Introduction
Osteoporosis is a ‘progressive systemic skeletal disease characterised by low bone mass and microarchitectural deterioration of bone tissue, with a consequent increase in bone fragility and susceptibility to fracture’( 1 ). The condition has been estimated to affect 75 million individuals in Europe, Japan and the USA( 2 ). Moreover, fragility fractures, the clinical manifestation of osteoporosis, are a major global health issue with an annual prevalence of 8·9 million fractures worldwide( Reference Johnell and Kanis 3 ). The elderly are the most at-risk population( Reference Kanis, Borgstrom and De Laet 4 ) and as the world's population aged 60 and 80 years plus is estimated to increase three- and seven-fold by 2100, respectively( 5 ), osteoporosis and related fractures will become an increasingly bigger health burden.
Risk factors for the development of osteoporosis and fragility fractures include genetic and biological factors, although environmental factors, including diet, are of great interest for developing preventative strategies, as they are modifiable. To date, a wide range of nutrients, foods and food groups has been studied in relation to bone health, including fruits and vegetables, with every increased serving or intakes of one to four portions per d, on at least three d per week, being positively associated with increased bone mass or a reduction in bone loss( Reference New, Bolton-Smith and Grubb 6 – Reference Prynne, Mishra and O'Connell 9 ). The mechanisms underlying these positive associations have not been fully elucidated but one such explanation is the potential buffering effect of the overall dietary acid load by constituents in fruits and vegetables( Reference Lanham-New 10 ). Moreover, epidemiological studies have suggested that these beneficial effects may also be due to micronutrients such as vitamin C, which may have mechanisms independent of these buffering effects( Reference Hall and Greendale 11 , Reference Simon and Hudes 12 ). Vitamin C, an essential nutrient to humans found in citrus and soft fruits( 13 , 14 ), has previously been linked to bone health, particularly bone structure. For example, in previous animal studies vitamin C deprivation resulted in a marked reduction in bone formation( Reference Togari, Arai and Nakagawa 15 – Reference Hasegawa, Li and Hara 17 ); and superoxide-induced bone loss in mice was restored by oral administration of 1 % vitamin C in drinking water, as evidenced by significant improvements in bone mineral density (BMD), bone weight, bone strength and collagen cross-links( Reference Nojiri, Saita and Morikawa 18 ). In the last two decades, observational and intervention studies have investigated a potential role for vitamin C in osteoporosis and fracture prevention; however, an overall consensus of the results of published studies does not exist.
The present article provides a review of the potential underlying mechanisms of vitamin C in bone metabolism. The current evidence in the literature investigating a potential role for vitamin C in the prevention of osteoporosis and related fractures will be discussed and avenues for future research highlighted. Databases, including MEDLINE (Ovid), PubMed and Google Scholar, were used to identify relevant observational and clinical studies published up to August 2013. As neither laboratory nor epidemiological studies can infer causality, criteria established by Sir Austin Bradford Hill in 1965 were used to assess whether vitamin C is causal in the prevention of osteoporosis and associated fractures( Reference Hill 19 ). The structure of the review will be discussed around these criteria.
Bradford Hill criteria
The Bradford Hill criteria (BHC) are a set of guidelines used to assess causality of hypotheses and associations from trial, laboratory and epidemiological research( Reference Hill 19 ). In brief, the nine criteria assess (1) biological plausibility, (2) coherence between laboratory and epidemiological studies, (3) temporality, (4) consistency, (5) strength, (6) analogy, (7) specificity, (8) dose–response effect, and (9) evidence from intervention studies. The criteria may not confirm the absence or presence of causality unconditionally, but are considered to be a useful tool for understanding associations between an exposure and a risk of disease.
Potential mechanisms of vitamin C in bone health
Scurvy, the clinical manifestation of vitamin C deficiency, is associated with wounds and fractures that fail to heal. The discovery of vitamin C in the early 20th century and subsequent animal studies led to the suggestion that scurvy symptoms result from impaired collagen formation in vitamin C deficiency( Reference Robertson 20 ). Collagen is an essential component of bone tissue, and more recently, many cell and animal studies reported that vitamin C may also mediate osteoclastogenesis and osteoblastogenesis( Reference Pradel, Mai and Gedrange 21 – Reference Urban, Hohling and Luttenberg 24 ), although the precise biological mechanisms have not been fully established yet.
