Introduction
Natural honey has been recognised for its medicinal and nutritional properties for more than 2000 years. Based on botanical sources, honey may be classified as floral (from nectar of flowers), non-floral/honeydew (from deposits secreted by the living parts of plants or excreted onto them by sap-sucking insects) and mixed (nectar and honeydew)(Reference Castro-Vázquez, Díaz-Maroto and Pérez-Coello1 , Reference Manyi-Loh, Ndip and Clarke2). Depending on the source, the chemical composition varies with different types of honey. Honey is composed mainly of sugars (about 76 %), with fructose being the major monosaccharide, and water (less than 20 %)(Reference Martinotti and Ranzato3). Honey has been reported to exhibit a broad range of biological properties including anti-bacterial(Reference Alvarez-Suarez, Tulipani and Díaz4), anti-inflammatory(Reference Afrin, Gasparrini and Forbes-Hernández5 , Reference Gasparrini, Afrin and Forbes-Hernández6), antioxidant(Reference Alvarez-Suarez, Giampieri and Battino7), anti-ulcer(Reference Almasaudi, El-Shitany and Abbas8) and anti-tumour(Reference Badolato, Carullo and Cione9) activities. Many studies have shown that the phenolic and flavonoid components of honey are mainly responsible for its biological activities. The phenolic compounds have also been proposed as biomarker compounds for identification of unifloral honeys(Reference Miguel, Antunes and Faleiro10).
There is an increasing trend in the usage of complementary medicines by cancer patients along with standard chemotherapeutic drugs, for reducing chemotherapy-associated side effects, enhancing anti-tumour immunity and improving cancer-related symptoms(Reference Byeongsang, Butow and Mullan11 , Reference Erejuwa, Sulaiman and Wahab12). Honey has been used as a part of complementary medicine to treat diverse diseases for many years. However, more recently, there has been an increased interest in the anti-cancer properties of various types of honey because of their different bioactive compounds. Several mechanisms have been reported to explain the anti-cancer or chemoprotective activities of honey, with studies ranging from cell culture to animal models and clinical trials. The present review focuses on the chemical composition and bioavailability of honey and the reported in vitro, preclinical and clinical studies with different types of honey in the context of cancer.
Bioactive profile of honey
The bioactive profile of honey is a complex one to describe since it is a combination of approximately 200 compounds, consisting of different types of sugars, proteins, free amino acids, organic acids, essential minerals, water, enzymes, vitamins, volatile compounds, pigments and a variety of phenolic compounds(Reference Alvarez-Suarez, Giampieri and Battino7 , Reference da Silva, Gauche and Gonzaga13).
Chemical composition of honey
The chemical composition of honey is variable, as already reported(Reference Battino, Forbes-Hernández and Gasparrini14). These diversities mainly depend on the floral source and geographical regions together with some external factors, such as seasonal and environmental factors, processing and storing conditions (Fig. 1)(15).
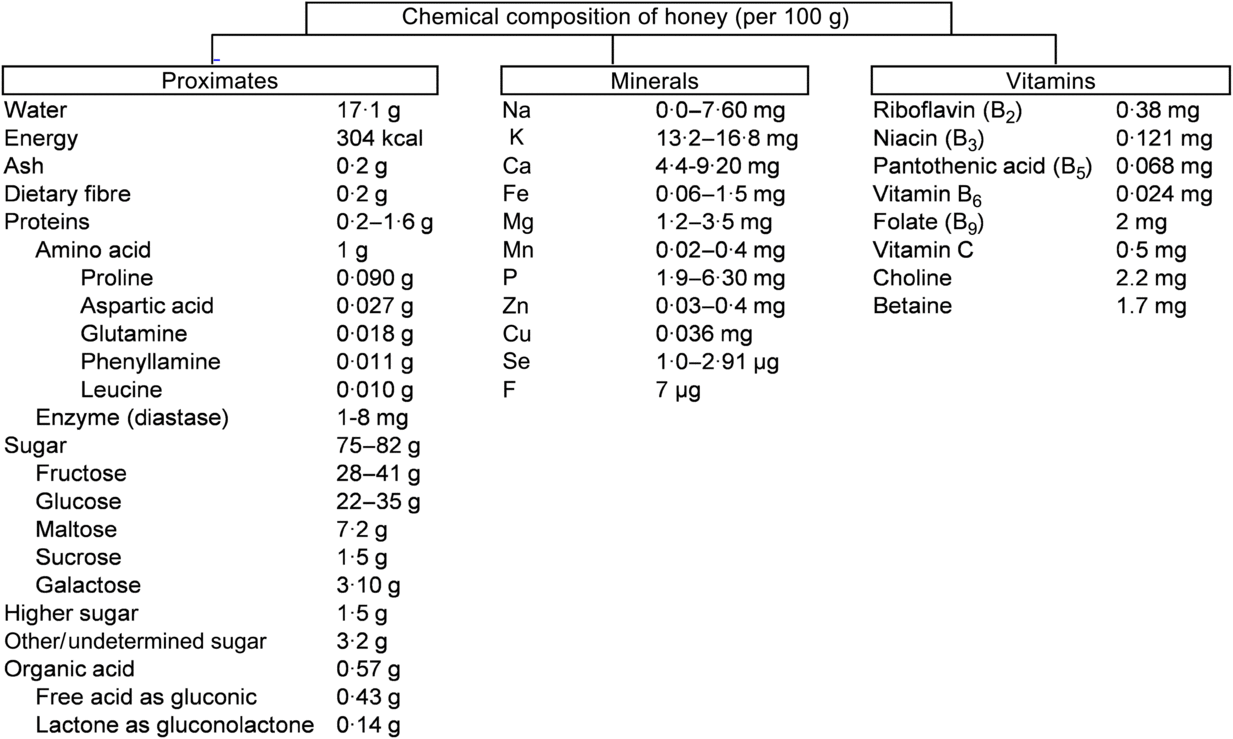
Fig. 1. Chemical composition of honey according to the United States Department of Agriculture(15). To convert kcal to kJ, multiply by 4·184.
Sugar in honey
About 75 % of the sugars present in honey are monosaccharides, fructose (about 40 %) and glucose (about 30 %) being the main components. In addition, 10–15 % are disaccharides, mainly maltose (about 7·20 %), sucrose (about 1·50 %) and small amounts of turanose, isomaltose, maltulose, trehalose, nigerose and kojibiose. The most abundant trisaccharides are maltotriose and melezitose(Reference Alvarez-Suarez, Giampieri and Battino7). Depending on the analytical technique used for the analysis of various types of honey, different types of disaccharides and trisaccharides have been identified in honey(Reference Alvarez-Suarez, Tulipani and Romandini16). Most of the disaccharides and trisaccharides (sucrose and maltotriose) are enzymically hydrolysed to monosaccharides. For example, sucrose contains one molecule of fructose linked to glucose by α-1,4 bonding. An equimolar mixture of hexoses is produced by hydrolysing with the enzyme invertase(Reference Kamal and Klein17). Similarly, maltotriose contains three molecules of glucose units which produce maltose by enzymic hydrolysis. Maltose again converts molecules of glucose by hydrolysing with the enzyme glucosidase(Reference Soldatkin, Peshkova and Saiapina18). The properties and the concentration of sugars in honey mainly depend on the botanical origin (types of flower used by honeybees), geographical origin (climate factors), and processing and storage conditions(Reference Escuredo, Dobre and Fernández-González19). The ratio between fructose and glucose is a useful marker of the categorisation of monofloral honey. Honey is an important source of energy for the human body as it is easy to digest and its main components (glucose and fructose) are quickly transported to the blood to provide the required energy. Interestingly, 100 g of honey provide 304 kcal (1272 kJ) which is equivalent to 64 kcal (268 kJ) per tablespoon (21 g)(15). A daily dose of 21 g represents 3 % of the daily recommended energy intake.
Proteins, amino acids and enzymes in honey
Depending on the species of honeybee, the content of proteins varies. For example, honey from Apiscerana contains 0·1–3·3 % of proteins, whereas honey from Apis mellifera contains 0·2–1·6 %(Reference Won, Lee and Ko20). Amino acids make up about 1 % (w/w) of honey. Proline is the major amino acid in honey, representing about 50–80 % of the total amino acid content. Usually, proline is created from the salivary discharge of honeybees (A. mellifera L.) during the conversion of nectar into honey(Reference Truzzi, Annibaldi and Illuminati21 , Reference Iglesias, De Lorenzo and Del Carmen Polo22). Proline content in honey has been used as an indicator of maturity of honey, and sometimes to check for adulteration with sugars. In pure honey, 180 mg of proline is the minimum accepted value per kg of honey(Reference Manzanares, García and Galdón23). Other amino acids identified in honey are glutamic acid, aspartic acid, glutamine, histidine, glycine, threonine, β-alanine, arginine, α-alanine, aminobutyric acid, proline, tyrosine, valine, methionine, cysteine, isoleucine, leucine, tryptophan, phenylalanine, ornithine, lysine, serine, asparagine and alanine(Reference Hermosín, Chicón and Cabezudo24). Honey also contains a small proportion of proteins in the form of enzymes. For example, invertase (sucrase, α- and β-glucosidases) hydrolyses sucrose into fructose. Invertase present in the honey sustains its activity when honey is ripened. The enzyme diastase (α- and β-amylases) hydrolyses starch chains into dextrin and maltose. This enzyme is used as an indicator of honey quality: high-quality honeys contain large amounts of diastase. Lastly, glucose oxidase converts glucose into δ-gluconolactone, producing gluconic and acid H2O2 (bactericidal properties)(Reference Bogdanov, Jurendic and Sieber25).
Organic acids in honey
All types of honey have minor acidity due to the presence of about 0·57 % of organic acids. These organic acids are produced by the honeybees during the conversion of nectar into honey(Reference da Silva, Gauche and Gonzaga13). Organic acids are used as a marker for differentiating the botanical or geographical origin of the honey and are related to the colour, flavour, acidity, pH and electrical conductivity. Moreover, the presence of these acids increases the stability of honey against micro-organisms and are partly associated with bactericidal properties(Reference Alvarez-Suarez, Giampieri and Battino7). While gluconic acid is the main component, other acids like aspartic acid, butyric acid, citric acid, acetic acid, formic acid, fumaric acid, galacturonic acid, glutamic acid, glutaric acid, glyoxylic acid, 2-hydroxybutyric acid, α-hydroxyglutaric acid, isocitric acid, α-ketoglutaric acid, lactic acid, malic acid, malonic acid, methylmalonic acid, 2-oxopentanoic acid, propionic acid, pyruvic acid, quinic acid, shikimic acid, succinic acid, tartaric acid and oxalic acid have also been reported(Reference da Silva, Gauche and Gonzaga13).
Vitamins and minerals in honey
Honey contains a very small amount of vitamins, most of them belonging to the vitamin B complex, including thiamine (B1), riboflavin (B2), nicotinic acid (B3), pantothenic acid (B5), pyridoxine (B6), biotin (B8 or H) and folic acid (B9). Vitamin C is also present in honey but its amount is difficult to determine given its instability due to its chemical and enzymic oxidation(Reference Bogdanov, Jurendic and Sieber25). The mineral content of honey varies from 0·04 to 0·2 % depending of the type of honey. Botanically, honeys can be classified according to their mineral content which depends on the geographical origin, and the type of soil in which the plant and nectar were found(Reference da Silva, Gauche and Gonzaga13). Honey contains several mineral elements of which K is the most abundant, representing one-third of the total minerals identified in honey. Other minerals in honey, present in small quantities, are Na, Fe, Cu, Si, Mn, Ca and Mg(Reference da Silva, Gauche and Gonzaga13).
Aroma and volatile compounds in honey
The aroma of honey is generated by the complex mixture of various volatile compounds, which may vary depending on the floral or botanical origin, processing and storage conditions. Unifloral honey has a typical aroma of plants because of the presence of specific volatile compounds from the nectars(Reference Castro-Vázquez, Díaz-Maroto and Pérez-Coello26). The flavour of honey is a vital quality for its use in the food industry as well as a selection criterion for consumer choice. The most common are cis-rose, trans-8-p-menthan-oxide-1,2-diol and 3,9-epoxy-1-p-mentadieno, which have been used as characteristic markers for lemon honey; sulfur compounds, diketones, and alkanes are used as markers for eucalyptus honey; heptanal and hexanal are used as markers for lavender honey; and methyl anthranilate, lilac aldehyde, hotrienol and 1-p-menthen-al are markers for citrus honey(Reference Alvarez-Suarez, Giampieri and Battino7 , Reference da Silva, Gauche and Gonzaga13).
