Introduction
A number of diverse viruses are known to infect the respiratory tract (Fig. 1). In broad terms severe disease is restricted to infections of the lower respiratory tract (LRT), resulting in bronchiolitis and pneumonia. Such infections are the leading cause of hospitalization and deaths in infants and young children particularly in the developed world, [Reference Reed1], and of severe illness and death in subjects aged >65 years in industrialized countries. For example, respiratory syncytial virus (RSV) infections resulted in the hospitalization of 3·4 million young children, with about 133 000 deaths in 2005 worldwide [Reference Nair2]. Influenza virus infected 90 million and caused about 70 000 deaths in the same age group in 2008 [Reference Nair3]. In the elderly population of the USA, about 100 000 deaths due to RSV and 36 000 deaths due to influenza occur each year, according to the World Health Organization (WHO). Viral infections of the respiratory tract not only pose a significant global health burden, but also a significant financial burden due to healthcare costs and workdays lost. The WHO has estimated that 23 million workdays are lost per annum in the USA due to the common cold.

Fig. 1 [colour online]. Common viral infections of the respiratory tract.
Outbreaks of respiratory viral infections, such as those caused by influenza A [Reference Juzeniene4] and RSV [Reference Shek and Lee5], have long been associated with the changing seasons, reaching a peak in the winter months and a nadir in the summer months. Various factors have been proposed to explain this pattern, including increased survival and transmission of the viruses at low temperature and humidity, cold dry air reducing mucus in the nasal passages, and the tendency for indoor congregation during winter months [Reference Juzeniene4], although human gathering occurs throughout the year, with public transport, schools and places of work likely to provide ample opportunity for viral transmission.
More than 30 years ago Hope-Simpson wrote:
latitude alone broadly determines the timing of epidemics [of respiratory infections] in the annual cycle, a relationship that suggests a rather direct effect of solar radiation acting positively or negatively upon the virus, the human host or their interaction [Reference Hope-Simpson6].
Therefore solar ultraviolet (UV) radiation could influence the seasonality of respiratory infections at the majority of latitudes, providing most protection in the summer months when the UVB (280–315 nm) in sunlight is at a maximum. In the tropics where there is little fluctuation in solar UVB radiation, the seasonality of respiratory viral infections is blunted [Reference Juzeniene4, Reference Shek and Lee5], with influenza being more prevalent in the rainy season when sunlight is impaired by clouds and rain [Reference Shek and Lee5]. As vitamin D is produced following exposure of the skin to solar UVB, individuals living at mid to high latitudes frequently have lower vitamin D status in winter compared to summer, because of the lack of UVB radiation during the winter months. The seasonal fluctuation in vitamin D level thus has an inverse correlation with the seasonality of many respiratory viral infections. Solar flare activity has been noted as influencing respiratory disease rates, with a higher number of reported cases following solar flares [Reference Hayes7]. Such flares increase atmospheric ozone, and hence reduce the UVB radiation reaching the earth's surface and, in turn, the generation of vitamin D in human skin is lower. Therefore a protective role for vitamin D in determining susceptibility to respiratory viral infections has been proposed, providing an intriguing explanation for the seasonality of respiratory viral infections, as well as offering a potential prophylactic for reducing the global burden of these diseases.
In this review, the immune mechanisms by which vitamin D could influence the host response are outlined, and the evidence from observational studies and clinical trials discussed in relation to vitamin D and respiratory viral infections. The potential of vitamin D to improve the efficacy of vaccination against some respiratory viruses is also examined.