Osteoclastogenesis
Vitamin C has been suggested to mediate osteoclast differentiation and possibly apoptosis( Reference Hie and Tsukamoto 22 , Reference Le Nihouannen, Barralet and Fong 25 ) and findings have been relatively consistent. In cell cultures containing both osteoblasts and osteoclasts, vitamin C promoted osteoclastogenesis( Reference Ragab, Lavish and Banks 26 – Reference Takarada, Hinoi and Kambe 28 ) and this was associated with an increase in receptor activator of NF-κB ligand (RANKL) expression( Reference Otsuka, Kato and Hirose 27 ). In concordance with these findings, vitamin C deficiency resulted in a decrease in osteoclast differentiation( Reference Ragab, Lavish and Banks 26 , Reference Otsuka, Kato and Hirose 27 ). However, in cultures containing only osteoclasts, stimulatory effects( Reference Tsuneto, Yamazaki and Yoshino 29 ) as well as inhibitory effects( Reference Hie and Tsukamoto 22 , Reference Takarada, Hinoi and Kambe 28 , Reference Xiao, Liao and Zhou 30 ) of vitamin C on osteoclast differentiation have been reported. Recent in vitro findings have helped explain these contradictory results by showing that vitamin C at a concentration of 50 μg/ml initially exhibited pro-oxidant activity resulting in an increase in the number, size and nucleation of osteoclasts, although vitamin C also initiated accelerated osteoclast death at later stages( Reference Le Nihouannen, Barralet and Fong 25 ). Deficiency studies are in agreement with most previous findings, indicating that vitamin C deficiency in animal models stimulated osteoclastogenesis via the up-regulation of the RANKL/RANK pathway( Reference Hie and Tsukamoto 22 , Reference Park, Lee and Kim 23 ). Moreover, vitamin C-deficient mice supplemented with vitamin C had a reduction in RANKL expression( Reference Park, Lee and Kim 23 ). Although there is some consistency of previous cell and animal studies reporting on the effects of vitamin C on osteoclastogenesis, the current discrepancies require further investigation in human subjects to help decide if vitamin C may be involved in osteoclastogenesis via mediating the RANK/RANKL pathway.
Osteoblastogenesis
Vitamin C may be involved in accentuating osteoblastogenesis. For example, a decrease in the number of osteoblasts and suppressed osteoblast differentiation has previously been observed in vitamin C-deficient mice( Reference Park, Lee and Kim 23 ). In concordance with these findings, an increase in the number of osteoblasts following vitamin C treatment has been reported from in vitro work( Reference Harada, Matsumoto and Ogata 31 ). Furthermore, studies using osteoblast-like cell cultures including human tissue have shown that osteoblast proliferation and differentiation were enhanced with the addition of vitamin C( Reference Pradel, Mai and Gedrange 21 , Reference Urban, Hohling and Luttenberg 24 , Reference Harada, Matsumoto and Ogata 31 – Reference Siggelkow, Rebenstorff and Kurre 33 ). Concentrations of 50 and 200 μg/ml vitamin C have previously been suggested as optimal and maximum concentrations for this effect( Reference Pradel, Mai and Gedrange 21 , Reference Urban, Hohling and Luttenberg 24 ).
Initially, work suggested that the effects of vitamin C on osteoblastogenesis may be through stimulating collagen synthesis( Reference Harada, Matsumoto and Ogata 31 , Reference Otsuka, Yamaguchi and Hirose 32 ), although more recent evidence suggests that the underlying mechanisms are more complex. For example, vitamin C has been reported to mediate gene expression of a number of genes involved in pre-osteoblast cell activities including growth, metabolism, communication and death( Reference Carinci, Pezzetti and Spina 34 ). Furthermore, animal studies have shown that the expression of PPAR-γ may mediate osteoblast differentiation resulting in bone loss( Reference Ali, Weinstein and Stewart 35 , Reference Liu, Li and Yu 36 ). Recently, these findings have been investigated further and a link to vitamin C has been established. An in vivo study reported that PPAR-γ expression in osteoblasts was significantly up-regulated in vitamin C-deficient mice and was accompanied by suppressed osteoblast differentiation, whereas treatment with vitamin C mediated PPAR-γ expression to almost normal levels( Reference Park, Lee and Kim 23 ). To date, there is consistent experimental evidence for a beneficial role of vitamin C in osteoblastogenesis. Recent work suggesting that vitamin C may mediate PPAR-γ expression has provided more insight into the mechanisms, and further experimental studies are needed to confirm these findings.
Bone collagen synthesis
Vitamin C is essential for collagen type I synthesis by osteoblasts. For example, early in vitro work reported that collagen synthesis increased more than four-fold in the presence of ascorbate( Reference Geesin, Darr and Kaufman 37 ). More recently, greater amounts of collagen were shown to be present at vitamin C concentrations of 200 μg/ml compared with 100 and 25 μg/ml( Reference Urban, Hohling and Luttenberg 24 ). The underlying mechanisms for this are thought to relate to the role of vitamin C in stimulating collagen synthesis and as a cofactor of hydroxylation reactions within collagen fibres. For the former, vitamin C is an important initiator of collagen synthesis in osteoblasts( Reference Franceschi and Young 38 ), possibly via stimulating pro-collagen type I mRNA( Reference Chan, Lamande and Cole 39 , Reference Franceschi and Iyer 40 ); whereas for the latter, vitamin C is an essential activator of enzymes involved in the hydroxylation of proline and lysine residues within collagen fibres( Reference Hutton, Tappel and Udenfriend 41 – Reference Myllyla, Majamaa and Gunzler 44 ). The hydroxylation reaction enables the formation of covalent bonds between the amino acid residues, increasing overall collagen strength. Early in vitro and in vivo studies found that the lack of ascorbic acid resulted in the formation of underhydroxylated and unhydroxylated collagen( Reference Stone and Meister 45 – Reference Peterkofsky 49 ), thus decreasing bone matrix stability and weakening bone structure. In contrast, the presence of vitamin C increased the hydroxylation of amino acid residues in vitro ( Reference Franceschi, Iyer and Cui 50 ). The hydroxylation of amino acid residues may occur while the collagen polypeptide chain is still being synthesised and attached to the ribosome( Reference Harwood, Grant and Jackson 51 , Reference Uitto and Prockop 52 ). However, more recent work suggested that this hydroxylation reaction takes place in the endoplasmic reticulum( Reference Nabavi, Pustylnik and Harrison 53 ).
