Book contents
- Frontmatter
- Contents
- List of illustrations
- List of tables
- Preface
- Acknowledgements
- 1 Astrophysical information
- 2 Photometry
- 3 Positional astronomy
- 4 Fourier transforms
- 5 Detection systems
- 6 Orthodox statistics
- 7 Stochastic processes and noise
- 8 Optics
- 9 Interference
- 10 Spectroscopy
- 11 Ultraviolet, x-ray, and gamma ray astronomy
- 12 Radio receivers, spectrometers, and interferometers
- 13 Modern statistical methods
- 14 Neutrino detectors
- 15 Cosmic ray detectors
- 16 Gravitational waves
- 17 Polarimetry
- Appendix A Physical constants and units
- Appendix B Acronyms
- Appendix C Additional reading
- References
- Index
- Plates
- References
References
Published online by Cambridge University Press: 05 June 2012
- Frontmatter
- Contents
- List of illustrations
- List of tables
- Preface
- Acknowledgements
- 1 Astrophysical information
- 2 Photometry
- 3 Positional astronomy
- 4 Fourier transforms
- 5 Detection systems
- 6 Orthodox statistics
- 7 Stochastic processes and noise
- 8 Optics
- 9 Interference
- 10 Spectroscopy
- 11 Ultraviolet, x-ray, and gamma ray astronomy
- 12 Radio receivers, spectrometers, and interferometers
- 13 Modern statistical methods
- 14 Neutrino detectors
- 15 Cosmic ray detectors
- 16 Gravitational waves
- 17 Polarimetry
- Appendix A Physical constants and units
- Appendix B Acronyms
- Appendix C Additional reading
- References
- Index
- Plates
- References
Summary
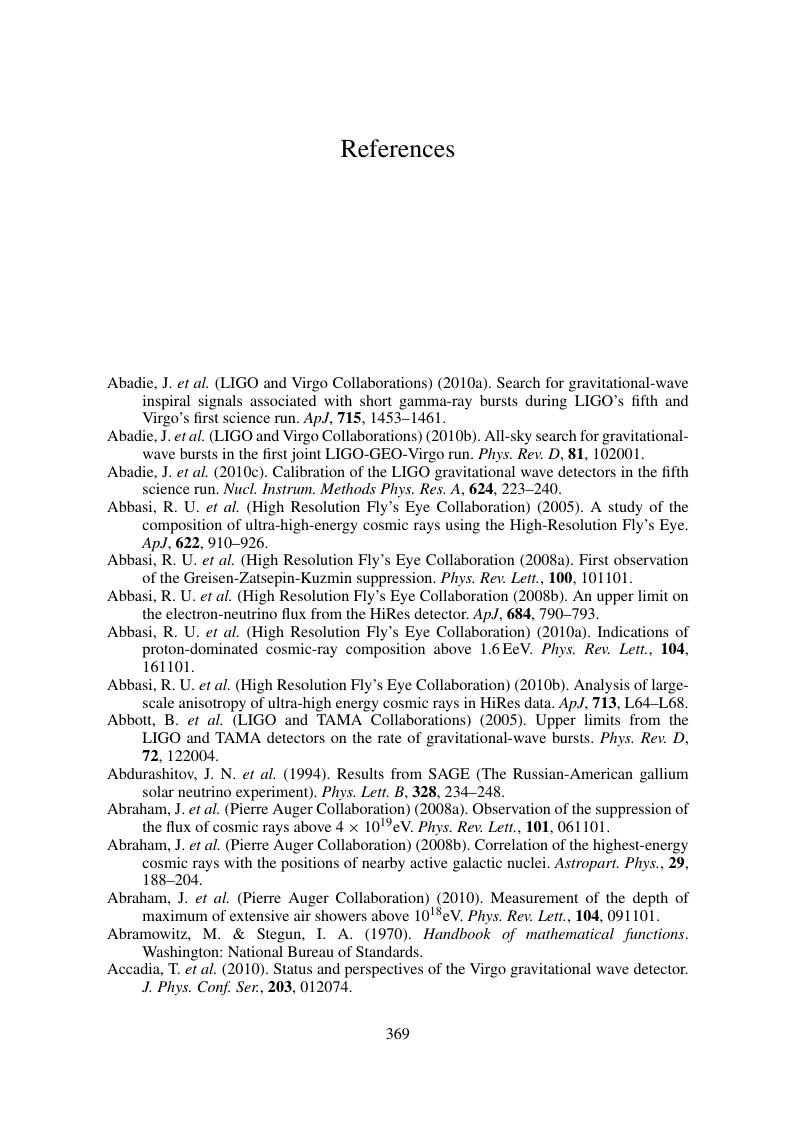
- Type
- Chapter
- Information
- Observational AstronomyTechniques and Instrumentation, pp. 369 - 377Publisher: Cambridge University PressPrint publication year: 2011