Book contents
- Thirst and Body Fluid Regulation
- Thirst and Body Fluid Regulation
- Copyright page
- Contents
- Figures
- Preface
- 1 Fundamentals of Thirst and Body Fluid Regulation
- 2 Body Fluid Compartments, Inputs, and Outputs
- 3 Intracellular Dehydration Thirst and Drinking
- 4 Intracellular Dehydration: Mechanism
- 5 Extracellular Dehydration Thirst and Drinking
- 6 Pregnancy and the Ontogeny of Thirst
- 7 Food-Associated Drinking and Nycthemeral Rhythms
- 8 Hybrid Dehydrations: Water Deprivation
- 9 Hybrid Dehydrations: Thermal Stress and Exercise
- 10 Thirst in Aging and Clinical Populations
- 11 Comparative Aspects of Body Fluid Regulation
- Appendix Methods in Physiology and Neuroscience
- References
- Index
- References
References
Published online by Cambridge University Press: 09 December 2021
- Thirst and Body Fluid Regulation
- Thirst and Body Fluid Regulation
- Copyright page
- Contents
- Figures
- Preface
- 1 Fundamentals of Thirst and Body Fluid Regulation
- 2 Body Fluid Compartments, Inputs, and Outputs
- 3 Intracellular Dehydration Thirst and Drinking
- 4 Intracellular Dehydration: Mechanism
- 5 Extracellular Dehydration Thirst and Drinking
- 6 Pregnancy and the Ontogeny of Thirst
- 7 Food-Associated Drinking and Nycthemeral Rhythms
- 8 Hybrid Dehydrations: Water Deprivation
- 9 Hybrid Dehydrations: Thermal Stress and Exercise
- 10 Thirst in Aging and Clinical Populations
- 11 Comparative Aspects of Body Fluid Regulation
- Appendix Methods in Physiology and Neuroscience
- References
- Index
- References
Summary
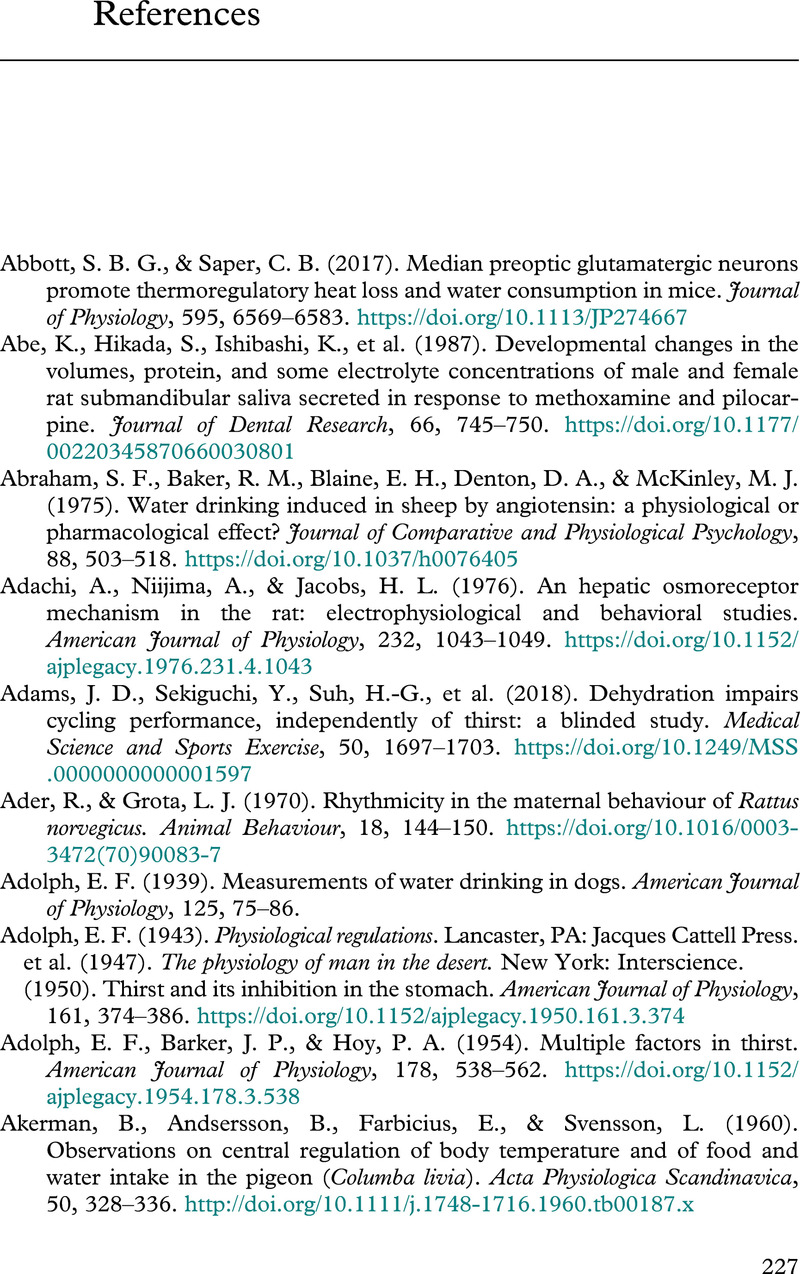
- Type
- Chapter
- Information
- Thirst and Body Fluid RegulationFrom Nephron to Neuron, pp. 227 - 270Publisher: Cambridge University PressPrint publication year: 2021