Vitamin D
The majority of vitamin D in most individuals is produced following exposure of the skin to the UVB component of sunlight (280–315 nm). The 7-dehydrocholesterol in the cutaneous keratinocyte membranes is converted in a series of steps to 25-hydroxyvitamin D3 [25(OH)D3, calcidiol] and then to the active form of vitamin D, 1,25-dihydroxyvitamin D3 [1,25(OH)2D3, calcitriol] [Reference Holick8] (Fig. 2). The actions of 1,25(OH)2D3 are mediated through ligation with a nuclear vitamin D receptor (VDR), resulting in the regulation of the transcription of at least 1000 genes. 1,25(OH)2D3 also induces rapid membrane signalling through a specific membrane receptor, recently identified as a protein disulfide isomerase. The amount of circulating 1,25(OH)2D3 is tightly regulated by negative feedback control through induction by the hormone of 24-hydroxylase which catabolizes both 25(OH)D3 and 1,25(OH)2D3 to various calcitronic acid products.

Fig. 2 [colour online]. Synthesis of 25-hydroxyvitamin D [25(OH)D] and 1,25-dihyroxyvitamin D [1,25(OH)2D] following solar UVB radiation or ingestion of vitamin D.
Dietary vitamin D, in the form of either D2 or D3, normally makes only a minor contribution to the total vitamin D requirement, and gets absorbed from the gastrointestinal tract into the circulation. Vitamin D is found in oily fish, egg yolk and irradiated food such as mushrooms, with the highest levels found in cod liver oil. A limited number of common staples are also fortified with vitamin D. Vitamin supplements in the form of D2 or D3, provide an alternative source. It has been reported that ingesting 10 000 IU vitamin D3 is equivalent to whole-body UV radiation exposure, at a dose sufficient to cause just perceptible erythema. Such an estimate is unlikely to be accurate under natural outdoor conditions, and efforts have been made recently to construct models which provide a better understanding of how much sun exposure an individual requires under different climatic conditions to result in a particular vitamin D status [Reference Kazantzidis9, Reference Diffey10].
UVB makes up about 6% of ambient sunlight at most. The percentage depends on many external factors including latitude, season, time of day, altitude, ozone, cloud cover, air pollution and reflective surfaces. At latitudes above 45° (London is 51° N), there is essentially no solar UVB reaching the surface of the earth during the winter months, due to the decreased solar zenith angle. This is likely to result in a reduction in vitamin D status, which begins to recover in the spring. For example, in the USA, the peak in vitamin D levels occurs in August and the trough in February, a lag of 8 weeks after the peak and trough, respectively, in ambient UVB [Reference Kasahara, Singh and Noymer11]. Personal factors are also important in determining vitamin D production from solar UVB irradiation, such as skin type, age, amount of clothing worn and head cover, use of sunscreen, body mass index, serum cholesterol concentration, polymorphisms in the VDR and enzymes of the vitamin D metabolic pathway, and baseline 25(OH)D level. For example, to produce the same amount of pre-vitamin D3, about a sixfold higher dose of UVB radiation is required for black skin compared to fair skin, due to absorption by melanin, and a twofold higher dose is required for an 80-year-old compared to a 20-year-old, due to a reduction in 7-dehydrocholesterol in older skin.
The vitamin D status of an individual is usually inferred by the concentration of 25(OH)D in the serum or plasma, but a number of caveats require consideration. In many studies only one sample per individual is assessed, frequently without taking into account the season, past history of sun exposure, place of residence and other environmental and personal factors. The 25(OH)D assay is known to lack accuracy, reproducibility and sensitivity [Reference Fraser and Milan12], although this should improve with the recent implementation of a vitamin D standardization programme using liquid chromography-tandem mass spectroscopy (LC-MS/MS). There is also no consensus regarding what concentration of 25(OH)D constitutes ‘satisfactory’. Although a level of at least 10 ng/ml 25(OH)D is thought necessary to promote bone mineralization and calcium homeostasis, this endpoint is not consistent between studies [Reference Cranney13]. A range of 20 ng/ml (50 nmol/l) to 50 ng/ml may best provide the immunomodulatory effects of vitamin D [Reference Ross14], while concentrations >100 ng/ml may be harmful, although toxicity is rare below 200 ng/ml [Reference Heaney15].
