Iodine is an essential trace element for the synthesis of thyroid hormones (TH). TH play an important role in growth and development, metabolism, and body organ function. Exposure to an abnormal iodine environment will seriously affect iodine homoeostasis and TH production(Reference Glinoer1). Moderate or severe iodine deficiency during pregnancy can lead to hypothyroidism in the mother and infant, impaired fetal neurological development, and increased risk of miscarriage and congenital abnormalities in the fetus(Reference Zimmermann2). In addition to the adverse effects of iodine deficiency, epidemiological studies have shown that excessive iodine exposure in early pregnancy is associated with an increased risk of maternal subclinical hypothyroidism and hypothyroxinemia(Reference Shi, Han and Li3). In the presence of large iodine loads, fetal hypothyroidism and goitre also occur(Reference Castro, Werner and Marinho4,Reference Pearce, Lazarus and Moreno-Reyes5) .
The fetal thyroid accumulates iodine and synthesises TH at 11 weeks(Reference De Felice and Di Lauro6). Thyroid cells actively accumulate I- against their concentration gradient using a Na+-driven force generated by the sodium/iodide symporter (NIS). Subsequently, I- is transported through the Ap membrane to the colloid, the thyroid lumen, by a group of transporters, including Pendrin, cystic fibrosis transmembrane conductance regulator and anoctamin 1(Reference Ravera, Reyna-Neyra and Ferrandino7). At the same time, studies have shown that the mRNA of NIS and Pendrin are both expressed in the human placenta for the accumulation of iodide in the placenta, but the expression level is lower than that in the thyroid tissue(Reference Sun, Han and Qian8). NIS is highly expressed in the apical layer of the syncytiotrophoblast and regulates iodine uptake into the syncytiotrophoblast, whereas Pendrin is localised in the brush border membrane of syncytiotrophoblast cells and plays a role in iodine efflux(Reference Bidart, Lacroix and Evain-Brion9). Studies in rats have shown that the expression of the iodine transporter Pendrin is increased during iodine deficiency to store more iodine and prevent maternal iodine deficiency from affecting fetal health. Compensatory NIS protein regulation in the placenta against decreased iodine intake mainly occurs during translation and glycosylation modification after translation(Reference Sun, Han and Qian8). There is a lack of research on how iodine transporters in the placenta change when iodine is excessive.
Act as a storage site supplying iodine to the fetus(Reference Burns, O’Herlihy and Smyth10), the placenta also provides iodine and TH to the fetus in the third trimester(Reference Adu-Gyamfi, Wang and Ding11). In pregnant women with excess dietary iodine intake, the placenta also acts as a barrier for maternal–fetal exchange, preventing harmful substances from reaching the fetus, and allowing trace elements, hormones and other substances to be transported from the mother to the fetus. In pregnant women with insufficient dietary iodine intake, the placenta may maintain the iodine supply of the fetus to defend against the harm of insufficient maternal dietary iodine intake(Reference Burns, O’Herlihy and Smyth10). Animal experiments have also shown that when iodine status is abnormal, iodine transporters such as NIS and Pendrin will have corresponding expression changes. For example, the expression of NIS is up-regulated in the placenta of rats fed a low-iodine diet(Reference Schröder-van der Elst, van der Heide and Kastelijn12). Pendrin and glycosylated NIS were significantly up-regulated in a dose-dependent manner to ingest more iodine into the placenta, which may represent an adaptive mechanism that protects the fetus from insufficient maternal iodine intake(Reference Neven, Cox and Vrijens13). However, the role of this adaptive mechanism in the human placenta remains to be further confirmed.
In this study, sixty pregnant women were selected and divided into different iodine nutritional statuses according to urinary iodine concentration (UIC) and serum iodine concentration (SIC). To explore the mechanism of iodine transport in the placenta of pregnant women with different iodine nutritional statuses, it is planned to test the correlation between serum and placenta TH levels and preliminarily explore the transport rules of TH in serum and placenta.
Methods and materials
Subjects and basis of grouping
Sixty pregnant women were recruited in Wuqing Second People’s Hospital from June 2021 to September 2021. This study was conducted according to the guidelines laid down in the Declaration of Helsinki, and all procedures involving human subjects/patients were approved by the Medical Ethics Committee of Tianjin Medical University (TMUhMEC2020033). Written informed consent was obtained from all subjects. The trial was registered at www.clinicaltrials.gov as NCT04492657. Baseline information (age, height, weight and gestational week) was collected at admission. Participants were required to have resided locally for more than 5 years and have no thyroid disease or other chronic conditions. Pregnant women which took iodine supplements or thyroid-related medications could not be included in the study. The subjects were informed of the procedure and purpose of the study and signed the informed consent. Fig. 1 illustrates the selection of subjects for the study.