Phenolic profile of honey
The phenolic components of honey are secondary metabolites of the plant, biosynthesised mostly for protection against oxidative damage and stress, and transmitted through the nectar to the honey. Two major families of phenolic compounds have been identified in honey: flavonoids and phenolic acids (Table 1)(Reference Moniruzzaman, Amrah Sulaiman and Gan27 – Reference Nweze, Okafor and Nweze47).
Table 1. Most common identified phenolic compounds and antioxidant capacity of studied honeys
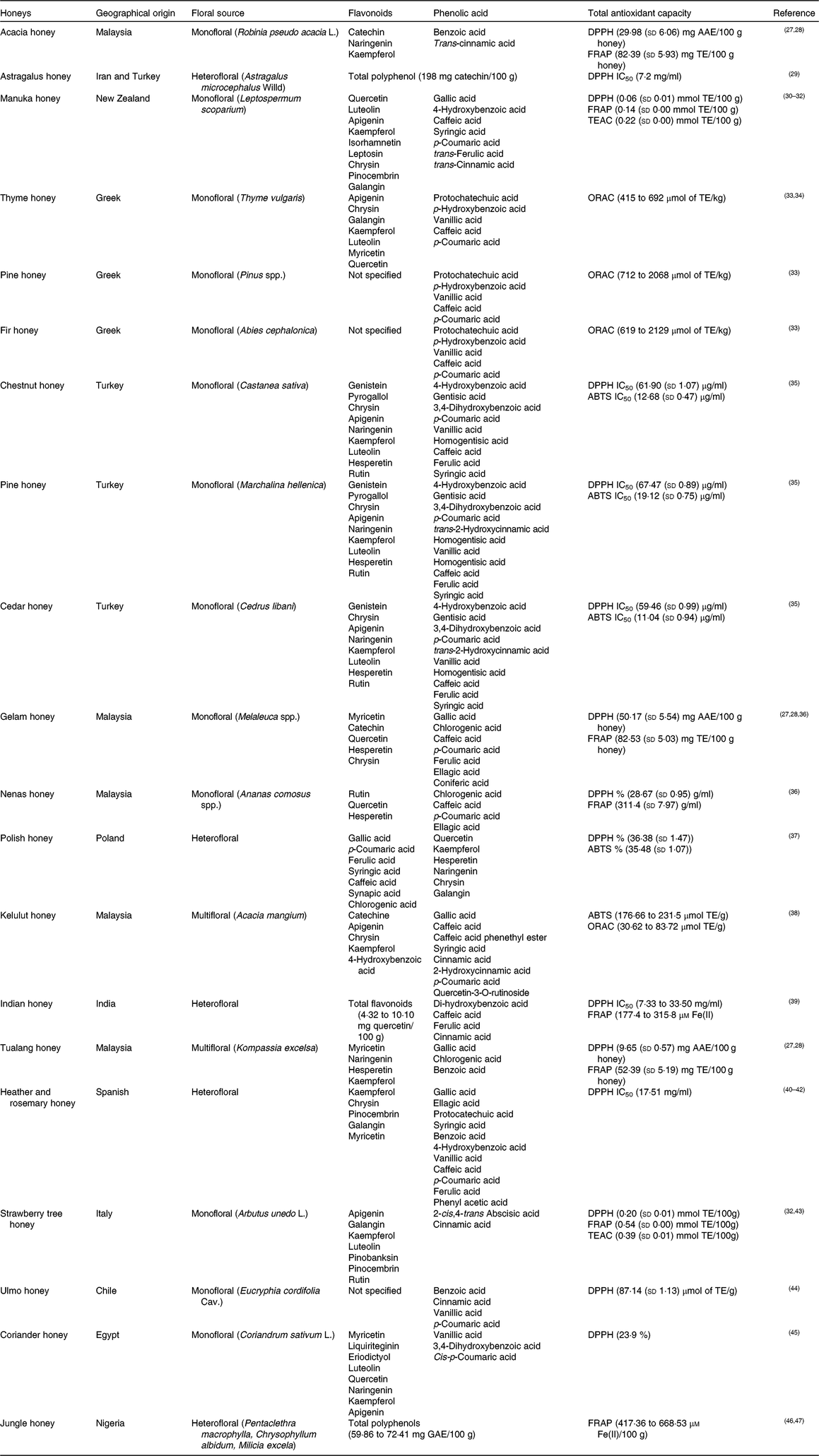
AAE, ascorbic acid equivalents; ABTS, 2,2'-azino-bis (3-ethylbenzothiazoline-6-sulphsulfonic acid; DPPH, 2,2-diphenyl-1-picrylhydrazyl radical; GAE, gallic acid equivalents; FRAP, ferric-reducing antioxidant power; IC50, half maximal inhibitory concentration (at the maximum concentration of honey in water, 45 g/l); ORAC, oxygen radical absorbance capacity; TE, Trolox equivalents; TEAC, Trolox equivalent antioxidant capacity.
This variability corresponds with the basis of the two major research themes belonging to the study of the phenolic fraction of honey: (i) the evaluation of the overall bioactive properties of honey from diverse geographical or botanical origins; and (ii) the geographical and/or floral origin of honey on the basis of the presence and abundance of one or more specific phenolic compounds, proposed as chemical marker(s) of origin(Reference Badolato, Carullo and Cione9).
Flavonoids in honey
Flavonoids are the main functional components of honey. They have a C6–C3–C6 nuclear structure, linking two benzene rings joined by a pyran ring. Replacement on the rings results in major classes of flavonoids: flavonols, flavones and flavanones. The concentration of flavonoids in honey is about 20 mg/kg and it differs depending on the botanical origin of the honey(Reference Alvarez-Suarez, Tulipani and Romandini16). According to different studies, the major flavonoid compounds identified in honey are: flavonols (quercetin, myricetin, kaempferol); flavones (chrysin, apigenin, luteolin, diosmetin); flavanones (hesperetin, pinocembrin, naringenin); and flavanols (catechin, epicatechin, epigallocatechin, epigallocatechin gallate) (Fig. 2). The highest content of flavonoids is found in manuka honey (a new Zealand monofloral honey), tualang honey (a multifloral honey originating from Malaysia) and buckwheat honey (a monofloral honey derived from various geographical origins), whereas the lowest content is observed in gelam honey and acacia honey(Reference Porcza, Simms and Chopra48). The variation usually depends not only on the floral, botanical and geographical origins, but also on the HPLC method used to determine these compounds(Reference Ciulu, Spano and Pilo49).
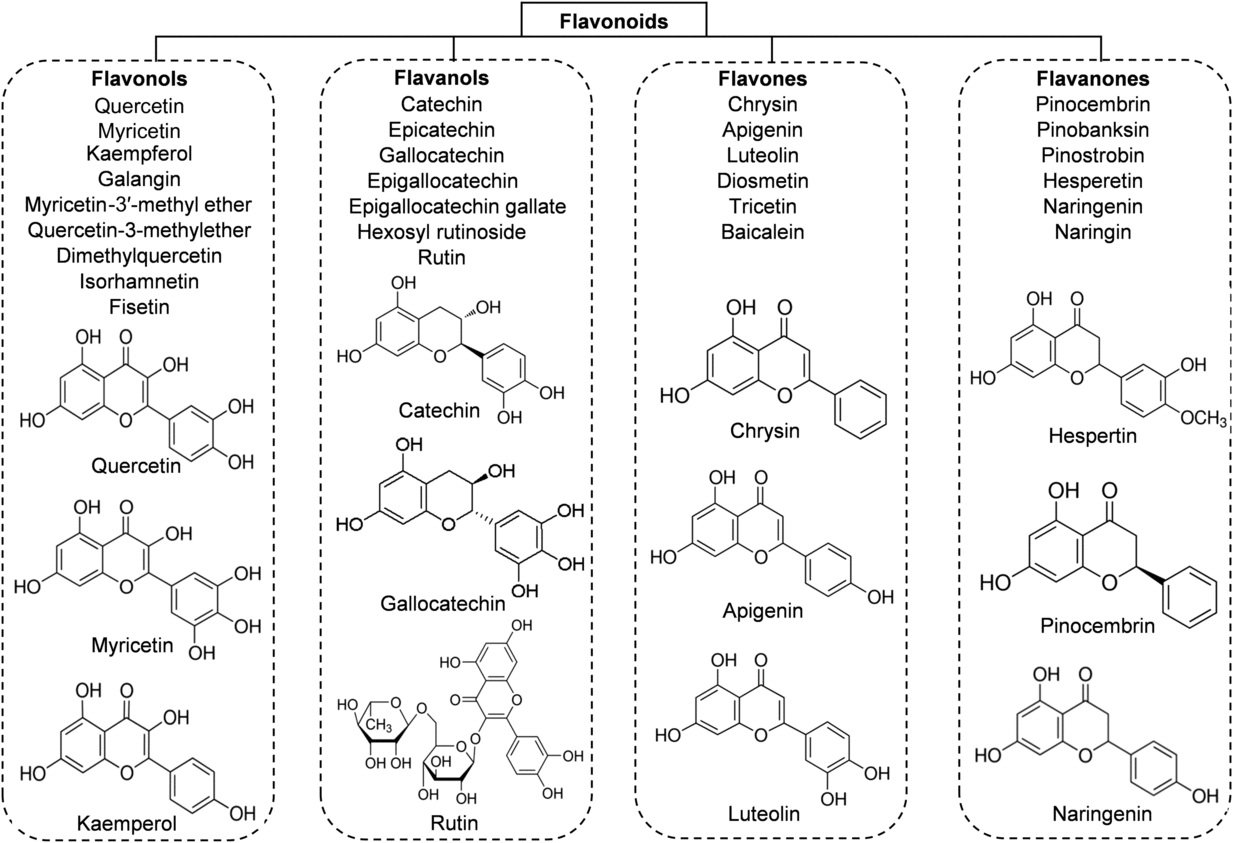
Fig. 2. Main classes of honey flavonoids with their chemical structures.
Phenolic acids in honey
The phenolic acids of honey can be divided, based on their chemical structure, into two subgroups: hydroxybenzoic acids and hydroxycinnamic acids. All hydroxybenzoic acids share a C1–C6 nuclear structure, derived from benzoic acid, but they differ in the hydroxylation and methylation of the aromatic ring(Reference da Silva, Gauche and Gonzaga13). The most common hydroxybenzoic acids found in honey are benzoic acid, vanillic acid, syringic acid, salicylic acid, gallic acid and ellagic acid (Fig. 3). Hydroxycinnamic acids usually share the nuclear structure C3–C6 and exhibit differences in the original rings (phenylacetic acids and acetophenones). The major identified hydroxycinnamic acids in honey are caffeic acid, p-coumaric acid, ferulic acid and sinapic acids (Fig. 3). Other phenolic acids such as p-hydroxycinnamic acids and chlorogenic acid could also be present in honey, depending on the botanical origins(Reference Alvarez-Suarez, Giampieri and Battino7 , Reference da Silva, Gauche and Gonzaga13).