Immune responses to respiratory viral infection
Following entry into the respiratory tract and infection of predominantly epithelial cells, viruses trigger the innate immune responses including the inflammatory response. Neutrophils enter the lung parenchyma within hours of infection, followed by monocytes/macrophages, natural killer (NK) cells and then T cells within a few days of infection. The T cell infiltration peaks at 7 days post-infection, correlating with viral clearance from the lung. Neutralizing antibodies are also present around 7 days post-infection, and are maintained in the host as a first line of defence against re-infection.
The innate immune cells elicit an early anti-viral response following recognition of the pathogen by pattern recognition receptors including Toll-like receptors (TLRs) and RIG-like receptors [Reference Kreijtz, Fouchier and Rimmelzwaan16]. The engagement of these receptors induces signalling cascades that generate type I interferon (IFN) and pro-inflammatory cytokines. These molecules control the infection by mechanisms of viral cleavage and inhibition of viral fusion, replication and translation, by activating cytolytic cells and stimulating humoral factors including acute phase proteins, defensins, collectins and complement proteins. However, as these responses can lead to immunopathology in the lung [Reference Nakano17] mediating the morbidity and mortality of respiratory viral infections, they require immune regulation.
The adaptive immune response is also responsible for the recovery from viral respiratory infection. Antigen-presenting cells, including macrophages and dendritic cells, process and present the viral antigens to CD4+ and CD8+ T cells in the draining lymph node. The T cells then migrate and accumulate in the infected tissue to mediate cytolytic and pro-inflammatory effects. CD4+ T helper cells are central: they promote the B cell response including proliferation, differentiation to plasma cells, immunoglobulin class switching to produce IgG and IgA antibodies, affinity maturation and memory B cell induction, and they also promote cytolytic CD8+ T cell responses [Reference Kreijtz, Fouchier and Rimmelzwaan16]. Antibodies produced by B cells can prevent viral entry into cells and promote phagocytosis of the virus by innate immune cells. IgA is particularly important in blocking transmission of a virus between hosts.
The innate and adaptive immune responses thus have interdependent roles in protection against respiratory viral infections.
Modulation of the innate immune response to respiratory viral infections by vitamin D
The VDR is widely expressed on cells of the immune system and on epithelial cells [Reference Milde18], with a single nucleotide polymorphism in the VDR correlating with the risk of RSV infection [Reference Kresfelder19]. 1,25(OH)2D stimulates neutrophils, macrophages, and NK cells of the respiratory tract, as well as epithelial cells, to produce antimicrobial peptides (AMPs), including defensins and cathelicidins [Reference Beard, Bearden and Striker20]. These AMPs have anti-viral activity with hCAP18/LL-37 in particular having anti-influenza effects [Reference Barlow21].
1,25(OH)2D also influences the innate immune response by increasing TLR and CD14 expression [Reference Beard, Bearden and Striker20], macrophage maturation and oxidative burst capacity [Reference Cannell22]. It therefore enhances the antimicrobial activity of macrophages. The importance of macrophages and neutrophils during the initial interaction of the virus with the host has been demonstrated in mice by increased disease severity following depletion of these cells prior to influenza infection [Reference Tumpey23]. However, despite the enhanced activity of macrophages, autophagy is reduced during the infection [Reference Khare24]. Autophagy, an important process in cellular homeostasis, has a role in cytokine induction. In influenza A virus infection, autophagy is associated with the production of IFN-α and CXCL10, and with increased viral replication [Reference Zhou25]. Thus inhibition of autophagy by vitamin D may be a further mechanism for the control of respiratory viral infections, in addition to controlling pathology in the lung. The effects of vitamin D on the innate immune response also extend to promoting the migration of myeloid dendritic cells to lymphoid organs distant from draining lymph nodes where they activate antigen-specific CD4+ T and B cells [Reference Khoo26, Reference Enioutina, Bareyan and Daynes27].