Experimental evidence for a role of vitamin C in bone collagen synthesis is well established. Vitamin C is important for the quality of collagen via its cofactor role in hydroxylation reactions in collagen fibres. Future studies should focus on the importance of vitamin C for the quantity of collagen synthesis via stimulating procollagen type I mRNA, as there are currently only limited data on this potential link.
In summary, a range of mechanisms of vitamin C in maintaining bone health has been suggested in a number of experimental studies. Thus, there is some good evidence for the BHC of biological plausibility for vitamin C deficiency and osteoporosis. The evidence for a role of vitamin C in osteoblastogenesis and in quality aspects of bone collagen synthesis is consistent. In contrast, the links between vitamin C and osteoclastogenesis as well as quantity aspects of collagen synthesis are currently less well defined and require further investigation.
Measures of vitamin C intake and status
Vitamin C intake may be measured from dietary assessment methods such as food diaries and FFQ( Reference Dehghan, Akhtar-Danesh and McMillan 54 ). Food diaries assess habitual intake through a detailed description of foods and drinks consumed typically in the preceding 3 to 7 d and FFQ make use of a food list with a frequency response section estimating intake usually from the previous 12 months. The mean vitamin C intake in the UK is 90 mg/d (calculated using food records)( Reference Bates, Lennox and Bates 55 ), reflecting sufficient intake according to the reference nutrient intake (RNI) of 40 mg/d( 13 ) and in comparison with the US recommendations of 90 and 75 mg/d for men and women, respectively( 56 ). The lower RNI (LRNI) has been set in the UK at 10 mg/d and is based on the prevention and cure of scurvy( 13 ). Currently, there is no upper limit for vitamin C intake. However, very high intakes of 1000 mg/d and above, achieved through the use of supplements, may present with side effects including gastrointestinal discomfort and diarrhoea( Reference Hoffer 57 ) and have previously been shown to increase the risk of renal stones( Reference Taylor, Stampfer and Curhan 58 ).
The ability to accurately assess vitamin C intake varies between the different dietary methods, with the correlation coefficients between blood vitamin C concentrations and dietary intake being higher for food diaries, dietary recalls (both r 0·46; 95 % CI 0·41, 0·52) and weighed records (r 0·39; 95 % CI 0·25, 0·53) compared with the correlation coefficient between blood vitamin C concentrations and dietary intake estimated from FFQ (r 0·35; 95 % CI 0·29, 0·40)( Reference Dehghan, Akhtar-Danesh and McMillan 54 ). Despite the ability to estimate vitamin C intake, the measurement of vitamin C status from blood may be more accurate than dietary intake assessments as it avoids human recall error and variations in individual bioavailability of the nutrient and accounts for factors that affect the vitamin C composition of food including length of storage of food items and cooking practices( Reference Levine, Rumsey and Daruwala 59 ). However, vitamin C in blood is influenced by a number of biological and lifestyle factors including age( Reference Drewnowski, Rock and Henderson 60 ), sex( Reference Galan, Viteri and Bertrais 61 , Reference Faure, Preziosi and Roussel 62 ), BMI( Reference Drewnowski, Rock and Henderson 60 ), body fat distribution( Reference Canoy, Wareham and Welch 63 ), smoking( Reference Chow, Thacker and Changchit 64 , Reference Schectman, Byrd and Gruchow 65 ) and infection( Reference Scrimshaw and SanGiovanni 66 ) which should be accounted for when evaluating its association with disease risk.
Dietary intake and plasma concentrations of vitamin C, when plotted against each other, show a sigmoidal relationship( Reference Levine, Conry-Cantilena and Wang 67 , Reference Levine, Wang and Padayatty 68 ). Average vitamin C intakes (60–100 mg/d) reflect plasma levels of about 40–60 μmol/l. Higher intakes result in a progressive flattening of the curve and very high intakes of 400 mg/d and above appear to saturate vitamin C in plasma at concentrations of 70–85 μmol/l, leading to the excretion of the vitamin( Reference Levine, Wang and Padayatty 68 ). The mean plasma vitamin C concentration of the general UK population is 53 μmol/l( Reference Bates, Lennox and Prentice 69 ). Vitamin C status may be categorised as severely deficient at plasma levels below 11 μmol/l indicating biochemical depletion; and 1 % of men and 2 % of women in the UK are classified as such( Reference Bates, Lennox and Prentice 69 ).
