 min cooking time at 55.6°C sufficient to inactivate T. spiralis larvae in pork (Noeckler et al., Reference Noeckler, Pozio, van der Giessen, Hill and Gamble2019).
Publications outlining the effect of the cooking process on Toxocara spp. larvae in animal tissues and eggs on vegetable produce are currently lacking. A 2007 study assessed the infectivity of T. canis larvae present in mouse liver following cooking for 5 min in a household microwave, with the internal temperature of the tissue reaching >70°C. Transmission of Toxocara to recipient mice only occurred if infected liver was consumed raw (Cetinkaya et al., Reference Cetinkaya, Gargili and Altaş2007). To assess this control step further, it would be necessary to determine precise thermal death curves (time vs temperature) for Toxocara spp. tissue larvae. This would be especially useful for meat more commonly served ‘rare’, such as beef, and to assess the risk of cooking methods more commonly associated with under-cooking, such as barbequing.
Conclusions and future directions
Human toxocariasis is a neglected disease which has a significant negative impact on global public health. It has been known for some time that people can acquire Toxocara by ingesting larvated eggs from the environment, but there is also mounting evidence for food-borne transmission of this parasite. Seropositivity and viable larvae in food animals and Toxocara eggs on vegetable produce have been demonstrated and are of great concern, especially as current food safety measures do not specifically take this parasite into consideration. However, current knowledge of the true importance of food consumption in the epidemiology of toxocariasis is lacking. Further research is now required to quantify the flow of Toxocara from the environment to the final food product and evaluate the potential impact of any control interventions. A multifaceted approach will be required to address the different steps in the food production pathway, with molecular techniques opening the doors to more sensitive detection methods for Toxocara species to support both research and food safety. A renewed research focus is urgently required to fill in the key gaps, inform food safety policy and, ultimately, protect consumer health.
Financial support
Sara Healy is funded by UK Research and Innovation, Biotechnology and Biological Sciences Research Council, through the FoodBioSystems Doctoral Training Programme.
Conflict of interest
None.
Ethical standards
Not applicable.