Vitamin D inhibits the production of pro-inflammatory cytokines. During influenza A infection, IFN-β, tumour necrosis factor (TNF)-α, interleukin (IL)-8, IL-6 and RANTES are all reduced in lung epithelial cells in response to treatment with 1,25(OH)2D [Reference Khare24]. During RSV infection of airway epithelial cells, vitamin D induces IκBα, thus inhibiting NFκB responses [Reference Hansdottir28]. Although such effects may appear counter-productive in the induction of effective host anti-respiratory viral responses, it is recognized that the pathogenicity of respiratory viruses is associated with hypercytokinaemia [Reference de Jong29], also referred to as cytokine storm, which is a potentially fatal self-perpetuating cycle of inflammatory responses. In particular, infection with the more pathogenic strains of influenza results in increased viral replication, neutrophil infiltration and cytokine and chemokine levels, relative to the less pathogenic strains [Reference Tumpey23]. Thus controlling pro-inflammatory responses is beneficial to the host exposed to respiratory viruses. In support, it has been shown that the levels of IL-1β, IL-6, TNF-α, IFN-γ and IL-10 are suppressed on TLR stimulation of human peripheral blood mononuclear cells in the summer months, when cases of respiratory viral infection are at their lowest, compared to the responses in winter [Reference Khoo26].
Modulation of the adaptive immune response to respiratory viral infections by vitamin D
The intriguing immunomodulating effects of vitamin D extend to the adaptive immune response, especially to T cells. 1,25(OH)2D downregulates the induction of both T helper (Th)1- and Th2-associated cytokines when applied during T helper cell activation; only the Th1-associated cytokines are downregulated if 1,25(OH)2D is applied after the establishment of the activated T helper cell subsets [Reference Staeva-Vieira and Freedman30]. This inhibition is at the level of cytokine transcription rather than any effect on cell cycle or Th1-/Th2-associated transcription factors. T cells in VDR knockout mice develop normally but over-produce the pro-inflammatory Th1- [Reference Froicu31] and Th17-associated cytokines [Reference Bruce32], thus substantiating the role of vitamin D in regulating inflammatory cytokines.
Vitamin D can also activate T regulatory (Treg) cell subsets. The VDR binds to a conserved non-coding region of the Treg cell-associated gene FoxP3, and promotes the expression of this gene in T helper cells [Reference Kang33]. This increases the number of Treg cells and their suppressor activity. Furthermore, increased production of the anti-inflammatory cytokine IL-10 by CD4+ T cells has been noted in response to moderate levels of 1,25(OH)2D [Reference Urry34]. Even topical administration of 1,25(OH)2D confers an increase in the suppressive activity of CD4+CD25+ T cells, as reported in an asthma model [Reference Gorman, Judge and Hart35]. Adoptive transfer of Treg cells to lymphocyte-deficient mice, which cannot generate an adaptive immune response, resulted in suppressed innate immune-mediated pathology during influenza infection [Reference Antunes and Kassiotis36]. The recipient mice had delayed weight loss and prolonged survival following infection. Therefore vitamin D has the potential to confer protective responses during respiratory viral infection by its effects on Treg cells.
Vitamin D can modulate both innate and adaptive immune responses by the mechanisms outlined above and VDR polymorphism has been correlated with susceptibility to respiratory viral infections, thus suggesting that vitamin D could provide a therapeutic target for such diseases. The role of vitamin D has been investigated in clinical studies and the results to date are now described.
Observational evidence associating vitamin D status with protection against respiratory viral infections
A number of studies published in the past 10 years have investigated the correlation between vitamin D status, as assessed by 25(OH)D concentration in the serum, and the occurrence of respiratory viral infections. A selection of these publications is outlined in Table 1. The studies were carried out in various countries and settings, with numbers of subjects ranging from fewer than 50 to many thousands. In most instances, the lower the concentrations of 25(OH)D, the higher the risk of respiratory viral infections. However, in the majority of cases, the population examined already had symptoms of the infection at the time of the 25(OH)D measurement. Thus whether low vitamin D status is a contributing factor or a consequence of the infection cannot be distinguished. Indeed in one study involving large numbers of subjects, it was found that a reduction in the risk of infection preceded an increase in 25(OH)D levels [Reference Berry45]. In addition, the infections were rarely diagnosed by laboratory tests but subjectively by self- or parental reporting or by clinical symptoms, making it impossible to attribute any effect of vitamin D status specifically to a viral pathogen. Finally, no studies have examined the possibility that the infections themselves could have an effect on catabolism of 25(OH)D and 1,25(OH)2D, resulting in an apparent vitamin D deficiency.