Current evidence on vitamin C, osteoporosis and fracture prevention
There is evidence from epidemiological studies for a potential role of vitamin C in maintaining different aspects of bone health, although the results have varied between studies. In the next section, randomised controlled trials (RCT) as the best indicator of causality will be discussed first and this will be followed by observational studies in hierarchical order of decreasing ability to determine causality. All types of studies will be evaluated against the BHC.
Intervention studies
RCT are the only studies that can definitively infer causality and determine factors influencing disease, making them the ‘gold standard’ in limiting selection bias and confounding. To our knowledge, there is only one such published RCT with a double-blind design that has examined the effects of vitamin C supplementation on indicators of bone health (Table 1). The study involving thirty men and women compared bone density of one group taking a placebo with that of two groups receiving 400 IU of vitamin E daily and either 500 or 1000 mg/d of vitamin C for 12 months( Reference Ruiz-Ramos, Vargas and Van der Goes 70 ). The group with the highest vitamin C intake had significantly less hip bone loss compared with the placebo group (effect sizes and P values not shown), although no such observations were made at the lumbar spine. However, this study did not investigate the effects of vitamin C independently and the inclusion criteria allowed for smokers and for participants with controlled chronic disease, which may have biased the study outcomes. Thus, it remains unclear to what extent vitamin C was involved in preventing bone loss in this study.
Table 1 Summary of intervention studies investigating the effects of vitamin C on bone mineral density (BMD) and markers of bone turnover

BSALP, bone-specific alkaline phosphatase; CTX, collagen type 1 cross-linked C-telopeptide; FN, femoral neck; LS, lumbar spine; RCT, randomised placebo-controlled trial; TH, total hip.
* Results were of mixed nature (M).
Two intervention studies used a combination of an exercise programme and supplementation with vitamins C and E( Reference Maimoun, Simar and Caillaud 71 , Reference Chuin, Labonte and Tessier 72 ). The first study was a randomised placebo-controlled pilot study in thirty-four women who followed an intervention of 60 min of resistant training three times per week and daily supplementation with vitamins C (1000 mg/d) and E (600 mg/d) for 6 months. Women were randomised into four treatment groups of placebo, vitamins, exercise and placebo, or exercise and vitamins( Reference Chuin, Labonte and Tessier 72 ). BMD of the lumbar spine but not the femoral neck decreased significantly by 1 % in the placebo group over 6 months (BMD pre: 1·01 (sd 0·17) g/cm2; BMD post: 1·00 (sd 0·16) g/cm2; P< 0·05) and was maintained in the other groups. No additive effects of the exercise intervention and the vitamin supplementation were found. However, the results may have been biased by changes in dietary habits as a reduction in vitamin C intake over the course of the study period was reported for the vitamin intervention group. Moreover, the study did not report on blinding in the protocol. The second study, a 2-month intervention in thirteen men and women, included 1 h of aerobic exercise three times per week and the daily use of vitamin C (500 mg/d) and vitamin E (100 mg/d) supplements for all subjects( Reference Maimoun, Simar and Caillaud 71 ). Although markers of Ca homeostasis improved significantly (effect sizes not reported), the bone formation marker bone-specific alkaline phosphatase decreased unexpectedly by 14·5 % (P value not reported). However, this study lacked a control group, was undertaken in only thirteen individuals, and since it was a mixed intervention, the effects of vitamin C could not be distinguished. Moreover, both studies were of short duration of only 2–6 months, although changes in BMD are more likely to be observed after a longer duration of treatment.
In summary, evidence from current trials investigating potential preventative effects of vitamin C in osteoporosis remains equivocal, even though the doses were greater than with diet alone. There are limitations regarding study design, inclusion and exclusion criteria, limited duration of treatment, small sample sizes and dietary intake that were not controlled for. Moreover, published intervention studies have used vitamin supplements containing vitamin E in addition to vitamin C and have included exercise programmes during treatment. Future trials should consider having more participants, stricter inclusion and exclusion criteria and interventions consisting of vitamin C supplementation only. The BHC of evidence from intervention studies is therefore not met.