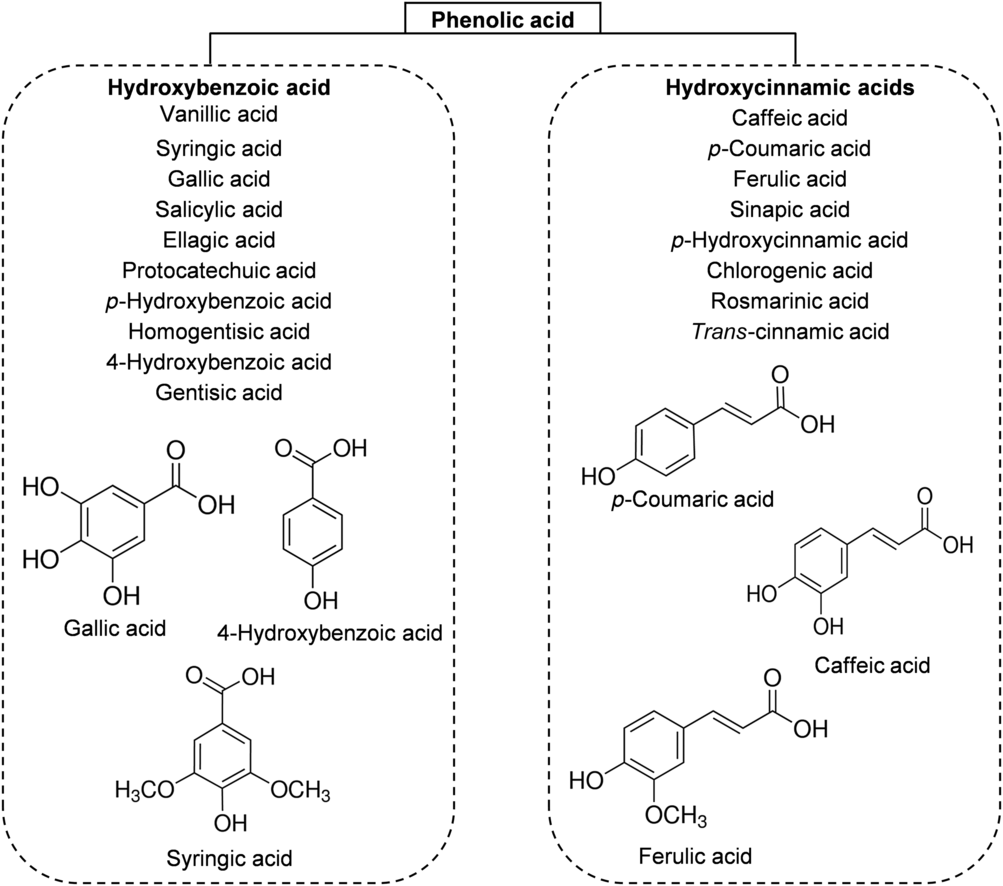
Fig. 3. Main classes of honey phenolic acids with their chemical structures.
Bioavailability and metabolites of honey
From a nutritional point of view, bioavailability is the fraction of a nutrient present in a food that is absorbed, retained and used for physiological functions through normal pathways. It is well established from animal and human studies that ingested phenolic compounds (from food sources) survive digestion in the upper digestive tract and reach different parts of the proximal and distal intestine in substantial doses. During the absorption process, phenolics are conjugated (usually methylated, sulfated and glucuronidated) in the small intestine and later in the liver, a metabolic detoxification process that facilitates biliary and urinary elimination. The colonic epithelium is in contact with both the parent and depredated phenolic compounds, which are widely metabolised to simpler phenolics by colonic microbiota and their metabolites can then be detected in urine, faeces, blood and tissue (Fig. 4)(Reference Manach, Williamson and Morand50).
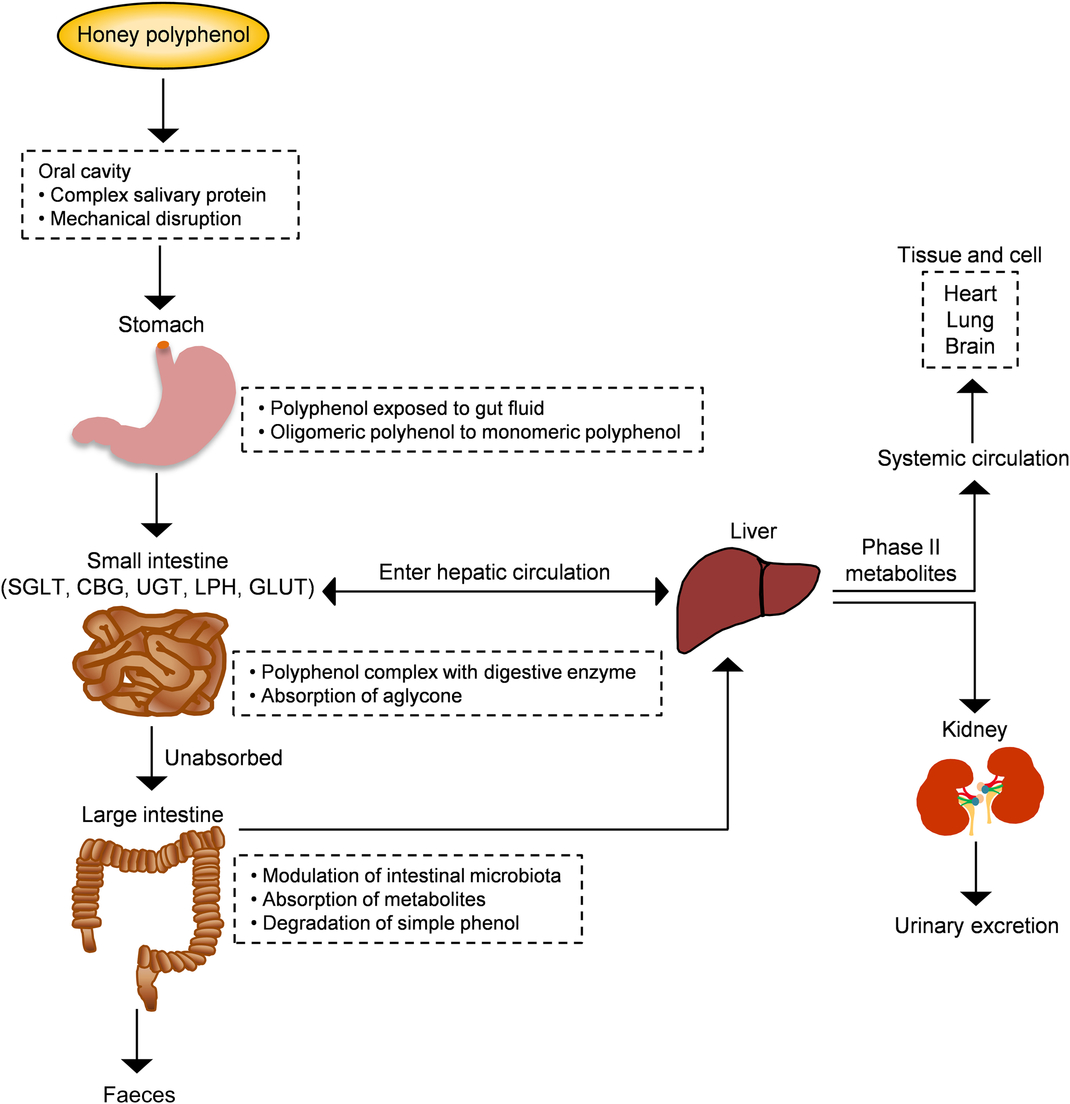
Fig. 4. Schematic depiction of the absorption and metabolism of honey polyphenols in the human gut. CBG, cytosolic β-glucosidase; LPH, lactase-phlorizin hydrolase; SGLT, sodium-glucose co-transporter; UGT, UDP-glucuronosyltransferase. For a colour figure, see the online version of the paper.
The sequence of absorption and quick elimination of phenolic compounds produces the final plasma concentration of oligomeric flavonoids of 1 µmol/l and flavanones of 5 µmol/l. In the case of phenolic acids, bioavailability is much lower due to the esterification process(Reference Manach, Williamson and Morand50 , Reference Manach, Scalbert and Morand51). Up to date, only one study investigated the bioavailability of buckwheat honey polyphenols in healthy human subjects. Two types of buckwheat honey at 1·5 mg/kg (containing 0·796 and 1·716 mg phenolic antioxidants per g) were supplemented in forty subjects. The total phenolic content along with the antioxidant and reducing capacities of plasma significantly increased 2 h after the honey supplementation and remained high for up to 6 h(Reference Schramm, Karim and Schrader52). This investigation supports that the phenolic compounds of honey are not only bioavailable but also exhibit attractive antioxidant activity for inducing defensive mechanisms against oxidative stress. In another study, bioaccessibility and bioavailability of bracatinga (Mimosa scabrella Bentham) honeydew honey were investigated after simulated in vitro digestion. The stability of phenolic constituents and minerals was the highest and, sometimes, increased further after in vitro digestion, while the antioxidant capacity was decreased. This finding suggests that honey components other than phenolic and mineral compounds have the ability to affect the antioxidant capacity(Reference Seraglio, Valese and Daguer53).
Studies on honey in cancer: mechanisms of chemoprevention
Numerous studies have examined the possible mechanisms by which honey exerts its chemoprevention and concluded that the preventive effects of honey depend on the presence of diverse antioxidant constituents as well as phenolic acids and flavonoids(Reference Porcza, Simms and Chopra48).
Diverse in vitro models have evaluated the efficiency of whole honey, flavonoid or phenolic extracts, or fractionated honey extracts on different types of cancer(Reference Afrin, Forbes-Hernandez and Gasparrini32 , Reference Porcza, Simms and Chopra48 , Reference Aliyu, Odunola and Farooq54 – Reference Aryappalli, Al-Qubaisi and Attoub56). Particular attention was given to the key mechanism of the anti-proliferative effect, induction of apoptosis, modulation of oxidative stress, as well as the anti-inflammatory, immune-modulatory and anti-metastatic effects (Fig. 5 and Table 2 (Reference Aliyu, Odunola and Farooq54 – Reference Aziz, Rady and Amer83)).
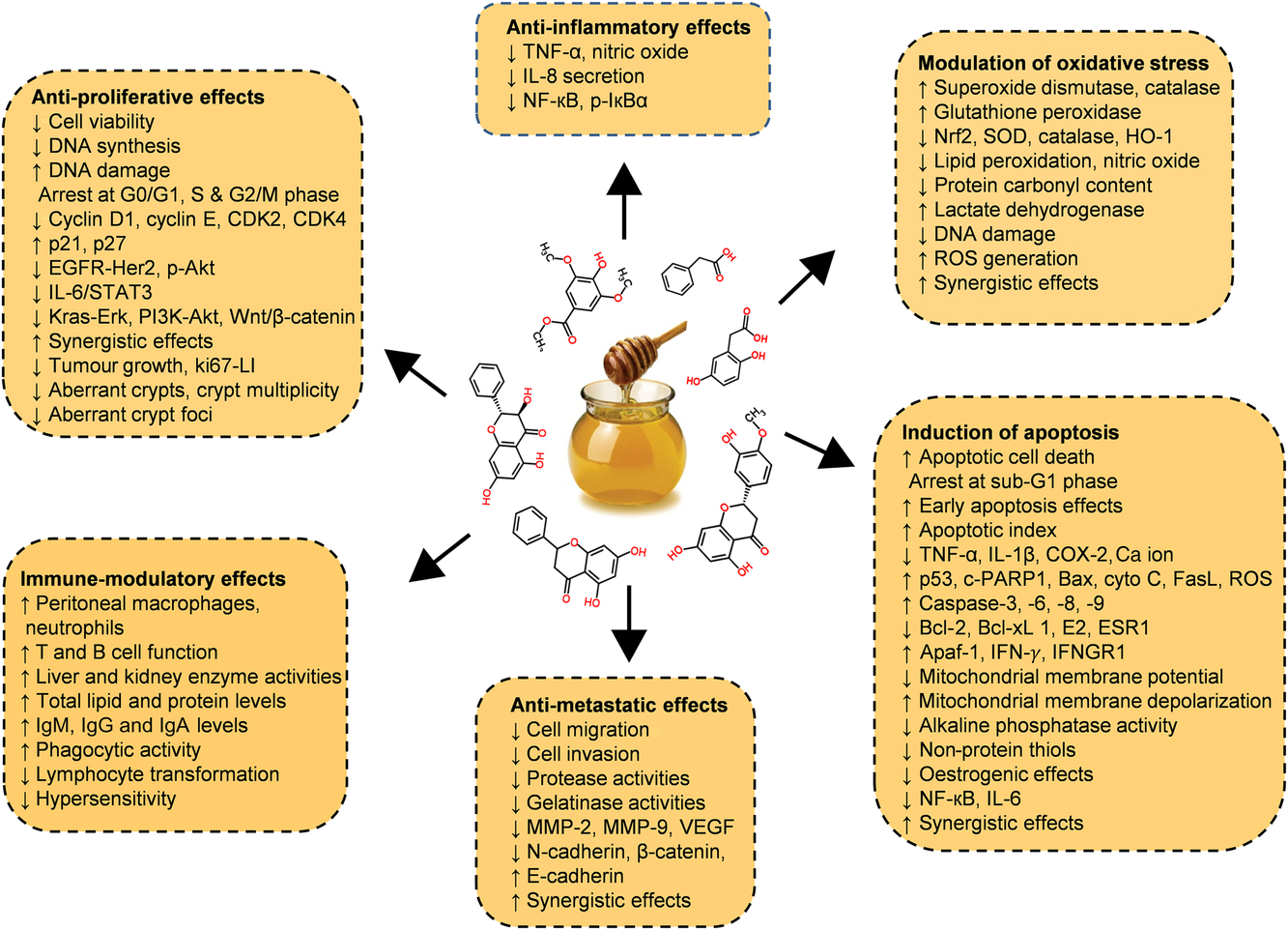
Fig. 5. Chemopreventive effects of honey against different types of cancer both in in vitro and in vivo models by targeting diverse mechanism of actions. Akt, protein kinase B; Apaf-1, apoptotic protease activating factor-1; Bax, Bcl-2 associated X protein; Bcl-2, B-cell lymphoma-2; CDK, cyclin-dependent kinase; COX-2, cyclo-oxygenase 2; c-PARP, cleaved poly (ADP-ribose) polymerase; cyto c, cytochrome c; EGFR, epidermal growth factor receptor; Erk, extracellular signal-regulated kinase; ESR1, oestrogen receptor 1; FasL, fatty acid synthetase ligand; HO-1, haeme oxygenase 1; IFN-γ, interferon-γ; IFNGR1, interferon-γ receptor 1; MMP, matrix metalloproteinase; Nrf2, nuclear related factor 2; p-IκBα, phosphorylated inhibitor of κB; ROS, reactive oxygen species; SOD, superoxide dismutase; STAT3, signal transducer and activator of transcription 3; VEGF, vascular endothelial growth factor; Wnt, wingless-type. For a colour figure, see the online version of the paper.