Table 1. Observational studies associating vitamin D status with risk of respiratory viral infections
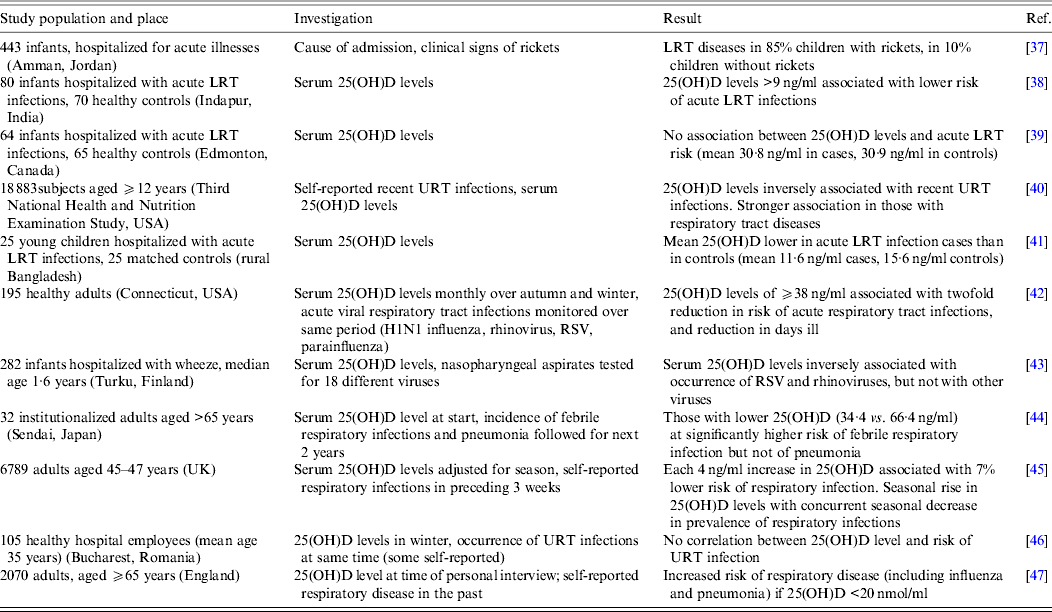
LRT, Lower respiratory tract; RSV, respiratory syncytial virus; URT, upper respiratory tract; 25(OH)D, 25-hydroxyvitamin D.
Whether the risk of respiratory viral infection in a child could be affected by the vitamin D status of the mother has also been investigated. Karatekin et al. [Reference Karatekin48] found that serum 25(OH)D levels were lower in newborns hospitalized with acute LRT infections and their mothers, than in healthy newborns and their mothers. Both Camargo et al. [Reference Camargo49] in New Zealand and Belderbos et al. [Reference Belderbos50] in The Netherlands have monitored 25(OH)D in cord blood and related this prospectively to the occurrence of respiratory infections in the child. In the former study, 882 newborns with <10 ng/ml 25(OH)D in cord blood were twice as likely to develop a respiratory infection (as reported by the mothers) at 3 months compared to those newborns with ⩾30 ng/ml. In the latter study, 156 newborns with <20 ng/ml 25(OH)D in cord blood were six times more likely to develop a LRT infection (as reported by the mother and by the occurrence of RSV-RNA in nose-throat swabs) in the first year of life compared to those with >30 ng/ml 25(OH)D. In addition a multicentre study based in Spain showed that the offspring of mothers, whose 25(OH)D levels were in the highest quartile, had a 23% reduction in their risk of LRT infection during their first year compared to the offspring of mothers in the lowest quartile [Reference Morales51]. These findings demonstrate that a low maternal vitamin D status could adversely affect the development of the fetal immune system, possibly leading to a less effective antiviral response after birth. In addition if the vitamin D content of the mother's breast milk is low, this will result in an insufficient vitamin D status in the child during breastfeeding. Alternatively a higher vitamin D status in the mother may represent a marker for more sun exposure that may have positive effects independent of vitamin D production. It is clearly important to monitor the 25(OH)D levels of children in early life to clarify these possibilities and to make informed recommendations regarding vitamin D supplements during pregnancy and in the first months after birth.