Prospective and longitudinal studies
Prospective cohort studies may be used to investigate the aetiology of a disease as the exposure is measured before the condition occurring, making studies less prone to recall bias than case–control studies. They may thus also be used to evaluate the BHC of temporality. Furthermore, as cases and controls are drawn from the same population, there is less selection bias. To date, only one prospective and two longitudinal studies have investigated potential vitamin C and bone associations (Table 2). One study of 944 men and women from the UK with a mean age of 72 years reported significantly less total hip BMD loss of up to 54 % for higher dietary intakes of vitamin C (99–363 mg/d) compared with lower intakes (7–57 mg/d)( Reference Kaptoge, Welch and McTaggart 73 ). Another study using a US cohort of 606 subjects with a mean age of 75 years reported that lumbar spine and trochanter BMD loss, but not femoral neck and radial shaft BMD loss, decreased significantly across tertiles of dietary vitamin C intake in men but not in women( Reference Sahni, Hannan and Gagnon 74 ). However, as highlighted above, the findings were not consistent across these two studies, with results varying mainly for sex and bone site. Potential explanations for this might be that the first study used 7 d food diaries and did not adjust for important confounders including age, sex and smoking( Reference Kaptoge, Welch and McTaggart 73 ), in contrast to the second study which used a semi-quantitative FFQ and measured BMD via two different types of bone scans (i.e. dual-photon absorptiometry (DPA) at baseline and dual X-ray absorptiometry (DXA) at follow-up)( Reference Sahni, Hannan and Gagnon 74 ). However, DXA scans have been shown to produce lower results than DPA scans( Reference Holbrook, Barrett-Connor and Klauber 75 ); hence the effect size in this study may be more modest than the true result.
Table 2 Prospective and longitudinal studies assessing associations between vitamin C intake or status and bone mineral density (BMD) or fracture risk

TH, total hip; LS, lumbar spine; FN, femoral neck; T, trochanter; RS, radial shaft.
* Total intake is the sum of dietary intake and intake from supplements.
† Results were of mixed nature (M) or non-significant (NS).
‡ Data shown for men/women.
§ Data shown for hip/non-vertebral fracture analyses.
A potential role for vitamin C in fracture prevention has only been investigated in one previous prospective study of 918 US men and women with a mean age of 75 years. There was a risk reduction in hip fracture of 44 % for supplemental vitamin C intake (mean: 260 mg/d compared with 0 mg/d) and of 69 % for total (dietary and supplemental) vitamin C intake (mean: 313 mg/d compared with 94 mg/d) after 15–17 years of follow-up (relative risk and 95 % CI not reported), although no significant risk reductions were found at other fracture sites( Reference Sahni, Hannan and Gagnon 76 ). As this study was comparatively small, further large prospective cohort studies of older men and women with long follow-up, which investigate fractures as the clinical endpoint of osteoporosis, are needed.
In summary, there are only limited data from three prospective and longitudinal studies investigating potential associations between vitamin C and bone health. Although these prospective studies meet the BHC of temporality, it is difficult to assess the strength of the associations and the potential for a dose–response relationship as not all studies reported effect sizes. Moreover, issues regarding analogy, inferring the absence of another confounder related to the predictor variable, and consistency were present. A greater number of prospective and longitudinal studies and more concordant adjustment for confounding factors may help establish more consistent findings of the relationship between vitamin C intake and osteoporosis and associated fractures. Moreover, the lack of evidence for a relationship between vitamin C status and bone health needs to be investigated further as the only study investigating this did not adjust for age, sex and smoking( Reference Kaptoge, Welch and McTaggart 73 ).
Case–control studies
Case–control studies, summarised in Table 3, are used to examine specific exposures as potential risk factors of a disease in individuals with and without the condition. Recall bias, where case subjects tend to have a better recollection of specific exposures than the controls, and selection bias, resulting from both outcomes being pre-defined, are common issues of these studies. To date, three case–control studies have consistently shown that osteoporosis and fracture patients had lower serum vitamin C concentrations (cases: 17–37 μmol/l; controls: 23–54 μmol/l) and lower plasma vitamin C concentrations (cases: 30 μmol/l; controls: 55 μmol/l) than controls( Reference Falch, Mowe and Bohmer 77 – Reference Martinez-Ramirez, Palma Perez and Delgado-Martinez 79 ). Only one study reported differently, but the authors inferred that their findings reflected most recent changes in food intake( Reference Lumbers, New and Gibson 80 ).
Table 3 Case–control studies assessing vitamin C intake or status in osteoporosis and fracture patients in comparison with controls
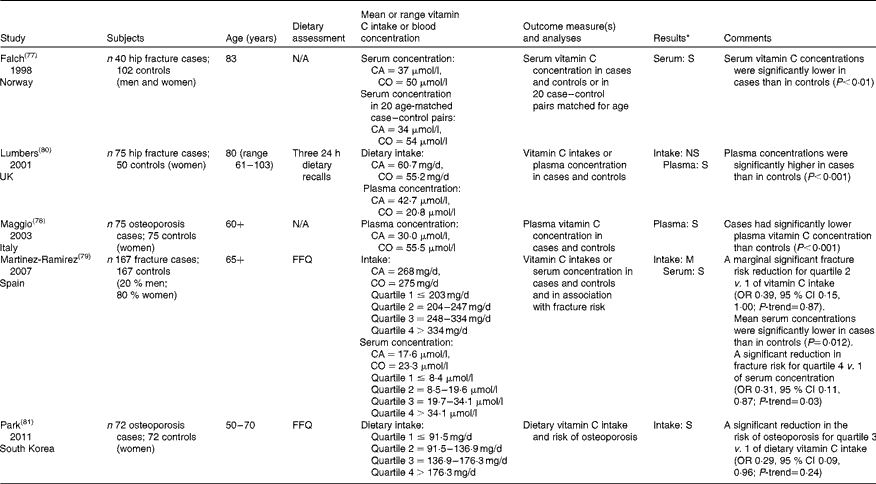
N/A, not applicable; CA, cases; CO, controls.
