Book contents
- The Evolution of Senescence in the Tree of Life
- The Evolution of Senescence in the Tree of Life
- Copyright page
- Dedication
- Contents
- Contributors
- Acknowledgements
- 1 Introduction: Wilting Leaves and Rotting Branches
- Part I Theory of Senescence
- Part II Senescence in Animals
- Part III Senescence in Plants
- Part IV Senescence in Microbes
- 17 Why Some Fungi Senesce and Others Do Not
- 18 Yeast Ageing
- 19 Organismal Senescence in Plant–Fungal Symbioses
- Part V Senescence across the Tree of Life
- Index
- References
18 - Yeast Ageing
Reproduction Strategies Determine the Longevity of Budding and Fission Yeasts
from Part IV - Senescence in Microbes
Published online by Cambridge University Press: 16 March 2017
- The Evolution of Senescence in the Tree of Life
- The Evolution of Senescence in the Tree of Life
- Copyright page
- Dedication
- Contents
- Contributors
- Acknowledgements
- 1 Introduction: Wilting Leaves and Rotting Branches
- Part I Theory of Senescence
- Part II Senescence in Animals
- Part III Senescence in Plants
- Part IV Senescence in Microbes
- 17 Why Some Fungi Senesce and Others Do Not
- 18 Yeast Ageing
- 19 Organismal Senescence in Plant–Fungal Symbioses
- Part V Senescence across the Tree of Life
- Index
- References
Summary
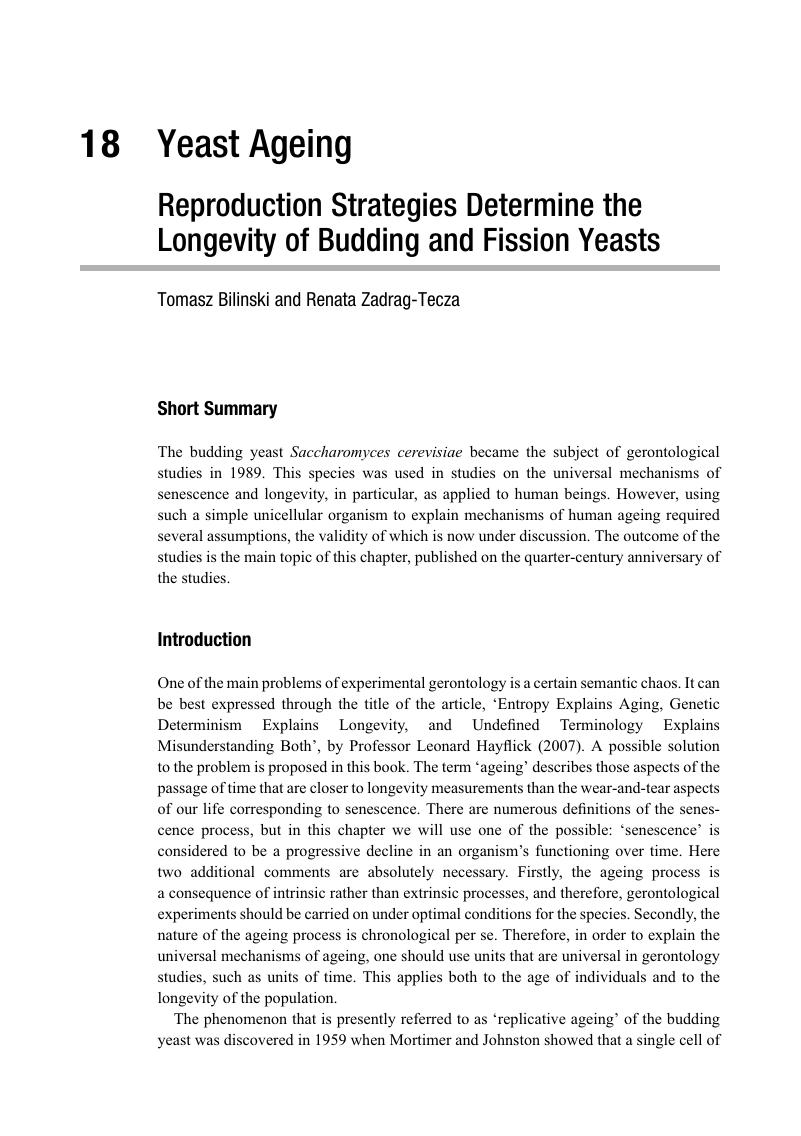
- Type
- Chapter
- Information
- The Evolution of Senescence in the Tree of Life , pp. 362 - 380Publisher: Cambridge University PressPrint publication year: 2017
References
- 1
- Cited by