Book contents
- Principles of Glacier Mechanics
- Reviews
- Principles of Glacier Mechanics
- Copyright page
- Dedication
- Contents
- Preface to the third edition
- Preface to the second edition
- Preface to the first edition
- Physical constants relevant to ice
- Derived SI units and conversion factors
- 1 Why study glaciers?
- 2 Some basic concepts
- 3 Mass balance
- 4 Flow and fracture of a crystalline material
- 5 The velocity field in a glacier
- 6 Temperature distribution in polar ice sheets
- 7 The coupling between a glacier and its bed
- 8 Water flow in and under glaciers: Geomorphic implications
- 9 Stress and deformation
- 10 Stress and velocity distribution in an idealized glacier
- 11 Numerical modeling
- 12 Applications of stress and deformation principles to classical problems
- 13 Ice streams and ice shelves
- 14 Finite strain and the origin of foliation
- 15 Response of glaciers to climate change
- 16 Ice core studies
- Problems
- References
- Index
- References
References
Published online by Cambridge University Press: 20 December 2019
- Principles of Glacier Mechanics
- Reviews
- Principles of Glacier Mechanics
- Copyright page
- Dedication
- Contents
- Preface to the third edition
- Preface to the second edition
- Preface to the first edition
- Physical constants relevant to ice
- Derived SI units and conversion factors
- 1 Why study glaciers?
- 2 Some basic concepts
- 3 Mass balance
- 4 Flow and fracture of a crystalline material
- 5 The velocity field in a glacier
- 6 Temperature distribution in polar ice sheets
- 7 The coupling between a glacier and its bed
- 8 Water flow in and under glaciers: Geomorphic implications
- 9 Stress and deformation
- 10 Stress and velocity distribution in an idealized glacier
- 11 Numerical modeling
- 12 Applications of stress and deformation principles to classical problems
- 13 Ice streams and ice shelves
- 14 Finite strain and the origin of foliation
- 15 Response of glaciers to climate change
- 16 Ice core studies
- Problems
- References
- Index
- References
Summary
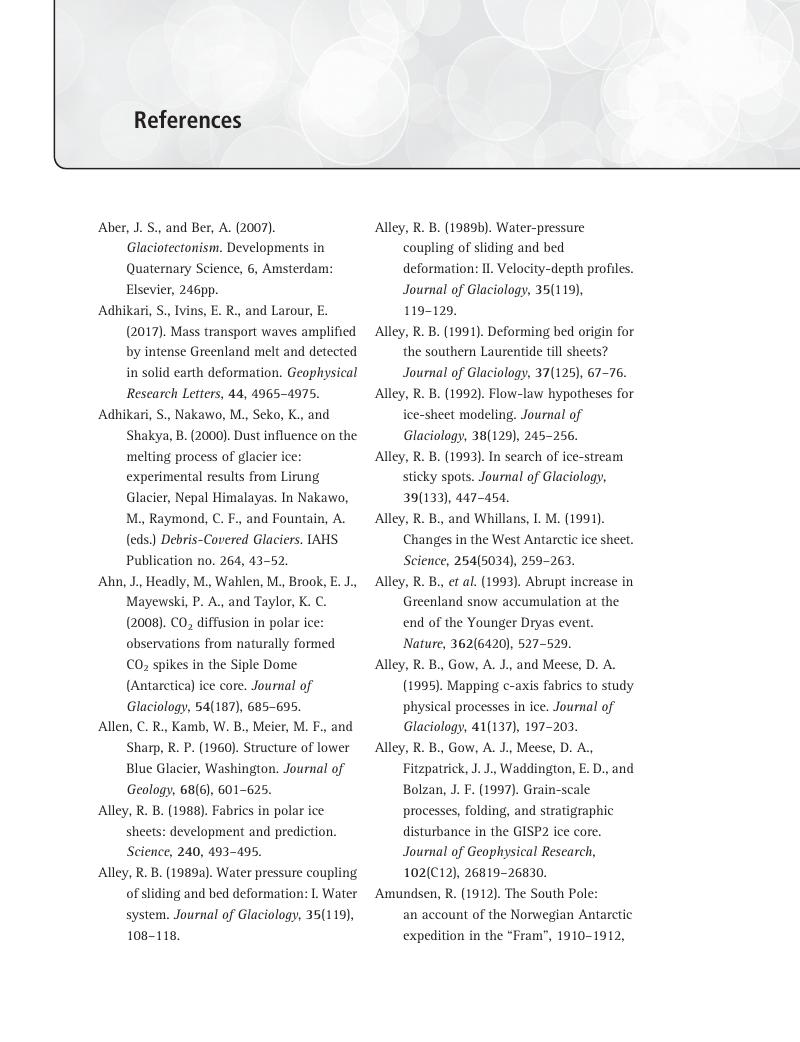
- Type
- Chapter
- Information
- Principles of Glacier Mechanics , pp. 465 - 505Publisher: Cambridge University PressPrint publication year: 2019