Table 2. Inhibitory effects of honey or its extract on cancer cell lines in vitro
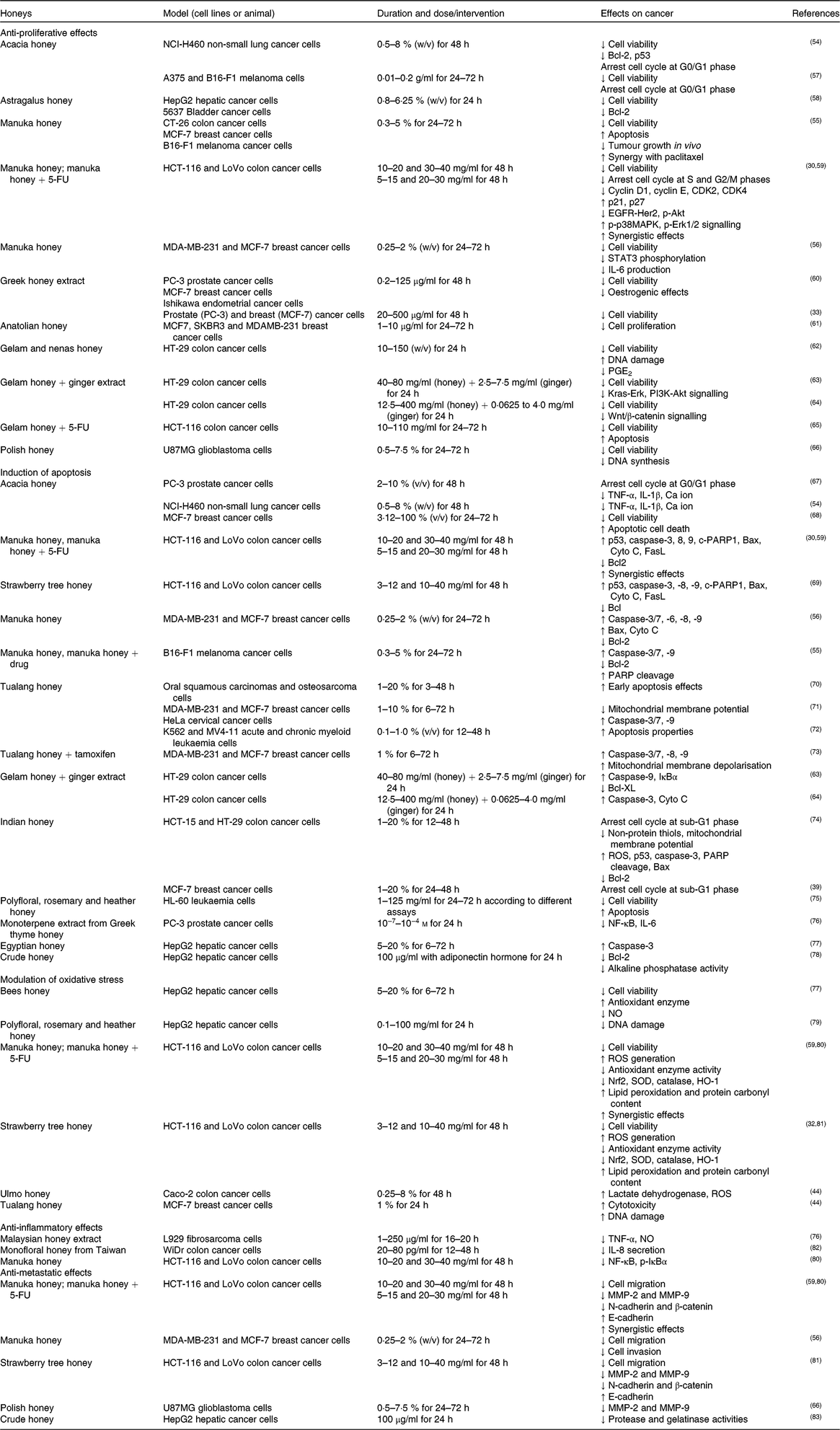
5-FU, 5-fluorouracil; Akt, protein kinase B; CDK, cyclin-dependent kinase; HER2, human epidermal growth factor receptor 2; MCF-7, Michigan Cancer Foundation-7; MMP, matrix metalloproteinase; p-Akt, phosphorylated protein kinase B; p-IκBα, phosphorylated inhibitor of κB; p-p38MAPK, phosphorylated p38 mitogen-activated protein kinase; ROS, reactive oxygen species; SOD, superoxide dismutase.
Anti-proliferative effects
The anti-proliferative effect of acacia honey was evaluated on non-small lung cancer (NCI-H460)(Reference Aliyu, Odunola and Farooq54) and melanoma (A375 and B16-F1) cell lines(Reference Pichichero, Cicconi and Mattei57). Honey treatment arrested the cell cycle at the G0/G1 phase and decreased the mRNA levels of B-cell lymphoma-2 (Bcl-2) and p53(Reference Aliyu, Odunola and Farooq54 , Reference Pichichero, Cicconi and Mattei57). The authors concluded that chrysin was the main phenolic compound responsible for the anti-proliferative effect(Reference Pichichero, Cicconi and Mattei57). Furthermore, Astragalus honey treatment decreased the viability of human hepatic (HepG2) and bladder (5637) carcinoma cells(Reference Sadeghi-Aliabadi, Hamzeh and Mirian58), where mRNA levels of only Bcl-2 were decreased but no significant changes were observed in p53 mRNA(Reference Sadeghi-Aliabadi, Hamzeh and Mirian58).
The anti-proliferative effect of manuka honey was observed on a panel of cancer cells such as colon (CT-26, HCT-116 and LoVo), breast (MDA-MB-231 and MCF-7 (Michigan Cancer Foundation-7)) and melanoma (B16-F1)(Reference Afrin, Giampieri and Gasparrini30 , Reference Fernandez-Cabezudo, El-Kharrag and Torab55 , Reference Aryappalli, Al-Qubaisi and Attoub56) and found to be time and dose dependent. The anti-proliferative effect was associated with cell cycle arrest at the S and G2/M phases due to alterations in cell cycle regulatory genes such as p21, p27, cyclin-dependent kinase (CDK) 2, CDK4, cyclin D1 and cyclin E. Moreover, it was reported that manuka honey suppressed the expression of oncogenic signalling pathways such as epidermal growth factor receptor (EGFR), human epidermal growth factor receptor (HER2), phosphorylated protein kinase B (p-Akt) and IL-6/signal transducer and activator of transcription (IL-6/STAT3), while it increased the expression of phosphorylated p38 mitogen-activated protein kinase (p-p38MAPK) and phosphorylated extracellular signal-regulated kinase 1/2 (p-Erk1/2)(Reference Afrin, Giampieri and Gasparrini30 , Reference Aryappalli, Al-Qubaisi and Attoub56). The anti-proliferative effects of manuka honey on HCT-116 and LoVo cancer cells significantly increased after this honey was combined with 5-fluorouracil (5-FU), while the concentration of 5-FU was lower compared with a single dose(Reference Afrin, Giampieri and Forbes-Hernandez59). Greek honey extract (thyme, pine and fir) significantly decreased the viability of breast (MCF-7), prostate (PC-3) and endometrial (Ishikawa) cancer cells(Reference Spilioti, Jaakkola and Tolonen33 , Reference Tsiapara, Jaakkola and Chinou60) whereas thyme honey inhibited MCF-7 cell progression by suppressing oestrogenic effects(Reference Tsiapara, Jaakkola and Chinou60). Anatolian honey with varied botanical origin (chestnut, pine and cedar) induced stronger inhibitory effects on different breast cancer cells, such as MCF-7, SKBR3 and MDA-MB-231, in a time- and dose-dependent manner(Reference Seyhan, Yılmaz and Timirci-Kahraman61).
In HT-29 cancer cells, gelam honey and nenas honey inhibited cell proliferation by increasing DNA damage and suppressing several inflammation markers (PGE2; PGE2) production(Reference Wen, Hussein and Abdullah62). In addition, the anti-proliferative effects of gelam honey increased when it was combined with ginger extract compared with single compounds(Reference Tahir, Sani and Murad63 , Reference Wee, Morad and Aan64). This co-treatment significantly suppressed the expression of Kirsten rat sarcoma virus oncogene homolog (Kras)-Erk, phosphatidylinositol 3-kinase (PI3K)-Akt, mammalian target of rapamycin (mTOR) and wingless-type (Wnt)/β-catenin pathways in HT-29 colon cancer cells(Reference Tahir, Sani and Murad63 , Reference Wee, Morad and Aan64). Synergistic effects were observed when this honey was combined with 5-FU via enhancement of pro-apoptotic effects on HCT-116 cancer cells compared with 5-FU alone(Reference Hakim, Alias and Makpol65).
Finally, in glioblastoma (U87MG) cells, Polish honey decreased cell viability by reducing DNA synthesis and diastase activity, whereas polyphenol and Cd content had a significant impact on its anti-proliferative effects(Reference Moskwa, Borawska and Markiewicz-Zukowska66).
In an azoxymethane-induced rat model of colon cancer, kelulut honey inhibited aberrant crypt formation while concurrently normalising liver and kidney functions and blood parameters(Reference Yazan, Zali and Shyfiq84). In a murine Ehrlich ascites carcinoma model, Indian honey and its phenolic constituent (eugenol) significantly decreased tumour growth(Reference Jaganathan, Mondhe and Wani85), while in leukaemia cancer they did not induce any significant changes(Reference Jaganathan, Mondhe and Wani86). Bee honey protected against diethylnitrosamine-induced rat hepatocarcinogenesis by reducing weight loss, tumour size and inflammatory responses(Reference El-kott, Kandeel and El-Aziz87). These effects correlated with normalisation of the levels of proliferation markers, like proliferating cell nuclear antigen (PCNA) and p53 in liver tissue(Reference El-kott, Kandeel and El-Aziz87).