Clinical trials of dietary vitamin D supplements and protection against respiratory viral infections
While studies associating 25(OH)D levels with the risk of respiratory viral infections are described above, this section examines clinical trials of vitamin D3 supplementation in the prevention of respiratory infections. Eight such investigations are summarized in Table 2. In common with the observational studies (Table 1), these reports include a wide range of subjects, in some cases with underlying health problems, living in various parts of the world, who were given vitamin D3 supplements at different doses and frequencies over varying time periods. There was also considerable variation in the measured endpoints with self-reported symptoms being frequent and laboratory identification of specific infectious agents uncommon. In brief, no consistent difference in the risk of respiratory viral infection between those given the supplement and those given the placebo was found. However, there was some evidence, such as reported by Camargo et al. [Reference Camargo58], that the supplement could have a protective effect in some individuals with a low baseline vitamin D status. This could indicate that boosting the 25(OH)D level from deficiency activates various innate and adaptive immune responses that are critical in the control of some respiratory viral infections, while, in contrast, boosting from a higher starting level of 25(OH)D provides no additional benefit.
Table 2. Prospective randomized double-blind trials of vitamin D supplementation and protection against respiratory viral infections

RT, Respiratory tract; URT, upper respiratory tract; 25(OH)D, 25-hydroxyvitamin D.
A novel approach involved subjecting a group of young adults to suberythemal sunbed exposure three times weekly during the winter, while another group ingested vitamin D supplements (1000 IU) daily, and a control group had no intervention [Reference de Gruijl and Pavel60]. The serum 25(OH)D level dropped in the control group, but rose in the other two groups. However, the percentage of subjects in each group who developed a self-reported upper respiratory tract infection did not differ. Thus it was concluded that neither the UVB exposures nor vitamin D supplements lowered the risk of such infection.
Vitamin D and the efficacy of vaccination
As discussed above, 1,25(OH)2D3 has the capacity to induce some anti-viral immune responses and therefore may have the potential to improve the efficacy of vaccination, such as by macrophage activation, monocyte chemotaxis, and migration of myeloid dendritic cells from the site of cutaneous vaccination to multiple lymphoid organs where they stimulate antigen-specific T and B cell responses [Reference Enioutina, Bareyan and Daynes27]. Experiments in adult mice showed that co-administration of 1,25(OH)2D3 with inactivated influenza virus enhanced both the antibody response against the viral haemagglutinin and mucosal immunity [Reference Daynes61]. This approach has been tested in a limited number of human studies thus far, details of which are shown in Table 3. In some cases, the 25(OH)D level in the subjects was assessed, and in other cases vitamin D supplements were administered in controlled trials. In general, the outcomes indicated that neither the induction of seropositivity, nor its degree, were determined by vitamin D status or by ingesting vitamin D supplements, although it should be noted that in all cases the number of subjects was small and frequently they were not healthy. Also serum rather than mucosal immunity was assessed, the latter being of greater importance in the initial interaction between a respiratory virus and the host.
