Book contents
- Frontmatter
- Contents
- Preface
- Publishers' Note
- Chapter 1 Structural organization of the nervous system
- Chapter 2 Resting and action potentials
- Chapter 3 The ionic permeability of the nerve membrane
- Chapter 4 Membrane permeability changes during excitation
- Chapter 5 Voltage-gated ion channels
- Chapter 6 Cable theory and saltatory conduction
- Chapter 7 Neuromuscular transmission
- Chapter 8 Synaptic transmission in the nervous system
- Chapter 9 The mechanism of contraction in skeletal muscle
- Chapter 10 The activation of skeletal muscle
- Chapter 11 Contractile function in skeletal muscle
- Chapter 12 Cardiac muscle
- Chapter 13 Smooth muscle
- Further reading
- References
- Index
- References
References
Published online by Cambridge University Press: 05 June 2012
- Frontmatter
- Contents
- Preface
- Publishers' Note
- Chapter 1 Structural organization of the nervous system
- Chapter 2 Resting and action potentials
- Chapter 3 The ionic permeability of the nerve membrane
- Chapter 4 Membrane permeability changes during excitation
- Chapter 5 Voltage-gated ion channels
- Chapter 6 Cable theory and saltatory conduction
- Chapter 7 Neuromuscular transmission
- Chapter 8 Synaptic transmission in the nervous system
- Chapter 9 The mechanism of contraction in skeletal muscle
- Chapter 10 The activation of skeletal muscle
- Chapter 11 Contractile function in skeletal muscle
- Chapter 12 Cardiac muscle
- Chapter 13 Smooth muscle
- Further reading
- References
- Index
- References
Summary
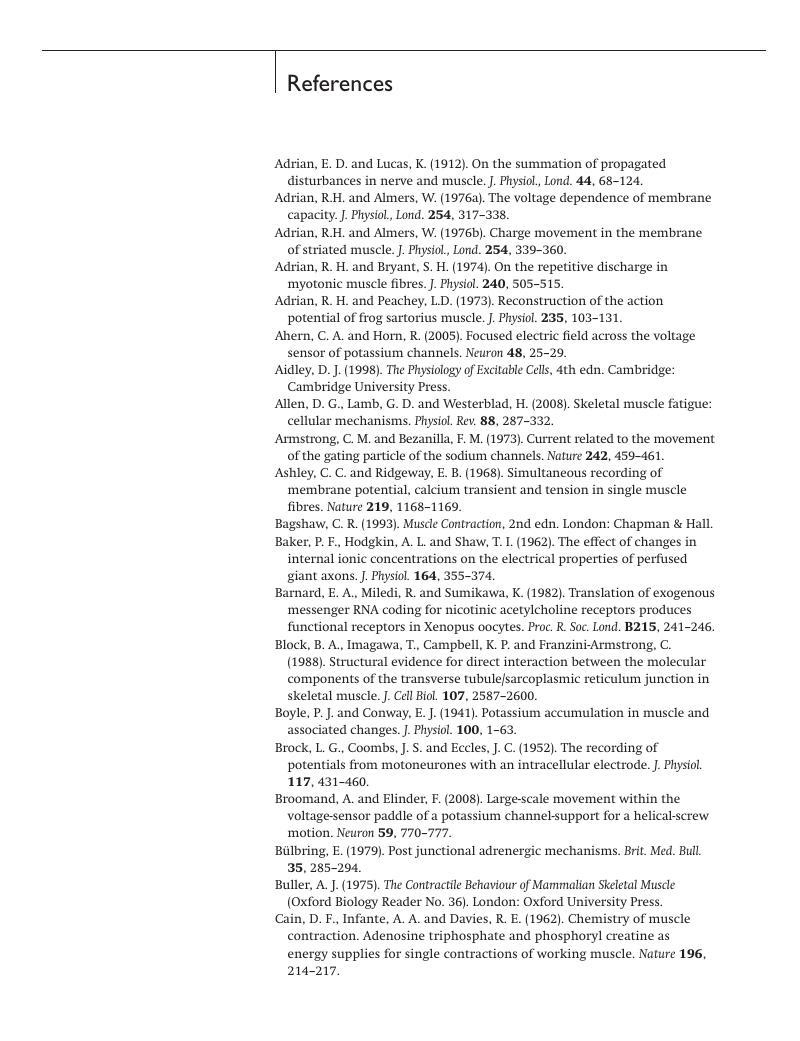
- Type
- Chapter
- Information
- Nerve and Muscle , pp. 170 - 177Publisher: Cambridge University PressPrint publication year: 2011