Induction of apoptosis
In lung (NCI-H460)(Reference Aliyu, Odunola and Farooq54), prostate (PC-3)(Reference Aliyu, Odunola and Farooq67) and breast (MCF-7)(Reference Salleh, Eshak and Ismail68) cancer cell lines, acacia honey induced apoptosis by arresting the cell cycle at the G0/G1 phase and increasing the production of immune-modulatory cytokines such as TNF-α and IL-1β, which induced Ca ion release from the endoplasmic reticulum(Reference Aliyu, Odunola and Farooq54 , Reference Aliyu, Odunola and Farooq67). Manuka and strawberry tree honey induced apoptotic death of HCT-116 and LoVo cells by increasing p53, cleaved poly (ADP-ribose) polymerase (c-PARP) and caspase-3 expression. Additionally elevated mRNA levels of both intrinsic and extrinsic apoptotic markers such as caspase-8, caspase-9, Bcl-2-associated X protein (Bax), fatty acid synthetase (Fas) ligand (FasL) and cytochrome C (Cyto C) were also observed after manuka honey treatment(Reference Afrin, Giampieri and Gasparrini30 , Reference Afrin, Giampieri and Cianciosi69). On the same cell lines, manuka honey induced synergistic effects when used with lower concentrations of a chemotherapeutic drug (5-FU)(Reference Afrin, Giampieri and Forbes-Hernandez59). Additionally, in MDA-MB-231 cells, manuka honey increased the enzymic activity of the caspase cascade (3/7, 6, 8 and 9) which correlated with increased Bax and decreased Bcl-2 expression, while in MCF-7 cells it induced only caspase-6 and caspase-9 activation(Reference Aryappalli, Al-Qubaisi and Attoub56). Concurrently, manuka honey treatment translocated Cyto C from mitochondria to cytosol and Bax from cytosol to mitochondria(Reference Aryappalli, Al-Qubaisi and Attoub56). In murine melanoma B16-F1 cells, manuka honey activated the mitochondria-dependent apoptotic pathway by increasing caspase-3/7 and caspase-9 enzyme activities as well as suppressing Bcl-2 expression, increasing c-PARP and DNA fragmentation(Reference Fernandez-Cabezudo, El-Kharrag and Torab55). The same group of researchers also reported that intravenous administration of manuka honey reduced tumour size and increased caspase-3 in a syngeneic melanoma model, additionally improving the survival rate of paclitaxel-treated mice by inducing parallel protective effects(Reference Fernandez-Cabezudo, El-Kharrag and Torab55).
Flow cytometric analysis revealed that tualang honey induced early apoptosis in oral squamous carcinomas and osteosarcoma cells(Reference Ghashm, Othman and Khattak70) and early and late apoptosis effects in breast (MCF-7 and MDA-MB-231) and cervical (HeLa) cancer cells(Reference Fauzi, Norazmi and Yaacob71). Tualang honey activated the mitochondrial apoptotic pathway by increasing caspase-3/7 and caspase-9 and decreased mitochondrial membrane potential(Reference Fauzi, Norazmi and Yaacob71). Tualang honey promoted the apoptotic activity of tamoxifen in MDA-MB-231 and MCF-7 cells by increasing caspase-3/7, caspase-8 and caspase-9 activity, and mitochondrial membrane depolarisation compared with tamoxifen alone(Reference Yaacob, Nengsih and Norazmi73). Signs of apoptosis, such as cytoplasmic blebs followed by formation of apoptotic bodies and rounded shape of acute and chronic myeloid leukaemia (K562 and MV4-11) cells, were also observed after this honey treatment(Reference Man, Khuzaimi and Hassan72).
The early apoptotic effects of gelam honey were enhanced when used in combination with ginger extract by increasing mRNA levels of caspase-3 and -9, and Cyto C, and decreasing Bcl-XL in HT-29 cells(Reference Tahir, Sani and Murad63 , Reference Wee, Morad and Aan64). Treatment of pure unfractionated Indian honey indicated apoptosis effects in HCT-15 and HT-29 colon and MCF-7 breast cancer cells by arresting the cell cycle at the sub-G1 phase(Reference Jaganathan, Mandal and Jana39 , Reference Jaganathan and Mandal74) and reducing intracellular non-protein thiols, concomitantly decreasing matrix metalloproteinase (MMP) due to an increased generation of reactive oxygen species (ROS)(Reference Jaganathan and Mandal74). Additionally, this honey increased p53, caspase-3, c-PARP and Bax, and decreased Bcl-2 protein expression in a time-dependent manner(Reference Jaganathan and Mandal74). Three types of Spanish honey from different floral origins such as rosemary, heather and polyfloral honey, induced ROS-independent apoptotic effects in leukaemia (HL-60) cells which were strongly co-related with their polyphenol and floral origin(Reference Morales and Haza75). Monoterpene extract from Greek thyme honey induced apoptotic cell death in PC-3 prostate cancer cells by suppressing NF-κ-light-chain-enhancer of activated B cells (NF-κB) phosphorylation and IL-6 secretion(Reference Kassim, Achoui and Mustafa76). Egyptian honey treatment significantly suppressed HepG2 cell viability by apoptotic activation with high caspase-3 levels(Reference Hassan, Mabrouk and Shehata77). Additionally, the HepG2 cell survival rate decreased when crude honey combined with adiponectin hormone induced apoptosis by decreasing Bcl-2 levels and reducing alkaline phosphatase activity(Reference Hanaa and Shaymaa78).
Modulation of oxidative stress
Honey is a good source of natural antioxidants whose activity is mainly due to the phenolic compounds present in honey, as we discussed earlier (Table 1). Other components such as amino acids, proteins, vitamins and carotenoid derivatives present in honey can also contribute to its antioxidant activity. The botanical and geographical origin of honey as well as climate conditions contribute to the variations in the antioxidant activity of different honeys. Several studies have reported a strong correlation between the total polyphenol and flavonoid contents and the antioxidant capacity of honey(Reference Alvarez-Suarez, Tulipani and Díaz4 , Reference Moniruzzaman, Sulaiman and Khalil88). The antioxidant activity of honey is accredited to the ability of its bioactive compounds to scavenge or reduce the formation of free radicals, along with the improvement of mitochondrial functionality and the inhibition of DNA damage and lipid peroxidation(Reference Alvarez-Suarez, Giampieri and Cordero31).
Bee honey was shown to inhibit the growth of HepG2 cells in vitro by improving the antioxidant status which could prevent the development of cancer cells, and by inducing apoptotic death(Reference Hassan, Mabrouk and Shehata77). Moreover, rosemary, heather and heterofloral honeys protected HepG2 cells from dietary mutagen-induced DNA damage(Reference Haza and Morales79). Increased ROS generation was observed in HCT-116 and LoVo cells after treatment with manuka honey and strawberry tree honey(Reference Afrin, Forbes-Hernandez and Gasparrini32 , Reference Afrin, Giampieri and Gasparrini80). Additionally, these honeys initiated oxidative stress associated with cancer cell death by: (i) decreasing antioxidant enzyme activities such as glutathione peroxidase, glutathione peroxidase, glutathione reductase, superoxide dismutase (SOD) and catalase; (ii) concomitantly suppressing the expression of nuclear-related factor 2 (Nrf2), SOD, catalase and haeme oxygenase 1 (HO-1); (iii) increasing the damage of cellular biomolecules (lipid, protein and DNA); and (iv) disrupting mitochondrial respiration and glycolysis function(Reference Afrin, Giampieri and Gasparrini80 , Reference Afrin, Forbes-Hernández and Cianciosi81). Additive effects were also observed when manuka honey was combined with 5-FU(Reference Afrin, Giampieri and Forbes-Hernandez59).
Ulmo honey is a good source of several volatile and non-volatile compounds, which induced high cytotoxicity to Caco-2 colon cancer cells by releasing glactate dehydrogenase and increasing intracellular ROS levels in a dose-dependent way(Reference Acevedo, Torres and Oomah44). Tualang honey potentiated the cytotoxic and genotoxic effects of 4-hydroxytamoxifen in MCF-7 breast cancer cells by increasing DNA damage and cell death. However, in non-cancer cells, this honey acted against 4-hydroxytamoxifen-induced toxicity through increasing DNA repair mechanisms(Reference Yaacob, Nengsih and Norazmi73).
Anti-inflammatory effects
Several studies have evaluated the anti-inflammatory effects of honey in different disease models(Reference Badolato, Carullo and Cione9 , Reference Porcza, Simms and Chopra48). Although inflammation is the key step for the initiation of carcinogenesis, only a few studies have addressed the anti-inflammatory effects of honey in fibrosarcoma(Reference Kassim, Achoui and Mustafa76) and colon cancer cells(Reference Afrin, Giampieri and Gasparrini80 , Reference Liu, Ye and Lin82). Flavonoid and phenolic acid extracts from Malaysian honey induced anti-inflammatory effects in L929 fibrosarcoma cells by decreasing TNF-α-induced cytotoxicity, and interferon-γ and lipopolysaccharide-induced NO levels(Reference Kassim, Achoui and Mustafa76). Furthermore, in WiDr, HCT-116 and LoVo colon cancer cells, monofloral honey from Taiwan and manuka honey from New Zealand inhibited inflammation through the suppression of IL-8 activity(Reference Liu, Ye and Lin82), and NF-κB and phosphorylated inhibitor of κB (p-IκBα) expression(Reference Afrin, Giampieri and Gasparrini80).
Anti-metastatic effects
Manuka and strawberry tree honey inhibited the migration and invasion ability of HCT-116 and LoVo human colon(Reference Afrin, Giampieri and Gasparrini80 , Reference Afrin, Forbes-Hernández and Cianciosi81) and MDA-MB-231 and MCF-7 breast cancer(Reference Aryappalli, Al-Qubaisi and Attoub56) cells in a time- and dose-dependent manner. These effects were mainly related to the inhibition of MMP-2 and MMP-9 expression, as well as a decrease in the expression of N-cadherin and β-catenin, and an increase in E-cadherin expression(Reference Afrin, Giampieri and Gasparrini80 , Reference Afrin, Forbes-Hernández and Cianciosi81). Interestingly, manuka honey increased the anti-migration and anti-invasion ability of therapeutic drugs compared with single compounds(Reference Afrin, Giampieri and Forbes-Hernandez59). Polyphenol-rich Polish honey was shown to inhibit metastasis of U87MG cells by decreasing the activity and expression of MMP-2 and MMP-9 in a dose-dependent manner(Reference Moskwa, Borawska and Markiewicz-Zukowska66). Decreased protease and gelatinase activities were observed in HepG2 cells after treatment with crude honey(Reference Aziz, Rady and Amer83).
Pre-clinical studies on honey in cancer
Studies of the anti-cancer activities of honey in preclinical models are limited (Fig. 4 and Table 3 (Reference Fernandez-Cabezudo, El-Kharrag and Torab55 , Reference Yazan, Zali and Shyfiq84 , Reference Jaganathan, Mondhe and Wani85 , Reference El-kott, Kandeel and El-Aziz87 , Reference Fukuda, Kobayashi and Hirono89 – Reference Hegazi, Abdel-Rahman and Abd-Allah96)).
Table 3. Preclinical studies evaluating the effect of honey in different cancer models

Apaf-1, apoptotic protease activating factor-1; Bax, Bcl-2 associated X protein; Bcl-2, B-cell lymphoma-2; COX-2, cyclo-oxygenase 2; DMBA, 7,12-dimethylbenzeneanthracene; IFNGR1, interferon-γ receptor 1; IFN-γ, interferon-γ; MBT-2, murine bladder cancer cell line; MNU, 1-methyl-1-nitrosourea; PCNA, proliferating cell nuclear antigen; ROS, reactive oxygen species; VEGF, vascular endothelial growth factor.
Tualang honey inhibited the growth of 7,12-dimethylbenzeneanthracene (DMBA)-induced mammary tumours in Sprague–Dawley rats. Animals treated with oral tualang honey starting the day after DMBA administration for 150 d had delayed tumour development, multiplicity, weights and volumes compared with control animals. Vascular endothelial growth factor (VEGF), a pro-angiogenic factor, was significantly lowered in honey-treated animals(Reference Kadir, Sulaiman and Yahya90).
