Part II - Processes
Published online by Cambridge University Press: 05 September 2015
Summary
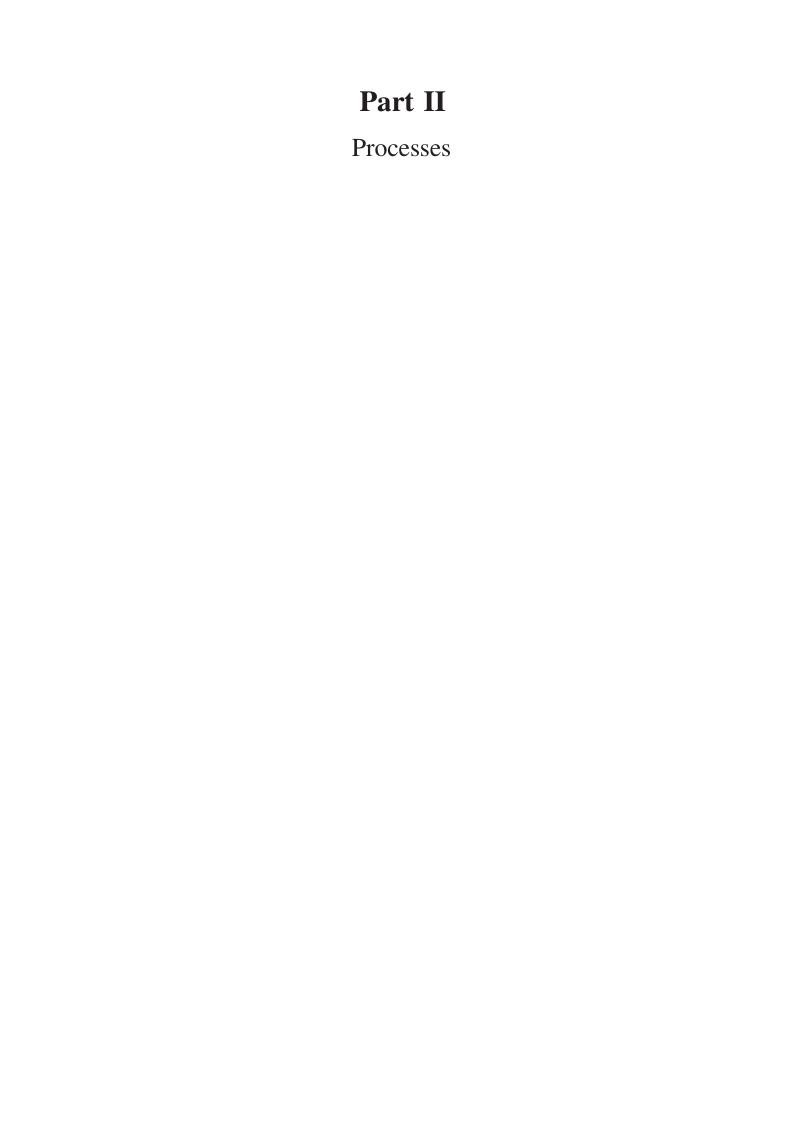
- Type
- Chapter
- Information
- The High-Mountain CryosphereEnvironmental Changes and Human Risks, pp. 107 - 246Publisher: Cambridge University PressPrint publication year: 2015