* Results were significant (S), non-significant (NS) or of mixed nature (M).
In contrast to vitamin C status measures, findings for potential differences in dietary vitamin C intakes between cases and controls are less consistent( Reference Martinez-Ramirez, Palma Perez and Delgado-Martinez 79 , Reference Lumbers, New and Gibson 80 ). Differences in measures of dietary intake and relatively small sample sizes may explain some of these inconsistent findings. However, associations with osteoporosis and fracture risk were reported when population intakes were stratified into quartiles of dietary vitamin C intake. For example, one case–control study showed a marginally significant fracture risk reduction for participants in the second quartile of vitamin C intake compared with the first (OR 0·39, 95 % CI 0·15, 1·00; vitamin C intake range: 204–247 mg/d compared with ≤ 203 mg/d)( Reference Martinez-Ramirez, Palma Perez and Delgado-Martinez 79 ). This was not significant for higher vitamin C intakes, possibly due to the high vitamin C intake of the study population (mean: 200 mg/d). Moreover, another case–control study reported that those in the third quartile of vitamin C intake had a significantly reduced risk of osteoporosis referent to the lowest quartile (OR 0·29, 95 % CI 0·09, 0·96; vitamin C intake range: 137–176 mg/d compared with ≤ 92 mg/d)( Reference Park, Heo and Park 81 ). Recall bias in this study was low due to the diagnosis of osteoporosis at screening and the subsequent reporting of current vitamin C intake.
In conclusion, published case–control studies of osteoporosis and fracture patients have reported consistently lower blood vitamin C concentrations but not dietary intake of vitamin C. Thus, the BHC of consistency is currently not fulfilled. Although reported effect sizes appear to be large, this evidence is currently limited to only two studies. More case–control studies are needed to help clarify the discrepancies in vitamin C intake between osteoporosis and fracture patients and matched controls currently reported in the literature.
Cross-sectional studies
Cross-sectional studies are used to report the prevalence of a disease in a defined population at a specific point in time. Whether the exposure predated the disease or not cannot be determined. Previous cross-sectional studies are summarised in Table 4. Positive associations indicated that higher dietary vitamin C intake was associated with 3–5 % higher BMD( Reference New, Bolton-Smith and Grubb 6 ) and every 100 mg/d increment in vitamin C intake was associated with 0·01–0·02 g/cm2 higher BMD( Reference Hall and Greendale 11 , Reference Simon and Hudes 12 ), although there is currently limited understanding of this clinical relevance. Moreover, users of vitamin C supplements (mean = 745 mg/d; range = 70–5000 mg/d) had 4 % higher BMD and users of supplement doses of ≥ 1000 mg/d had 14 % higher BMD than non-users( Reference Morton, Barrett-Connor and Schneider 82 ). Although positive associations between dietary vitamin C intake and supplements and bone density have previously been reported, findings have been inconsistent( Reference New, Robins and Campbell 8 , Reference Prynne, Mishra and O'Connell 9 , Reference Sahni, Hannan and Gagnon 74 , Reference Sowers, Wallace and Lemke 83 – Reference Pasco, Henry and Wilkinson 85 ). The use of different dietary assessment methods as means of measuring vitamin C intake and differences in the adjustment for confounding factors may explain some of these discrepancies. Dietary methods have included semi-quantitative FFQ with 97–126 food items( Reference New, Bolton-Smith and Grubb 6 , Reference New, Robins and Campbell 8 , Reference Hall and Greendale 11 , Reference Sahni, Hannan and Gagnon 74 , Reference Wolf, Cauley and Pettinger 84 , Reference Leveille, LaCroix and Koepsell 86 , Reference Sugiura, Nakamura and Ogawa 87 ), 3 to 7 d food diaries( Reference Prynne, Mishra and O'Connell 9 , Reference Ilich, Brownbill and Tamborini 88 ) and 24 h recalls( Reference Simon and Hudes 12 , Reference Sowers, Wallace and Lemke 83 ). Moreover, total (dietary and supplemental) vitamin C intake has not been linked with BMD in women( Reference Sowers, Wallace and Lemke 83 , Reference Wolf, Cauley and Pettinger 84 , Reference Ilich, Brownbill and Tamborini 88 ); and both positive and negative associations have been reported in men( Reference Sahni, Hannan and Gagnon 74 ), although the latter findings may have been biased by the population's smoking behaviour. Dietary intakes of vitamin C have previously been shown to be significantly lower in smokers than non-smokers( Reference Schectman, Byrd and Gruchow 65 ) and serum vitamin C levels are lower in smokers independent of dietary intakes( Reference Chow, Thacker and Changchit 64 , Reference Schectman, Byrd and Gruchow 65 ). Hence, the exclusion of smokers to the study may have led to more consistent findings.