Another study reported that tualang and manuka honeys were able to slow down tumour progression in carcinogen 1-methyl-1-nitrosourea (MNU)-induced breast cancer in Sprague–Dawley rats(Reference Ahmed, Sulaiman and Othman91). In this study, treatments were started after the animals developed a palpable tumour. The percentage reduction in tumour growth was significant with both tualang (71 %) and manuka (57 %) honeys. The expression levels of apoptotic protease activating factor-1 (Apaf-1) and pro-apoptotic protein, caspase-9, increased in tumour specimens of honey-treated rats. Both honeys potentiated the immune system of animals, which was evident from the increased expression levels of interferon-γ and IFNGR1. The serum levels of oestrogen and oestrogen receptor 1 were significantly reduced in treated animals compared with controls. Thus, tualang and manuka honeys administered orally exhibit anti-cancer activities by modulating the immune system and activating the intrinsic apoptotic pathway(Reference Ahmed, Sulaiman and Othman91). In a separate study, oral administration of kelulut honey reduced the number of azoxymethane-induced aberrant crypt foci and crypt multiplicity in Sprague–Dawley rats, indicating that it has chemopreventive properties. Honey was administered orally, twice daily for 2 weeks, after induction with azoxymethane(Reference Yazan, Zali and Shyfiq84). The authors tried to mimic the traditional human dosage of honey, which they reported as twice daily, and based on density of honey the dose conversions were made. However, this study did not give much insight on the mechanism. In another study, aloe vera increased the chemopreventive effects of honey in an in vivo Walker carcinoma model by reducing tumour growth and Ki67-Li expression, and increasing tumour apoptosis(Reference Tomasin and Cintra Gomes-Marcondes94). The study administered a combination of oral honey and aloe vera solution to tumour-bearing mice, but failed to give discernment on whether the activity was synergistic or not.
Dietary supplementation with Egyptian honey and Nigella sativa induced higher protection against MNU-triggered oxidative damage and colon adenocarcinoma in Sprague–Dawley rats via reduction of lipid peroxidation and NO levels. Oral treatments began 1 week after MNU induction and the authors reported that honey and N. sativa administered together gave 100 % protection compared with 80 % protection by N. sativa alone(Reference Mabrouk, Moselhy and Zohny97). Bee honey was also found to prevent liver carcinogenesis in diethylnitrosamine (DEN)-administered rats. The authors used 2 g honey per rat per d orally for 6 months starting 1 week after DEN and showed that honey had a protective effect against inflammation and DEN-induced carcinogenesis(Reference El-kott, Kandeel and El-Aziz87). Such a concentration of honey given for a long period of time could have confounded the findings due to the carbohydrates and a control of sugar intake should have been included.
In a separate study, pre-treatment with Egyptian honey was shown to inhibit the growth of Ehrlich ascites tumours in mice by increasing cell recruitment and enhancing the function of T and B cells as well as macrophages(Reference Attia, Gabry and El-Shaikh95). The authors reported that this preventive peroral treatment 4 weeks before tumour inoculation also normalised liver and kidney functions in tumour-bearing mice. In a related study, coriander honey improved the immune status of Ehrlich ascites carcinoma-bearing mice by enhancing macrophage phagocytic activity and immunoglobulin levels and maintaining normal kidney and liver enzyme activities, leading to enhanced survival(Reference Hegazi, Abdel-Rahman and Abd-Allah96). The precise mechanism by which honey affects the immune status in this model is unknown.
A study on the effect of various bee products in murine tumour models demonstrated a predominant anti-metastatic effect. The study was done in two transplantable, syngeneic animal models, a murine mammary carcinoma and an anaplastic colon adenocarcinoma in rats(Reference Orsolić, Knezević and Sver92). Treatment with oral honey led to a pronounced decrease in lung metastasis in both tumour models when given daily, starting 10 d before tumour inoculation. Surprisingly, however, administration of honey after tumour implantation appeared to enhance metastasis. This suggests that honey and its polyphenolic components stimulate the host’s anti-tumour defence. Furthermore, intravenous, but not intraperitoneal or subcutaneous, administration of royal jelly had a significant anti-metastatic effect. This study also reported on the anti-tumour and anti-metastatic effects of bee venom, water-soluble derivatives and related polyphenolic compounds of propolis(Reference Orsolić, Knezević and Sver92).
Jungle honey was reported to enhance anti-tumour immunity in mice injected with Lewis lung carcinoma/2 cells. Decreased tumour incidence in honey-treated mice (1 mg per mouse per d intraperitoneally starting 7 d before tumour inoculation) correlated with an increased chemotactic response by neutrophils and ROS production by activated neutrophils(Reference Fukuda, Kobayashi and Hirono89). In another study, intraperitoneal administration of 25 % (v/v) bee honey 1 d after tumour inoculation significantly inhibited the growth of Ehrlich ascites carcinoma in mice. The anti-tumour effect of honey against Ehrlich ascites was attributed to the phenolic content and its antioxidant ability(Reference Jaganathan, Mondhe and Wani85). Insights into the mechanisms that underlie these effects await further, more detailed, studies.
In a therapeutic murine melanoma model, intravenous administration of manuka honey was shown to increase the survival of B16-F1 tumour-bearing mice. Animals treated with honey alone or in combination with paclitaxel, 11 d post-tumour implantation, showed a 33 and 66 % reduction in tumour growth, respectively(Reference Fernandez-Cabezudo, El-Kharrag and Torab55). Immunohistochemical examination of the tumours showed an increased number of caspase-3-positive cells in honey or paclitaxel-treated groups compared with control animals. The number of apoptotic cells was highest in the animals treated with manuka honey plus paclitaxel(Reference Fernandez-Cabezudo, El-Kharrag and Torab55). Interestingly, overall survival of animals treated with the combination of manuka honey and paclitaxel was significantly higher than animals treated with the honey or paclitaxel alone. This suggested that combining manuka honey with paclitaxel improves efficacy of the treatment while decreasing the toxic side effects of the chemotherapeutic drug(Reference Fernandez-Cabezudo, El-Kharrag and Torab55).
Finally, bee honey was investigated for its tumour-inhibitory effect in the MBT-2 bladder cancer model in C3H/He mice. This study reported that 6 and 12 % solutions of honey were effective in reducing the tumour volume when injected into the tumour lesions (intralesional). Moreover, a 50 % solution administered perorally was able to inhibit tumour growth(Reference Swellam, Miyanaga and Onozawa93). However, no mechanism for this anti-tumour effect was described.
Overall, these studies corroborate the anti-cancer potential of honey in preventative and therapeutic models. However, there is a lack of well-controlled preclinical studies on its immunomodulatory and chemopreventive effects at physiologically relevant concentrations. There is also a need to standardise the honeys studied and include proper sugar controls in experiments. Another shortcoming of the previous studies is the paucity of data on the effect of combination treatment with honey and standard chemotherapeutic drugs. Despite several studies investigating the effect of oral honey, there are few promising reports available on its potential effects when administered parenterally, an area of investigation that could be further explored. Moreover, mechanistic studies are needed to identify the cellular targets of honey and investigate its effect on specific cancer-related signalling pathways.
A note on clinical studies on honey in cancer
Honey has been used as a complementary medicine and is believed to improve the quality of life of cancer patients. However, there is a dearth of clinical trials testing the potential utility of honey in cancer patients. One of the few areas in which good evidence has been obtained is in the capacity of orally administered honey to ameliorate radiation-induced mucositis in patients with head and neck cancer. Several recent studies conducted in different centres have confirmed that different types of honey, including manuka and thyme, can alleviate radiation-induced mucositis in patients treated for head and neck cancers(Reference Rao, Hegde and Rao98 – Reference Charalambous, Raftopoulos and Paikousis101). Importantly, no effect on actual cancer growth was reported in these studies. In a meta-analysis of randomised clinical trials, it was concluded that honey can effectively reduce the severity of chemotherapy-induced mucositis(Reference Xu, Xia and Sun102). In contrast, a randomised clinical trial testing the effectiveness of manuka honey on radiation-induced esophagitis reported that it was not superior to the standard supportive care(Reference Fogh, Deshmukh and Berk103). In a separate double-blind randomised trial involving fifty-two subjects, cancer-related fatigue was reduced in patients who received 5 ml (twice daily for 4 weeks) of processed honey and royal jelly(Reference Mofid, Rezaeizadeh and Termos104). In another double-blind, randomised, placebo-controlled study in 107 patients receiving chemotherapy for acute myeloid leukaemia, administration of honey and ardeh (sesame paste) ameliorated gastrointestinal complications, neutropenia and reduced fever(Reference Tomasin and Cintra Gomes-Marcondes94). Moreover, a randomised cross-over clinical trial in forty children, aged 2·5–10 years, with acute lymphoblastic leukaemia reported a significant decrease in febrile neutropenia episodes and improved levels of Hb after honey (raw clover honey) consumption(Reference Mabrouk, Moselhy and Zohny97). Overall, there is good evidence for the beneficial use of honey in reducing chemotherapy/radiotherapy-induced toxic side effects, including fatigue, mucositits, neutropenia and gastrointestinal complications. Furthermore, a recent study reported that honey could also have a direct effect on growth of breast cancer. In a randomised controlled trial on patients with hormone receptor-positive breast cancer, combining tualang honey with the aromatase inhibitor anastrozole as adjuvant endocrine therapy reduced background parenchymal enhancement, a correlate of cancer recurrence, more effectively than treatment with anastrozole alone (42 % compared with 10 % reduction, respectively)(Reference Hizan, Hassan and Haron105). These encouraging early findings should promote further interest in conducting well-controlled trials to directly evaluate the potenial utility of honey as an adjuvant treatment in different types of cancer.
Anti-cancer effects of major phenolic/flavonoid compounds in honey
The composition of honey varies depending on the source and geographical origin. For example, the principal flavonoids in manuka honey are pinobanksin, pinocembrin, luteolin and chrysin accounting for about 61 % of the total flavonoid content, with other flavonoids like quercetin, 8-methoxykaempferol, isorhamnetin, kaempferol and galangin found in lesser amounts(Reference Chan, Deadman and Manley-Harris106). In tualang honey, the major flavonoids are catechin, kaempferol, naringenin, luteolin and apigenin(Reference Khalil, Alam and Moniruzzaman107 , Reference Ahmed and Othman108). The anti-cancer effects of the major flavonoids of honey (Table 4)(Reference Czyżewska, Siemionow and Zaręba109 – Reference Wang, Yang and Li153) will be discussed in this section.
Table 4. Effect of flavonoids on different pathways in cancer

5-FU, 5-fluorouracil; Akt, protein kinase B; AP-1, activator protein 1; ATF, activating transcription factor; Bax, Bcl-2 associated X protein; Bcl-2, B-cell lymphoma-2; EGFR, epidermal growth factor receptor; eIF2α, eukaryotic initiation factor 2α; EMT, epithelial-mesenchymal transition; Erk, extracellular-signal-regulated kinase; FAK, focal adhesion kinase; Fas, fatty acid synthetase; FasL, fatty acid synthetase ligand; HIF-1α, hypoxia-inducible factor-1α; HO-1, haeme oxygenase 1; JNK, c-Jun N-terminal kinase; MAPK, mitogen-activated protein kinase; MCF-7, Michigan Cancer Foundation-7; MG, methylglyoxal; Notch1, Notch homolog 1; Nrf2, nuclear related factor 2; PC-3, prostate cancer cell line; PERK, protein kinase RNA-like endoplasmic reticulum kinase; p-Erk, phosphorylated extracellular-signal-regulated kinase; PI3K, phosphatidylinositol 3-kinase; PTEN, phosphatase and tensin homolog; ROS, reactive oxygen species; STAT3, signal transducer and activator of transcription 3; TGF-β, transforming growth factor β; VEGF, vascular endothelial growth factor; Wnt, wingless-type.
