Book contents
- Marijuana and Madness
- Marijuana and Madness
- Copyright page
- Contents
- Contributors
- Preface
- Part I Pharmacology of Cannabis and the Endocannabinoid System
- Part II The Changing Face of Cannabis
- Part III Cannabis and the Brain
- Part IV Cannabis, Anxiety, and Mood
- Part V Cannabis and Psychosis
- Part VI Cannabinoids and Schizophrenia: Aetiopathology and Treatment Implications
- Part VII Cannabinoids and Schizophrenia: Aetiopathology and Treatment Implications
- Part VIII Special Topics
- Index
- References
Part I - Pharmacology of Cannabis and the Endocannabinoid System
Published online by Cambridge University Press: 12 May 2023
- Marijuana and Madness
- Marijuana and Madness
- Copyright page
- Contents
- Contributors
- Preface
- Part I Pharmacology of Cannabis and the Endocannabinoid System
- Part II The Changing Face of Cannabis
- Part III Cannabis and the Brain
- Part IV Cannabis, Anxiety, and Mood
- Part V Cannabis and Psychosis
- Part VI Cannabinoids and Schizophrenia: Aetiopathology and Treatment Implications
- Part VII Cannabinoids and Schizophrenia: Aetiopathology and Treatment Implications
- Part VIII Special Topics
- Index
- References
Summary
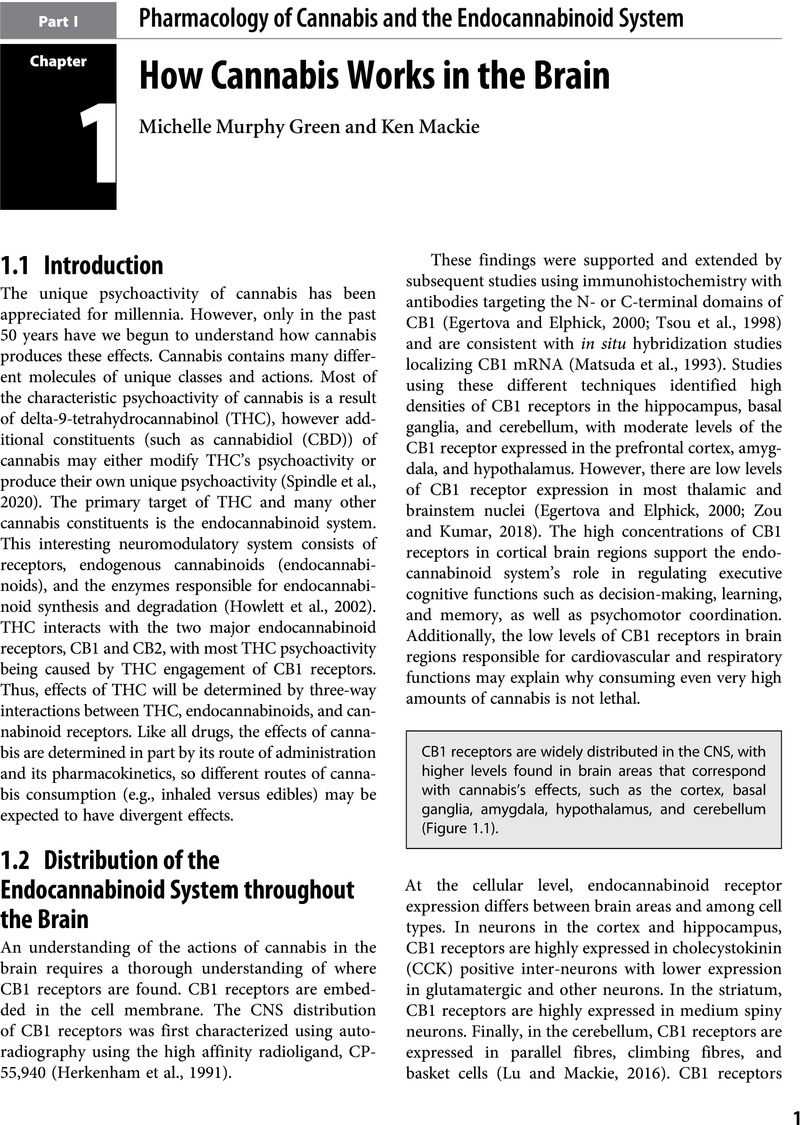
- Type
- Chapter
- Information
- Marijuana and Madness , pp. 1 - 30Publisher: Cambridge University PressPrint publication year: 2023