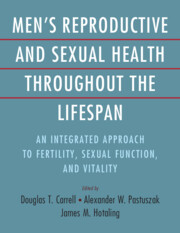
Book contents
- Men’s Reproductive and Sexual Health throughout the Lifespan
- Men’s Reproductive and Sexual Health throughout the Lifespan
- Copyright page
- Contents
- Contributors
- Preface
- Section 1 An Introduction to Men’s Health Care
- Section 2 The Biology of Male Reproduction and Infertility
- Section 3 Clinical Evaluation and Treatment of Male Infertility
- Section 4 Laboratory Evaluation and Treatment of Male Infertility
- Chapter 20 The Modern Semen Analysis
- Chapter 21 The Future of Computer-Assisted Semen Analysis in the Evaluation of Male Infertility
- Chapter 22 Reactive Oxygen Species and Sperm DNA Damage
- Chapter 23 Clinical Value of Sperm DNA Fragmentation Tests
- Chapter 24 The Current Use of Sperm Function Assays
- Chapter 25 Sperm Selection in the Laboratory
- Chapter 26 Methods to Select Ejaculated, Epididymal, and Testicular Spermatozoa for Assisted Conception
- Chapter 27 Optimal Sperm Selection in the ICSI Era
- Chapter 28 Microfluidics for Sperm Sample Preparation and Sperm Identification
- Chapter 29 Practical Concerns for Patient Semen Banking
- Chapter 30 The Potential Future Applications of In Vitro Spermatogenesis in the Clinical Laboratory
- Chapter 31 Spermatogonial Stem Cell Culture and the Future of Germline Gene Editing
- Section 5 Medical and Surgical Management of Issues of Male Health
- Index
- References
Chapter 30 - The Potential Future Applications of In Vitro Spermatogenesis in the Clinical Laboratory
from Section 4 - Laboratory Evaluation and Treatment of Male Infertility
Published online by Cambridge University Press: 06 December 2023
- Men’s Reproductive and Sexual Health throughout the Lifespan
- Men’s Reproductive and Sexual Health throughout the Lifespan
- Copyright page
- Contents
- Contributors
- Preface
- Section 1 An Introduction to Men’s Health Care
- Section 2 The Biology of Male Reproduction and Infertility
- Section 3 Clinical Evaluation and Treatment of Male Infertility
- Section 4 Laboratory Evaluation and Treatment of Male Infertility
- Chapter 20 The Modern Semen Analysis
- Chapter 21 The Future of Computer-Assisted Semen Analysis in the Evaluation of Male Infertility
- Chapter 22 Reactive Oxygen Species and Sperm DNA Damage
- Chapter 23 Clinical Value of Sperm DNA Fragmentation Tests
- Chapter 24 The Current Use of Sperm Function Assays
- Chapter 25 Sperm Selection in the Laboratory
- Chapter 26 Methods to Select Ejaculated, Epididymal, and Testicular Spermatozoa for Assisted Conception
- Chapter 27 Optimal Sperm Selection in the ICSI Era
- Chapter 28 Microfluidics for Sperm Sample Preparation and Sperm Identification
- Chapter 29 Practical Concerns for Patient Semen Banking
- Chapter 30 The Potential Future Applications of In Vitro Spermatogenesis in the Clinical Laboratory
- Chapter 31 Spermatogonial Stem Cell Culture and the Future of Germline Gene Editing
- Section 5 Medical and Surgical Management of Issues of Male Health
- Index
- References
Summary
In this review we reflect on the many attempts highlighting key achievements in the field of in vitro spermatogenesis made so far. The research in this field is at a crucial juncture. The evolving technologies (like biofabricated 3D organoids, 3D bioprinting, microfluidics, or organ-on-a-chip) may offer excellent tools and in vitro testicular model systems to advance our understanding and bridge the existing knowledge gaps. Each of these culture systems offers unique advantages and may complement each other to address the common goal of achieving primate spermatogenesis in vitro. There are various possibilities and future scenarios for applying in vitro spermatogenesis as a tool for research and clinical applications in the future.
Keywords
- Type
- Chapter
- Information
- Men's Reproductive and Sexual Health Throughout the LifespanAn Integrated Approach to Fertility, Sexual Function, and Vitality, pp. 229 - 243Publisher: Cambridge University PressPrint publication year: 2023