Pinocembrin and pinobanksin
Pinocembrin is a flavonoid present in honey and various plants of the Piperaceae, Lauraceae and Asteraceae families and reported to have various pharmacological properties including anti-bacterial, antioxidant, anti-cancer and anti-mutagenic activities(Reference Rasul, Millimouno and Ali Eltayb154 – Reference Hsu, Yu and Yen156). Pinocembrin induced Bax-dependent apoptosis in HCT-116 colon cancer cells(Reference Kumar, Nair and Hema157). The proapoptotic activity of various polyphenols (caffeic acid, chrysin, galangin, ferulic acid, pinocembrin and p-coumaric acid) was studied in CAL-27 (human tongue squamous cell carcinoma) cells. Galangin was the most potent among the group with a half maximal inhibitory concentration (IC50) of 44·5 μg/ml, followed by chrysin (54·1 μg/ml), ferulic acid (99·6 μg/ml), caffeic acid (130·3 μg/ml), pinocembrin (135·2 μg/ml) and p-coumaric acid (139·2 μg/ml). Polyphenols were able to induce tumour apoptosis through mitochondrial and death receptor pathways(Reference Czyżewska, Siemionow and Zaręba109). Pinocembrin inhibited metastasis of retinoblastoma cells (Y-79 cells) through the inhibition of αvβ3 integrin receptor and the focal adhesion kinase (FAK)/p38α/NF-κB signalling pathway, thereby decreasing the expression of MMP-2 and MMP-9. Pinocembrin inhibited the transforming growth factor β (TGF-β1)-induced invasion and migration of Y-79 cells, increased E-cadherin, and decreased vimentin and N-cadherin levels(Reference Chen, Shi and Chien110). Pinocembrin also had an anti-proliferative and apoptotic effect on androgen-sensitive (LNCaP) and androgen-independent (PC3 and DU-145) prostate cancer cell lines through the disruption of MMP and arrest of cell cycle at the S and G2/M phases(Reference Chen, Rasul and Zhao111). Pinobanksin and its derivatives have been reported to have antioxidant activity and induce apoptosis in B-cell lymphoma cell lines through a loss of mitochondrial membrane potential and activation of caspases. In this study, Sonoran propolis was studied for apoptotic activity and its chemical components were identified. Eighteen flavonoids were characterised, with pinobanksin and its ester derivatives, pinocembrin and chrysin, identified as the major components. The IC50 of pinobanksin was found to be 52·1 μm whereas pinocembrin did not show any anti-proliferative effects(Reference Alday, Valencia and Carreño112).
Chrysin
Chrysin has been reported to have efficacy against various types of cancers in vitro and in vivo. In a recent study, chrysin inhibited the growth of B16-F10 melanoma cells in vitro by inducing cell cycle arrest and apoptosis(Reference Sassi, Maatouk and Bzéouich113). In addition, oral administration of chrysin to B16-F10-implanted mice was shown to decrease tumour growth significantly. This was associated with an enhanced anti-tumour activity of macrophages, natural killer cells and cytotoxic T lymphocytes(Reference Sassi, Maatouk and Bzéouich113). However, the mechanism(s) for chrysin-induced immune system enhancements remains to be elucidated. A combination of chrysin and silibinin had a synergistic anti-proliferative effect on T47D breast cancer cells which was partly due to down-regulation of cyclin D1 and hTERT genes(Reference Maasomi, Soltanahmadi and Dadashpour114). A comparative study on human prostate cancer cells (PC-3) showed that the inhibitory concentrations of honey and chrysin were 2·5 % and 24·5 µm, after 48 h, and 1·8 % and 8·5 µm after 72 h, respectively(Reference Samarghandian, Afshari and Davoodi158).
Notch homolog 1 (Notch1), a tumour suppressor and modulator of apoptosis, was activated by chrysin in a xenograft model of anaplastic thyroid carcinoma. In these carcinoma cell lines (HTh7 and KAT18), chrysin increased the expression of both Notch1 and its downstream target, Hes1(Reference Yu, Phan and Patel115). Chrysin treatment suppressed tumour growth by 59 % and elevated levels of c-PARP and Notch1 were observed in tumour tissues of mice treated with chrysin(Reference Yu, Phan and Patel115). In colorectal cancer cells (SW48, SW480 and SW620), chrysin increased autophagy-related markers, light chain 3 (LC3)-II, and induced ROS generation which in turn inhibited the Akt/mTOR pathway leading to decreased cell viability(Reference Lin, Chen and Hsiang116). Furthermore, the accumulation of ROS was associated with the anti-tumour effects of chrysin in bladder cancer cells. Chrysin induced apoptosis in these cells through the intrinsic apoptotic pathway, inhibition of p-STAT3 and induction of endoplasmic reticulum stress. Activation of protein kinase RNA-like endoplasmic reticulum kinase (PERK), eukaryotic initiation factor 2α (eIF2α) and activation of transcription factor 4 (ATF4) were also observed(Reference Xu, Tong and Ying117). A similar mechanism of action has been reported for chrysin-induced cell death in prostate cancer cells(Reference Ryu, Lim and Bazer118). In gliobastoma cells (U251 and U87 cells), chrysin suppressed haeme oxygenase 1 (HO-1) and NAD(P)H quinine oxidoreductase-1 by deactivation of the Nrf2 signalling pathway. Chrysin also inhibited tumour growth in U87 xenograft-bearing nude mice and these tumours exhibited decreased levels of p-ERK and Nrf2, suggesting that chrysin exerted its anti-cancer activity by modulating the ERK/Nrf2 pathway(Reference Wang, Wang and Sun119).
Polyphenols, including chrysin, were shown to induce p53 and proline oxidase (PRODH/POX), the catalytic enzyme for the conversion of proline to pyrroline-5-carboxylate, in oral adenosquamous carcinoma (CAL-27 cells). Treated cells showed a significant reduction in collagen biosynthesis and glutathione levels, the loss of which is directly related to apoptosis. Imidodipeptides (containing proline) are the degradation products of collagen, and prolidase is an enzyme which further hydrolyses these peptides to amino acids. Therefore, prolidases contribute to collagen re-synthesis by providing proline. Mitochondrial degradation of proline to pyrroline-5-carboxylic acid by PRODH/POX generates superoxide anions that may contribute to the induction of apoptosis(Reference Celińska-Janowicz, Zaręba and Lazarek120). Finally, chrysin was able to suppress cell invasion of triple negative breast cancer cells (MDA-MB-231 and BT-549) through inhibition of MMP-10, PI3K/Akt and epithelial to mesenchymal transition(Reference Yang, Huang and Xiang121).
Galangin
Galangin is a pharmacologically active flavonoid with potent antioxidant, chemopreventive, anti-metastatic and anti-tumour activities(Reference Zhang, Tang and Huang122 , Reference Russo, Longo and Vanella159 , Reference Heo, Sohn and Au160). Galangin suppressed the migration and motility of B16-F10 cells in vitro through a reduction in the expression level of FAK, a regulator of cancer cell invasion and metastasis. Galangin also reduced the metastatic lung nodules of B16-F10 melanoma cells and immunohistochemical studies showed reduced FAK expression in lung tissues(Reference Zhang, Tang and Huang122).
Proliferation, invasion and metastasis of human laryngeal cells (TU212 and HEP-2) were prevented by galangin through suppression of the PI3K/Akt/NF-κB pathway. Galangin induced apoptosis and regulated autophagy in both cell lines. In the TU212 xenograft mouse model, galangin decreased the tumour volume with reduction of Ki-67 expression and increased number of TUNEL positive cells(Reference Wang and Tang123). It has also been reported that galangin inhibits the cell cycle and induces apoptosis in human breast cancer (MCF-7) and human nasopharyngeal carcinoma (NPC-TW076 and NPC-TW039) by inhibiting the PI3K-Akt signalling pathway(Reference Liu, You and Luo161 , Reference Lee, Lin and Meng162). Galangin suppressed the proliferation of retinoblastoma cells (HXO-Rb44 and Y-79) both in vitro and in vivo reportedly through reduction in Akt activity, induction of apoptosis and the increased expression of the phosphatase and tensin homolog (PTEN) tumour suppressor gene(Reference Zou and Xu125). In a separate study, galangin suppressed epithelial–mesenchymal transition (EMT) and proliferation of 786-0 and Caki-1 renal cell carcinoma. The expression of N-cadherin and vimentin decreased while there was an increased expression of E-cadherin, indicative of EMT suppression(Reference Cao, Wang and Chen124).
MMP-9 is an enzyme involved in tumour development that allows cancer cells to degrade type IV collagen present in the basement membrane, thereby favouring invasion and metastasis. The flavonoids galangin and kaempferol individually suppressed phorbol 12-myristate 13-acetate-induced MMP-9 transactivation in HT-1080 (human fibrosarcoma) cells by inhibiting NF-κB and activator protein 1 (AP-1) pathways(Reference Choi, Lee and Lee126).
In ovarian cancer cells (A2780/CP70 and OVCAR-3), VEGF, the key mediator in angiogenesis, was inhibited by galangin and myricetin in a dose-dependent manner. Additionally, expression of the Akt/p70S6K/hypoxia-inducible factor-1α (HIF-1α) pathway was also inhibited after the above treatments. HIF-1α directly increases the expression of VEGF. The ribosomal protein S6 kinase which is a downstream mediator of the PI3K/Akt pathway further regulates angiogenesis by modulating HIF-1α and VEGF proteins. In chicken chorioallantoic membrane assay, galangin and myricetin significantly reduced the formation of blood vessels induced by OVCAR-3 cells(Reference Huang, Chen and Rojanasakul127). There is also evidence that the combination of galangin and cisplatin induced apoptosis in cisplatin-resistant A549 lung cancer cells (A549/DDP). The synergistic combination acted through multiple targets like STAT3/NF-κB and Bax/Bcl-2 pathways and was effective in reducing tumour growth in vivo. Galangin and cisplatin synergistically suppressed nuclear expression of pSTAT3 and p65 and increased the Bax:Bcl-2 ratio. These results were supported by in vivo studies in the A549/DDP xenograft model where the combined treatment was shown to be more efficient than treatment with either compound alone(Reference Yu, Gong and Li128).
The glyoxalase system protects cells from dicarbonyl stress by converting the toxic methylglyoxal (MG) to d-lactate. Galangin modulated Nrf-2 levels in HeLa cells resulting in decreased glyoxalase-1 levels and thereby leading to decreased MG detoxification. Increased accumulation of MG resulted in oxidative stress-induced cell death(Reference Kumar and Tiku129). Manuka honey contains elevated levels of MG which contribute to its anti-bacterial properties(Reference Hayes, Wright and Gardner163). Recently MG has been studied for its role in cancer, and multiple mechanisms have been reported. MG is an endogenously produced metabolite and a potent glycating agent of cell components resulting in production of advanced glycation endproducts. MG can induce apoptosis through ROS generation, accumulation of advanced glycation endproducts or oxidative DNA damage(Reference Paramita and Wisnubroto164). MG induced apoptosis in human osteoblasts and Jurkat leukaemia T cells through oxidative stress, c‐Jun N‐terminal kinase (JNK) activation, loss of MMP, Cyt c and activation of caspases(Reference Du, Suzuki and Nagase165 , Reference Chan, Wu and Shiao166). MG also inhibited mitochondrial complex I in sarcoma 180 cells resulting in mitochondrial membrane potential loss and release of Cyt c, which ultimately led to apoptosis(Reference Ghosh, Bera and Ray167). Moreover, MG was found to disturb the defence mechanisms of MCF-7 cells to oxidative stress and activated caspase-3. The expression of Ki-67 (cell proliferation marker) was lowered, which was indicative of the anti-proliferative effect of MG(Reference Paramita and Wisnubroto164). These studies stipulate that in addition to its anti-bacterial effects, MG also contributes to the anti-cancer potential of manuka honey.
In a human gastric cancer cell line (SGC-7901), treatment with galangin and quercetin increased the number of apoptotic cells compared with control. Galangin was more potent than quercetin in decreasing mitochondrial membrane potential, leading to apoptosis through a mitochondrial pathway involving caspase-8/Bid/Bax activation(Reference Xu, Wang and Zhao130). Further, in HepG2 hepatocellular carcinoma, galangin treatment activated TGF-βR and receptor-regulated SMAD and suppressed the inhibitor SMAD, resulting in increased TGF-βR/SMAD signalling and induction of autophagy and apoptosis in a dose-dependent manner(Reference Wang, Wu and Lin131). Another study reported that apoptosis induction by galangin in hepatocellular carcinoma cells was due to prolonged endoplasmic reticulum stress via activation of MAPK pathways (p38 MAPK, JNK and Erk subfamilies). These MAPK are positive regulators of endoplasmic reticulum stress-induced apoptosis. Galangin also increased cytosolic free Ca2+ and mitochondrial Ca2+ uptake leading to mitochondria-mediated cell death(Reference Su, Chen and Wu132).
