Book contents
- Insect Diapause
- Insect Diapause
- Copyright page
- Dedication
- Contents
- Preface
- 1 Confronting the Challenges of a Seasonal Environment
- 2 Which Seasons Are Being Avoided?
- 3 Variation in the Diapause Response
- 4 The Cost of Diapause and Some Diapause Alternatives
- 5 Interpreting Seasonal Cues to Program Diapause Entry
- 6 Preparing for Diapause
- 7 The Diapause State
- 8 Ending Diapause and Reinitiating Development
- 9 Molecular Signaling Pathways that Regulate Diapause
- 10 Genetic Control of Diapause
- 11 Evolution of Diapause
- 12 Wider Implications
- References
- Species Index
- Subject Index
- Plate Section (PDF Only)
- References
References
Published online by Cambridge University Press: 13 January 2022
- Insect Diapause
- Insect Diapause
- Copyright page
- Dedication
- Contents
- Preface
- 1 Confronting the Challenges of a Seasonal Environment
- 2 Which Seasons Are Being Avoided?
- 3 Variation in the Diapause Response
- 4 The Cost of Diapause and Some Diapause Alternatives
- 5 Interpreting Seasonal Cues to Program Diapause Entry
- 6 Preparing for Diapause
- 7 The Diapause State
- 8 Ending Diapause and Reinitiating Development
- 9 Molecular Signaling Pathways that Regulate Diapause
- 10 Genetic Control of Diapause
- 11 Evolution of Diapause
- 12 Wider Implications
- References
- Species Index
- Subject Index
- Plate Section (PDF Only)
- References
Summary
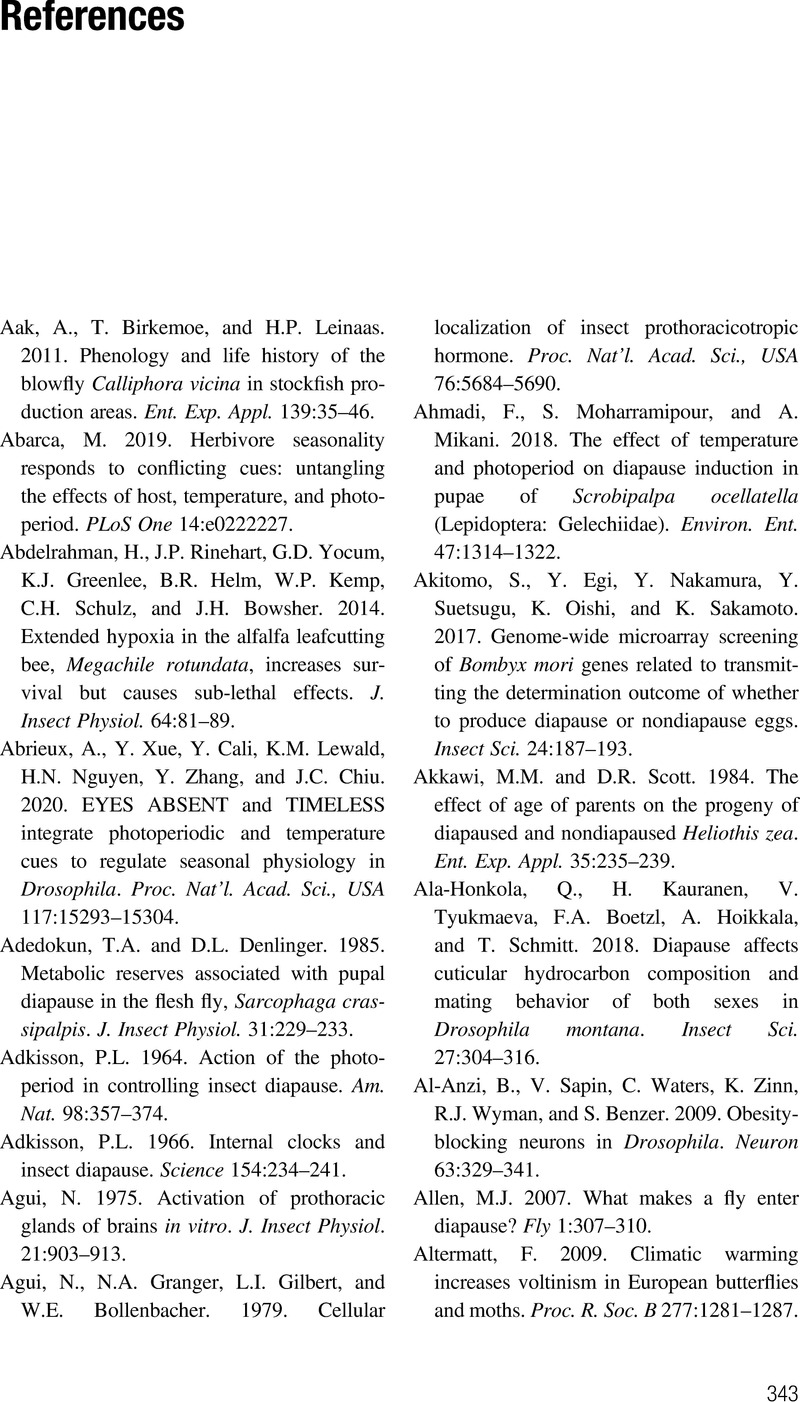
- Type
- Chapter
- Information
- Insect Diapause , pp. 343 - 441Publisher: Cambridge University PressPrint publication year: 2022