Book contents
- Keynes & Aidley’s Nerve and Muscle
- Keynes & Aidley’s Nerve and Muscle
- Copyright page
- Dedication
- Contents
- Preface
- Acknowledgements
- Abbreviations used in the text
- 1 Structural Organisation of the Nervous System
- 2 Resting and Action Potentials
- 3 Background Ionic Homeostasis of Excitable Cells
- 4 Membrane Permeability Changes During Excitation
- 5 Voltage-Gated Ion Channels
- 6 Cable Theory and Saltatory Conduction
- 7 Neuromuscular Transmission
- 8 Synaptic Transmission in the Nervous System
- 9 The Mechanism of Contraction in Skeletal Muscle
- 10 The Activation of Skeletal Muscle
- 11 Excitation–Contraction Coupling in Skeletal Muscle
- 12 Contractile Function in Skeletal Muscle
- 13 Cardiac Muscle
- 14 Ion Channel Function and Cardiac Arrhythmogenesis
- 15 Smooth Muscle
- Further Reading
- References
- Index
- References
References
Published online by Cambridge University Press: 07 November 2020
- Keynes & Aidley’s Nerve and Muscle
- Keynes & Aidley’s Nerve and Muscle
- Copyright page
- Dedication
- Contents
- Preface
- Acknowledgements
- Abbreviations used in the text
- 1 Structural Organisation of the Nervous System
- 2 Resting and Action Potentials
- 3 Background Ionic Homeostasis of Excitable Cells
- 4 Membrane Permeability Changes During Excitation
- 5 Voltage-Gated Ion Channels
- 6 Cable Theory and Saltatory Conduction
- 7 Neuromuscular Transmission
- 8 Synaptic Transmission in the Nervous System
- 9 The Mechanism of Contraction in Skeletal Muscle
- 10 The Activation of Skeletal Muscle
- 11 Excitation–Contraction Coupling in Skeletal Muscle
- 12 Contractile Function in Skeletal Muscle
- 13 Cardiac Muscle
- 14 Ion Channel Function and Cardiac Arrhythmogenesis
- 15 Smooth Muscle
- Further Reading
- References
- Index
- References
Summary
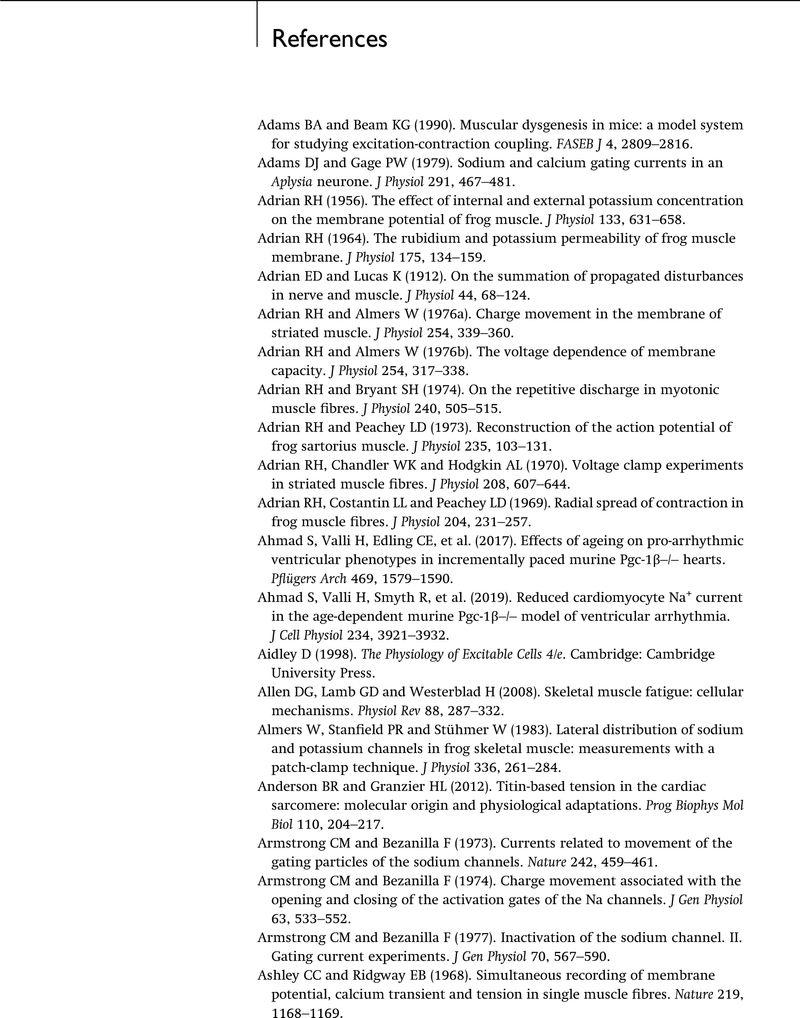
- Type
- Chapter
- Information
- Keynes & Aidley's Nerve and Muscle , pp. 274 - 291Publisher: Cambridge University PressPrint publication year: 2020