Luteolin
Luteolin (3,4,5,7-tetrahydroxy flavone) is a heat-stable flavonoid with anti-cancer, anti-oxidant and anti-inflammatory properties(Reference Tuorkey168). In prostate cancer, luteolin inhibited cell growth through the regulation of the Wnt/β-catenin pathway. Luteolin inhibited the migration of PC-3 cells in transwell and wound healing assays. Moreover, it decreased spheroid formation and self-renewal (or stemness) of these cells by up-regulating frizzled class receptor 6, a negative regulator of the Wnt pathway, that plays an important role in tumorigenesis(Reference Han, Lang and Zhang133). Another study using U-87 MG and U-251 MG glioblastoma cells demonstrated that luteolin inhibited epidermal growth factor receptor (EGFR) and its downstream Akt and MAPK signalling pathways. Luteolin reduced Bcl‐xL and increased the levels of cleaved caspase-3, indicating apoptosis. DNA repair pro-survival mechanism was inhibited by luteolin treatment, which was indicated by increased c-PARP levels(Reference Anson, Wilcox and Huseman134). In HL-60 leukaemia cells, luteolin triggered apoptosis through Fas/FasL‐mediated extrinsic pathway that was mediated by increasing acetylation of histone H3 and activation of Erk and JNK pathways. Luteolin treatment activated caspase-3 and -8, and enhanced c-Jun activation which was correlated with FasL expression(Reference Wang, Chen and Chow135).
Numerous studies have reported that luteolin’s ability to inhibit STAT3 is responsible for its apoptotic and anti-metastatic activities. Luteolin blocked Hsp90, which is a stabiliser of p-STAT3 and enhanced the degradation of both Tyr705- and Ser727-phosphorylated STAT3 resulting in apoptosis of various cancer cell types(Reference Fu, Chen and Zhao136). In A-549 lung cancer cells, luteolin and kaempferol directly blocked STAT3–DNA interaction by inhibiting STAT3 communication with the promoter region of claudin-2, a membrane protein present at cell tight junctions(Reference Sonoki, Tanimae and Endo137). Another study reported that in PANC-1 and SW1990 pancreatic cells, luteolin inhibited STAT3 and EMT in a dose-dependent manner. Luteolin treatment also inhibited the metalloproteinases MMP-2, MMP-7 and MMP-9 and reversed IL-6-induced EMT in these cells, which was partly attributed to STAT3 inhibition(Reference Huang, Dai and Dai142).
STAT3 inhibition by luteolin has also been reported in gastric cancer cells. The drug-resistant cell lines (SGC7901/DDP, BGC823 and HGC27) showed higher sensitivity to luteolin when compared with the drug-sensitive cell line SGC7901. In SGC7901/DDP cells, luteolin treatment disrupted the interaction of HSP-90 and STAT-3 by increasing the binding of SHP-1 to STAT3, which ultimately promoted STAT-3 dephosphorylation. Inhibition of STAT3 was also observed in xenograft models where tumour growth significantly decreased after luteolin treatment in SGC7901/DDP and HGC27-bearing mice but not in those implanted with SGC7901 cells(Reference Ryu, Lim and Bazer118). Similarly, luteolin inhibited Janus kinase/STAT3 activation and decreased viability of human cholangiocarcinoma (KKU-M156) cells. Luteolin significantly reduced IL-6-mediated migration of these cells(Reference Aneknan, Kukongviriyapan and Prawan139).
In a recent study, luteolin and apigenin were shown to suppress MMP-1 and CYP1A1 activity, which are the triggering factors of intravasation, in MDA-MB231 cells, thus preventing the movement of cell spheroids through the lymph–endothelial barrier. MMP-1 inhibition prevented the activation of FAK, a protein that facilitates cancer cell migration by loosening the cell matrix. The synergistic inhibition of CYP1A1 by apigenin and luteolin leads to decreased expression of 12(S)-HETE, a pro-intravasation metabolite that helps tumour cells to cross the endothelial barrier through the formation of circular chemorepellent-induced defects in the lymph endothelial cells(Reference Hong, Fristiohady and Nguyen140).
The effect of treatment with a combination of luteolin and conventional anti-cancer drugs has been reported. Enhanced apoptosis of MDA-MB-231 cells was observed with co-treatment of luteolin and paclitaxel compared with paclitaxel alone. The blocking of STAT3 resulted in the activation of Fas and caspases-3 and -8. In an in vivo orthotropic breast tumour model in nude mice, administration of luteolin or paclitaxel alone or in combination reduced the tumour volume by 62·3, 81·8 and 96·5 %, respectively(Reference Yang, Huang and Xiang121). A synergistic pro-apoptotic effect was observed when luteolin was used in combination with sorafenib, a small-molecule multi-kinase inhibitor, in hepatocellular carcinoma cells(Reference Feng, Rong and Wang143).
Quercetin
Quercetin is a ubiquitous flavonoid and its anti-cancer properties have been widely reported. A recent study reported that quercetin sensitised human ovarian cancer cells towards X-irradiation and aggravated DNA damage with significant reduction of tumour growth in vivo (Reference Gong, Yang and Zhang144). In OV2008 and A2780 cells, quercetin induced the endoplasmic reticulum stress marker CHOP (CCAAT/enhancer-binding protein homologous protein) through the PERK/ATF4/eIF2α pathway which in turn promotes apoptosis by increasing the Bax:Bcl-2 ratio. Quercetin enhanced the sensitivity of cells to irradiation leading to increased DNA damage and apoptosis. Quercetin increases H2AX phosphorylation and decreases expression of Rad51, indicative of DNA damage. In an OV2008 xenograft mouse model, only the administration of quercetin 1 h before radiation significantly reduced tumour volume, compared with the individual treatments(Reference Gong, Yang and Zhang144).
Quercetin treatment arrested HL-60 leukaemia cells at the G1 phase and reduced tumour growth in xenograft models. In addition to induction of apoptosis, the autophagic progression of cells was also activated. Quercetin was able to stimulate autophagy of the HL-60 cells by increasing BECLIN-1, PI3K, ATG5-ATG12, ATG7 and also converting LC3-I to LC3-II, which is a distinct feature of autophagy(Reference Calgarotto, Maso and Junior145). Moreover, quercetin inhibited the growth of prostate cancer cells by modulating MAPK, Akt and ROS production(Reference Ward, Mir and Kapur169). In pancreatic cancer, quercetin inhibited invasion, metastasis and EMT via a blockade of the STAT3 pathway. This was mediated through a reduction in the levels of E-cadherin and increased levels of N-cadherin, vimentin, Zeb1, Twist, Slug and Snail, and the MMP-2 and MMP-7 enzymes(Reference Yu, Ye and Xiang149). Other studies showed that quercetin interacted with multiple pathways and induced apoptosis and autophagy in primary effusion lymphoma cells. Quercetin dephosphorylated GSK-3 leading to down-regulation of Wnt/β-catenin and PI3K/Akt/mTOR signalling. Quercetin also inhibited the activation of STAT3(Reference Granato, Rizzello and Montani146). Mitochondria-mediated apoptosis of lung cancer cells was induced by quercetin through the down-regulation of the IL-6/STAT3 pathway by modulation of NF-κB activation. Quercetin had a time-dependent effect on apoptosis, with a significant up-regulation of caspase-3 and PARP, and down-regulation of the Bcl-2:Bax ratio(Reference Mukherjee and Khuda-Bukhsh147).
Quercetin enhanced the efficacy of conventional chemotherapeutic drugs in multi-drug-resistant BEL/5-FU (human hepatocellular carcinoma) cells over-expressing ABC transporters. Quercetin dose-dependently decreased mRNA expression of multiple transporters by blocking the FZD7/β-catenin pathway(Reference Chen, Huang and Ma148). In breast cancer cells, quercetin down-regulated the drug-efflux ABC transporters, increased intracellular doxorubicin levels and potentiated its effect. Moreover, quercetin had a synergistic effect with doxorubicin in inducing apoptosis in MCF-7 and MDA-MB-231 cells. It has also been reported that the combination effectively eliminated stem cells in both cell lines, compared with doxorubicin treatment alone(Reference Li, Yuan and Zhao170).
Quercetin has also been reported to inhibit proteasomal system via suppression of the MEK1/Erk1/2 pathway, in HepG2 cells. Quercetin attenuated the β-subunits of proteasome including β5, which is responsible for its chymotrypsin-like activity(Reference Ding, Chen and Wang150). Furthermore, quercetin inhibited EMT induced by TGF-β1 in SW480 (human colorectal adenocarcinoma) cells via increased expression of E-cadherin and decreased expression of vimentin and Twist1, which are well-known markers of EMT and metastasis(Reference Feng, Song and Jiang152).
In breast cancer cells, quercetin inhibited mammosphere formation, decreased number of foci and migration of CD44+/CD24− cancer stem cells (CSC)(Reference Wang, Yang and Li153 , Reference Li, Zhou and Wang171). In MCF-7 cells, quercetin decreased proliferation and induced apoptosis and G1 arrest of the cell cycle by decreasing the levels of oestrogen receptor-α, cyclin D1 and Bcl-2, and enhancing Bax expression(Reference Li, Zhou and Wang171). The inactivation of CSC was through inhibition of the PI3K/Akt/mTOR-signalling pathway(Reference Li, Zhou and Wang171). Using MDA-MB-231 breast CSC, quercetin was shown to lower the expression levels of aldehyde dehydrogenase 1A1, chemokine receptor type 4, mucin 1, and epithelial cell adhesion molecules resulting in suppressed cell proliferation and invasiveness. Quercetin also arrested the G2/M phase of the cell cycle in MDA-MB-231 cells and further induced their apoptosis(Reference Wang, Yang and Li153).
It is quite intriguing that individual flavonoids exhibited anti-cancer activities at much higher concentrations than are normally present in honey. This might suggest that the anti-cancer properties of honey may be due to their synergistic or additive effects.
Concluding remarks
It is evident from the available reports that honey is an immune modulator and possesses anti-proliferative, apoptotic and anti-metastatic effects against various types of cancer. The anti-inflammatory and free radical-scavenging properties of honey also contribute to its chemopreventive effects. The chemical composition of honey is well studied and reported. Phenolic compounds, which are well-known secondary plant metabolites, are enriched in some types of honey. Until now, only two studies have addressed the bioavailability and metabolites of honey(Reference Schramm, Karim and Schrader52 , Reference Seraglio, Valese and Daguer53). Significant findings from these studies strongly encourage carrying out further research work on the bioavailability properties of honey in the future. Flavonoids are an important class of phenolic compounds exhibiting a wide variety of anti-cancer, antioxidant and anti-inflammatory activities. Numerous published reports have attributed the biological/physiological properties of honey to its phenolic components. Many of the studied flavonoids and other phenolic compounds showed synergistic or additive effects with standard anti-cancer drug regimens. It is quite apparent that the major flavonoids of honey, reviewed in the present paper, may act through many common pathways in different cancer cells. The ability of natural compounds to ubiquitously act on multiple pathways could be the reason for their various modes of action and greater safety profiles. However, one of the potentially important factors that has not been addressed in the majority of previous studies is the effect of sugars on the models tested.
Carcinogenesis is a multi-step process with initiation, promotion and progression stages. There is evidence that honey is able to successfully combat the different stages of cancer development. Researchers are exploring the effect of honey on various signalling pathways to uncover the mechanisms by which it acts against cancer. One of the major tasks would be to define the precise upstream molecular targets by which honey affects cancer growth. In preclinical studies, honey has been shown to be safe with no detectable side effects. Honey also has the ability to mitigate the toxicity of standard chemotherapeutic drugs, most probably through its antioxidant properties. Additional preclinical studies using different models of cancer on honey are needed to verify and extend the promising in vitro data before moving on to clinical trials.
Acknowledgements
Work in Professor Basel al-Ramadi’s laboratory was supported by a Zayed Centre grant (no. 31R025) from the Office of Research and Sponsored Projects, UAE University.
S. A. and S. M. H. researched the data and prepared the first draft of the manuscript. M. J. F.-C. and F. G. supervised the writing and edited the manuscript. B. K. a.-R. and M. B. designed and planned the manuscript, and revised and edited the final version for submission. All authors approved the manuscript.
The authors have no relevant interests to declare.