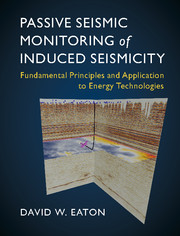
Book contents
- Frontmatter
- Contents
- Preface
- List of Symbols
- Part I Fundamentals of Passive Seismic Monitoring
- 1 Constitutive Relations and Elastic Deformation
- 2 Failure Criteria and Anelastic Deformation
- 3 Seismic Waves and Sources
- 4 Stress Measurement and Hydraulic Fracturing
- Part II Applications of Passive Seismic Monitoring
- Appendix A Glossary
- Appendix B Signal-Processing Essentials
- Appendix C Data Formats
- References
- Index
4 - Stress Measurement and Hydraulic Fracturing
from Part I - Fundamentals of Passive Seismic Monitoring
Published online by Cambridge University Press: 07 June 2018
- Frontmatter
- Contents
- Preface
- List of Symbols
- Part I Fundamentals of Passive Seismic Monitoring
- 1 Constitutive Relations and Elastic Deformation
- 2 Failure Criteria and Anelastic Deformation
- 3 Seismic Waves and Sources
- 4 Stress Measurement and Hydraulic Fracturing
- Part II Applications of Passive Seismic Monitoring
- Appendix A Glossary
- Appendix B Signal-Processing Essentials
- Appendix C Data Formats
- References
- Index
Summary
But though he is confined to its crust, he may penetrate into all its secrets.
Jules Verne (The Steamhouse, Part II, 1881)Hydraulic fracturing is a reservoir-stimulation method used to create permeable pathways deep into a rock formation, thereby enhancing fluid transport within the reservoir medium (Smith and Shlyapobersky, 2000). Extensive deployment of this technology for development of low-permeability unconventional hydrocarbon reservoirs has had a “gamechanging” effect on world markets (Montgomery and Smith, 2010). Simply put, a hydraulic fracture is initiated when engineered fluids are pumped into a wellbore at a rate that is faster than the fluids can escape into the formation, resulting in a pressure buildup that ultimately surpasses the tensile strength of the rockmass. Initiation and growth of a hydraulically stimulated fracture network is usually accompanied by brittle rock-failure processes that can be monitored using passive-seismic (microseismic) methods (Maxwell, 2014). Passiveseismic monitoring is also the primary surveillance technology for induced seismicity (Shapiro, 2015), which in rare instances can be caused by hydraulic fracturing (Bao and Eaton, 2016).
Numerical simulation of hydraulic fracturing is widely used for hydraulic fracturing and completion design (Adachi et al., 2007). The in situ stress field exerts a first-order control on evolution of a hydraulic-fracture network; hence, a robust and accurate model of the stress state is required in order to achieve meaningful results. The first part of this chapter provides a concise overview of methods that are used to determine components of the stress tensor in the subsurface. Stress is difficult to measure directly, so most methods are based upon indirect inference of stress from induced strain observations, coupled with knowledge of the constitutive properties of the medium. Next, this chapter summarizes salient aspects of technology that is used for hydraulic fracturing of low-permeability rock formations. Taken together, these concepts are foundational for understanding practical applications of passive-seismic monitoring that are covered in the following chapters.
How Subsurface Stress Is Determined
The in-situ state of stress in the subsurface represents a complex superposition of interacting stresses due to overburden and tectonic forces, as well as gradients in temperature and pore pressure arising from natural and anthropogenic factors (Amadei and Stephansson, 1997).
- Type
- Chapter
- Information
- Passive Seismic Monitoring of Induced SeismicityFundamental Principles and Application to Energy Technologies, pp. 95 - 126Publisher: Cambridge University PressPrint publication year: 2018