Table 4 Cross-sectional studies assessing associations between vitamin C intake or status and bone mineral density (BMD), markers of bone turnover or fracture risk

MR, mid-radius; FN, femoral neck; LS, lumbar spine; T, trochanter; WT, Ward's triangle; TH, total hip; PYD, pyridinoline; DPD, deoxypyridinoline; N/A, not applicable; UR, ultradistal radius; WB, whole body; BMC, bone mineral content; RS, radial shaft; HRT, hormone replacement therapy; CTX, collagen type 1 cross-linked C-telopeptide; BSALP, bone-specific alkaline phosphatase.
* Total intake is the sum of dietary intake and intake from supplements.
† Results were non-significant (NS), of mixed nature (M) or significant (S).
‡ Data shown for men/women.
§ Geometric mean (95 % CI).
∥ Intake range.
Potential associations between vitamin C from the diet or in serum and fracture risk have currently been examined in only one cross-sectional study of more than 13 000 men and women aged 20–90 years( Reference Simon and Hudes 12 ). Findings were non-significant, although men with mean dietary vitamin C intakes of 200 mg/d reported fewer fractures than men with higher or lower intakes. One may be critical about the large age range of the study population. As osteoporosis and associated fractures are known to be more prevalent in the elderly population( Reference Kanis, Borgstrom and De Laet 4 ), the inclusion of very young participants may be an explanation for the non-significant findings.
Cross-sectional data on vitamin C and markers of bone homeostasis are sparse, with only two studies investigating potential associations. One study found that higher intakes of vitamin C were associated with lower excretion of deoxypyridinoline (no effect size shown), indicating reduced bone resorption( Reference New, Robins and Campbell 8 ). Similarly, the other study reported a significant association between the duration of vitamin C supplement use and markers of bone resorption, with serum collagen type 1 cross-linked C-telopeptide concentrations being 0·022 pg/ml lower for every 1-year supplement use increment( Reference Pasco, Henry and Wilkinson 85 ).
Although there are data from a number of cross-sectional studies investigating vitamin C and bone health associations, the BHC of consistency, analogy and temporality were not fulfilled. The effect sizes of published cross-sectional studies are comparable with those previously reported for other dietary factors including K, although many studies did not report effect sizes. The limited number of BHC currently fulfilled by cross-sectional studies may indicate that the reported associations between vitamin C intake and osteoporosis and fractures are less reliable evidence than relationships reported by prospective cohort studies and RCT.
In summary, support for studies, which have investigated the potential underlying mechanisms between vitamin C and osteoporosis prevention, has come from a variety of epidemiological studies, although differences in study populations, dietary exposure, outcome measures and use of confounding factors in statistical analyses may have resulted in inconsistent findings. Current observational data are particularly limited for men as most studies have consisted of only women and for biological markers of vitamin C status which may be less subjective to recall bias and factors influencing the vitamin C content of food( Reference Bates, Thurnham, Bingham, Margetts and Nelson 89 ). More observational studies in the general population are needed to address these limitations.
Moderate v. high vitamin C intakes
Results from three observational studies have indicated that significant associations with bone health were surprisingly stronger for moderate rather than higher vitamin C intakes( Reference New, Bolton-Smith and Grubb 6 , Reference Martinez-Ramirez, Palma Perez and Delgado-Martinez 79 , Reference Park, Heo and Park 81 ). For example, vitamin C intake was significantly associated with higher bone density or a reduction in fracture risk for the second quartile( Reference Martinez-Ramirez, Palma Perez and Delgado-Martinez 79 ) or for the third quartile( Reference New, Bolton-Smith and Grubb 6 , Reference Park, Heo and Park 81 ) of vitamin C intake rather than the highest intake levels. Similar cross-sectional observations have been reported for associations with serum vitamin C levels( Reference Simon and Hudes 12 ). This may suggest that vitamin C may be related to bone density in a bell-shaped dose–response fashion with intakes below and above the optimum not being beneficial. The potential underlying mechanisms for this may relate to the properties of vitamin C rather than bone tissue itself. It has previously been suggested that vitamin C may not only have antioxidant properties, but may also exhibit pro-oxidant traits at higher concentrations, as supplementation of men and women with 500 mg vitamin C per d was shown to promote oxidative DNA damage( Reference Podmore, Griffiths and Herbert 90 ) which may also be relevant to osteoporosis. Moreover, there is evidence from in vitro studies of a vitamin C dose-dependent suppression of bone cell growth and differentiation as well as collagen type I synthesis( Reference Pradel, Mai and Gedrange 21 , Reference Urban, Hohling and Luttenberg 24 , Reference Takamizawa, Maehata and Imai 91 ). For example, vitamin C concentrations of 50 μg/ml were optimal for stimulation of human osteoblast-like cell lines and collagen type I synthesis, whereas higher levels resulted in the inhibition of cell differentiation( Reference Pradel, Mai and Gedrange 21 ). Another experimental study investigating bovine osteoblast-like cell proliferation observed similar effects, although vitamin C concentrations of 200 μg/ml were found to be most effective( Reference Urban, Hohling and Luttenberg 24 ). As suggested by the authors, the use of different cell types may be an explanation for the inconsistencies in optimal vitamin C concentrations in these cell-culture studies. The potential bell-shaped dose–response relationship between vitamin C and indicators of bone health may also further explain the lack of positive results reported in the intervention studies discussed above which included high supplement doses of 500–1000 mg/d. The potentially detrimental effects of higher vitamin C concentrations on the skeleton need to be investigated further; and this is a crucial step towards establishing optimal vitamin C intake levels.
