Book contents
- Applied Nanophotonics
- Applied Nanophotonics
- Copyright page
- Dedication
- Contents
- Preface
- Notation
- Acronyms
- 1 Introduction
- Part I Basics
- 2 Electrons in potential wells and in solids
- 3 Quantum confinement effects in semiconductors
- 4 Lightwaves in restricted geometries
- 5 Spontaneous emission of photons and lifetime engineering
- 6 Stimulated emission and lasing
- 7 Energy transfer processes
- Part II Advances and challenges
- Index
- References
4 - Lightwaves in restricted geometries
from Part I - Basics
Published online by Cambridge University Press: 23 November 2018
- Applied Nanophotonics
- Applied Nanophotonics
- Copyright page
- Dedication
- Contents
- Preface
- Notation
- Acronyms
- 1 Introduction
- Part I Basics
- 2 Electrons in potential wells and in solids
- 3 Quantum confinement effects in semiconductors
- 4 Lightwaves in restricted geometries
- 5 Spontaneous emission of photons and lifetime engineering
- 6 Stimulated emission and lasing
- 7 Energy transfer processes
- Part II Advances and challenges
- Index
- References
Summary
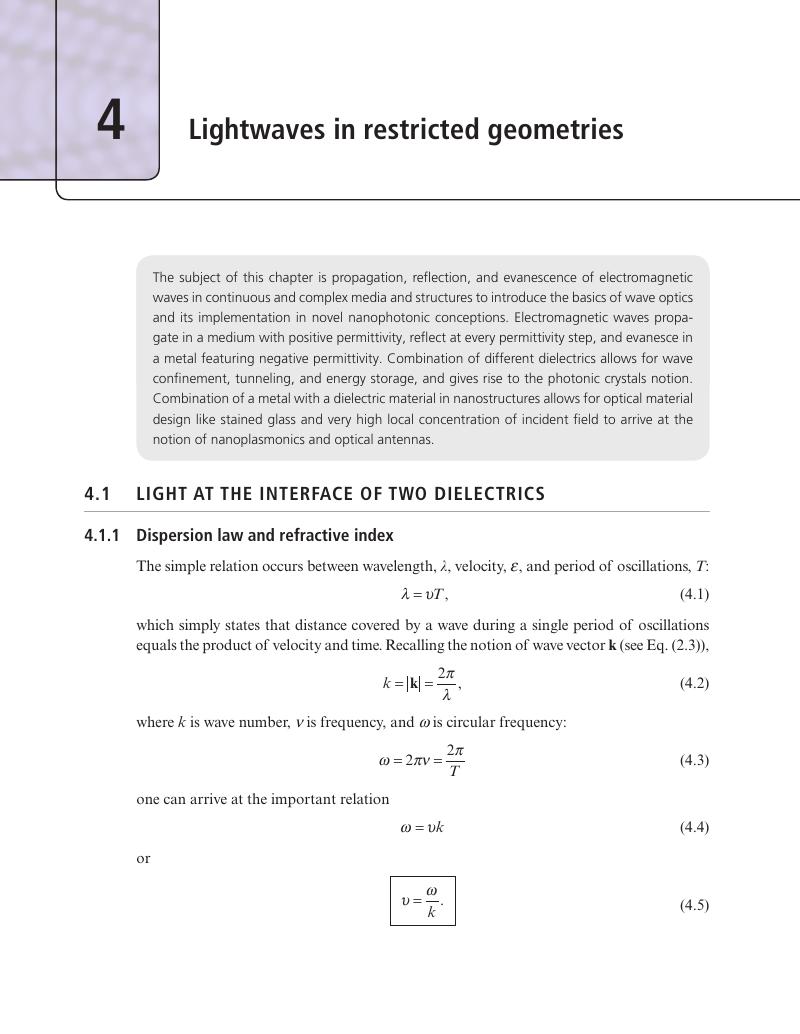
- Type
- Chapter
- Information
- Applied Nanophotonics , pp. 92 - 146Publisher: Cambridge University PressPrint publication year: 2018
References
Further reading
References
- 1
- Cited by