Table 3. Summary of studies relating vitamin D status to the efficacy of vaccination
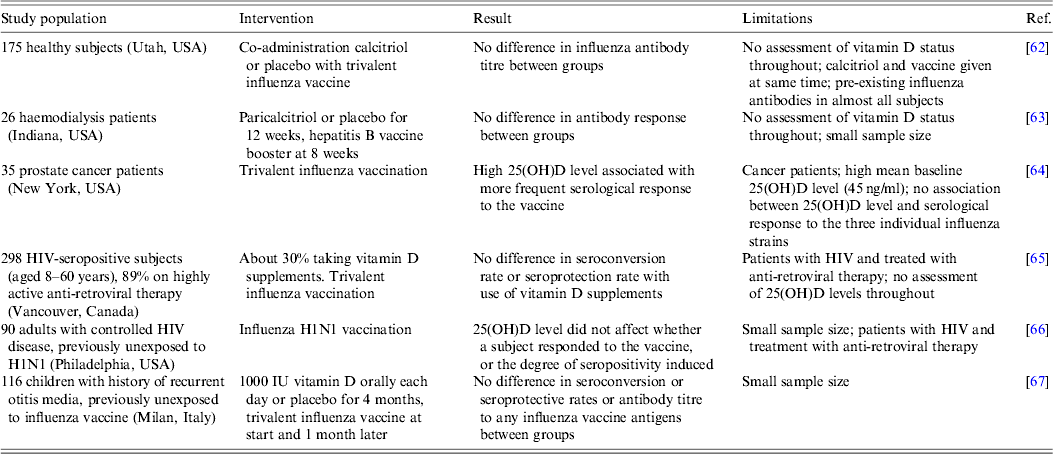
Conclusions
It is clear that 1,25(OH)2D has many immunomodulating properties which could potentially lessen the risk of a symptomatic respiratory viral infection. The majority of the observational studies indicate that low levels of 25(OH)D increased the risk of respiratory viral infections but, in most instances, it was not possible to determine the timing of the infection with respect to the vitamin D status of the individual. In the prospective clinical trials to date, almost all have not demonstrated conclusively that vitamin D supplements influence the risk of respiratory viral infections. However, where the starting 25(OH)D levels were very low, there was some indication of a protective effect from the supplements. The limited studies carried out thus far do not show, in general, that vitamin D status affects the immune response to vaccination.
While many of the studies reviewed here do not demonstrate a clear unambiguous role for vitamin D in protection against respiratory viral infections, the approaches taken provide pointers for the design of future trials. Thus consideration needs to be given to the health, vitamin D status and sample size of the subject group. For example, if the vitamin D status of the group is already sufficient, then it is unlikely that dietary supplements will have any effect. It is also possible that polymorphisms in the VDR, vitamin D binding protein or hydroxylase enzymes could affect the ability of an individual to respond to the vitamin D supplement, thus genotyping may be required to distinguish subgroup effects. In addition the administration and quantity of the dietary supplement is of importance – whether it should be given intermittently at high dose, on a monthly basis, or more frequently at lower dose, on a weekly or daily basis [Reference Hollis and Wagner68]. The length of the study period needs to be assessed, taking into account the seasonal variation in 25(OH)D levels at many latitudes, and that there may be a low frequency of respiratory viral infections in the study population. In large-scale prospective trials not due to be completed for at least 4 years, doses range from 2000 IU daily to 100 000 (2·5 mg) IU vitamin D3 each month. Laboratory-based methods to identify the microorganisms causing the clinical symptoms are required and to explore the possibility that vitamin D might be protective against one type of virus but not another. Finally it is possible, as has been suggested in several recent reports that solar UV radiation may have beneficial effects on health other than through the production of vitamin D [Reference Hart, Gorman and Finlay-Jones69, Reference Milliken70]
It remains a tantalizing, but so far unfulfilled, hope that increasing the vitamin D status of a population could be an effective and inexpensive prophylactic against a range of respiratory viral infections, particularly in those subjects most at risk of severe symptoms, such as young children and the elderly.
Acknowledgements
We thank Dr Kate Templeton (Royal Infirmary of Edinburgh) for her critical review of Figure 1.
Declaration of Interest
None.