Part II - Marsh Dynamics
Published online by Cambridge University Press: 19 June 2021
Summary
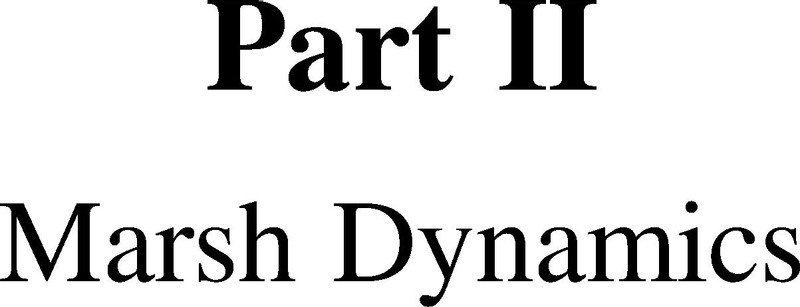
- Type
- Chapter
- Information
- Salt MarshesFunction, Dynamics, and Stresses, pp. 155 - 334Publisher: Cambridge University PressPrint publication year: 2021