Discussion and conclusions
Evaluating the current evidence for a potential role of vitamin C in osteoporosis and fracture prevention according to the BHC in the absence of RCT provides some clarity regarding causality( Reference Hill 19 ). The BHC of specificity, inferring that a cause leads to a single effect, cannot be met, as biological functions of vitamin C are versatile. However, there is emerging experimental evidence for a potential role of vitamin C in bone health, thus fulfilling the BHC of biological plausibility. The mechanisms include the involvement of vitamin C in osteoclastogenesis via RANKL expression, osteoblastogenesis via PPAR-γ expression( Reference Hie and Tsukamoto 22 , Reference Park, Lee and Kim 23 ) and collagen synthesis via stimulation of pro-collagen mRNA expression and the hydroxylation of collagen fibres( Reference Franceschi and Young 38 – Reference Franceschi and Iyer 40 ). A number of observational studies support these findings; thus the BHC of coherence between laboratory and epidemiological studies is met. However, differences in study populations, different methods of measuring dietary exposure, outcome measures and use of confounding factors in these observational studies may have resulted in inconsistent findings. Consequently, the BHC of consistency and analogy are currently not fulfilled. Addressing these limitations in future epidemiological studies may help establish more consistent results.
Most observational studies published to date were of a cross-sectional nature. Thus, the BHC of temporality, inferring that the exposure preceded the disease outcome, was not met, and more cohort studies in the general population are needed to overcome this problem. Moreover, evaluating the BHC of the strength of the association based on the evidence currently available in the literature leads to equivocal conclusions, as a large number of studies did not report effect sizes of their findings. Future studies should report effect sizes to help understand the overall clinical relevance of vitamin C for the prevention of osteoporosis and fractures.
The present review has highlighted that potential associations between vitamin C and bone health may not follow an expected dose–response curve due to the vitamin exhibiting antioxidant properties at lower and pro-oxidant traits at higher concentrations. Potentially detrimental effects on the skeleton from higher vitamin C concentrations need to be investigated further, as this may be an issue with vitamin C supplementation, and understanding this is a crucial step towards establishing optimal vitamin C intake levels for the general population.
The final BHC of evidence from intervention studies is currently not fulfilled, although the conventional hierarchy of the validity of study designs may be less applicable to nutritional research, as cross-sectional studies tend to capture long-term dietary intake more so than intervention studies. Nevertheless, published intervention studies were not designed to evaluate the independent effects of vitamin C supplementation on potential improvements in bone health as interventions included additional supplementation with vitamin E and exercise programmes. Overall, the data are limited as only one double-blind RCT and two intervention studies have investigated this and dietary intake was not controlled for. Moreover, further issues regarding study design, inclusion and exclusion criteria, duration of treatment and sample size were present. To our knowledge, published RCT investigating the potential link between vitamin C and bone that use a supplement containing vitamin C only are still lacking and are urgently needed.
In conclusion, over the last few decades, in vitro and in vivo studies have provided insights and knowledge as to how vitamin C may influence the mechanisms that benefit the skeleton, and observational studies have provided some evidence for a potential role of vitamin C in osteoporosis and fracture prevention. However, data are limited, as good-quality studies are scarce and more investigations, particularly well-designed RCT, are urgently needed to address the limitations outlined in the present review.
Acknowledgements
This research was supported by a PhD studentship funded by the postgraduate funding office of the Faculty of Medicine and Health Sciences at the University of East Anglia, and received no specific grant from any funding agency in the commercial sector. The Faculty of Medicine postgraduate funding office had no role in the design, analysis or writing of this article.
A. A. W. was the principal investigator and initiated the study. H. F. conducted the literature review and drafted the manuscript. A. A. W. and A. R. H. directed the preparation of the manuscript and A. A. W., A. R. H and A. J. contributed significantly to the drafting and critical reviewing of the article. All authors read and approved the final manuscript.
There are no conflicts of interest.