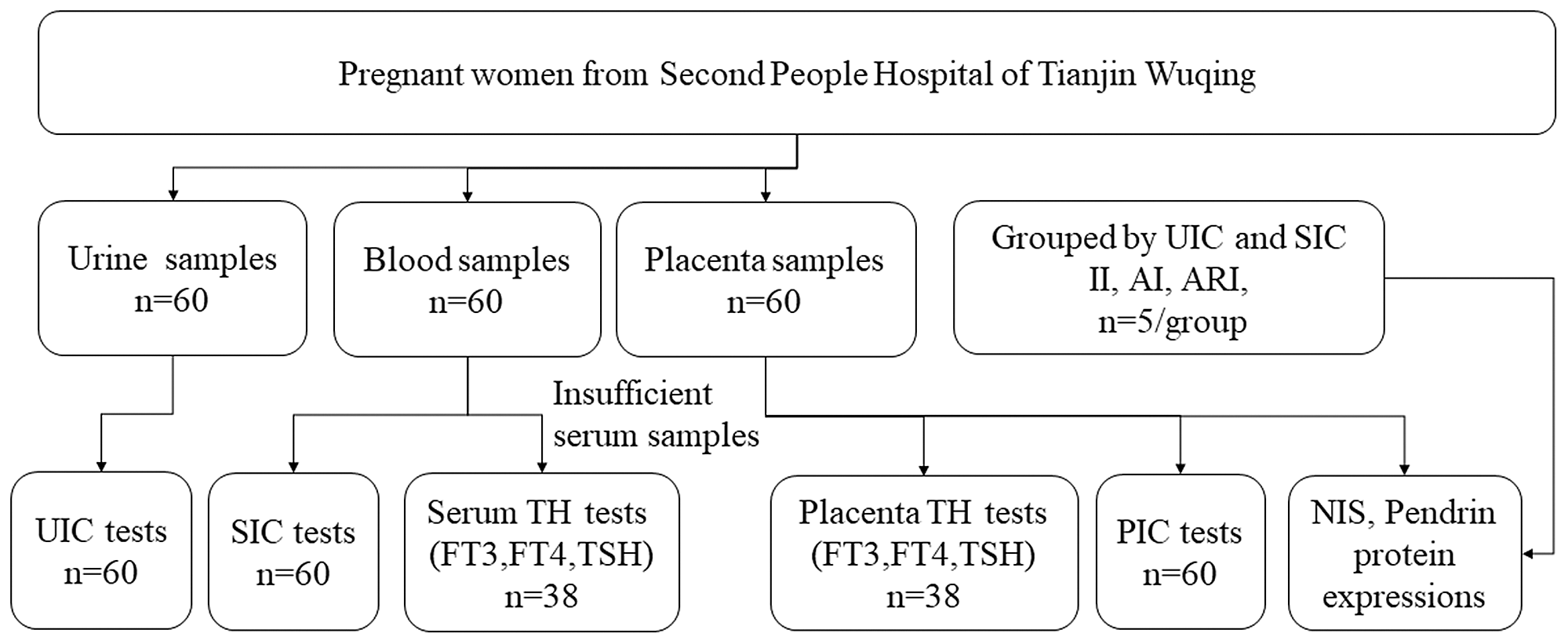
Fig. 1. Flow chart for participant selection in the study. UIC, urinary iodine concentration; SIC, serum iodine concentration; PIC, placental iodine concentration; TH, thyroid hormones; FT3, free triiodothyronine; FT4, free tetraiodothyronine; TSH, thyroid-stimulating hormone.
We plan to group the pregnant according to the results of UIC and SIC, and five pregnant women were selected as insufficient intake (II), adequate intake (AI) and above requirements intake (ARI) (details of the groups were shown in online Supplementary Table 1). The samples in the II were selected according to the overlap of the top 25 % of UIC and SIC, the AI group was selected according to the overlap of the middle 50 % of UIC and SIC, and the samples in the ARI were selected according to the overlap of the last 25 % of UIC and SIC.
Collection of blood and urine samples
Each participant was informed to collect her spot urine samples (mid-stream urine), using 5 ml of iodine-free centrifuge tubes. The urine samples were sealed and stored in the refrigerator at −80°C. Venous blood was stood for 2 h before centrifuging (3000–5000 g, room temperature, 10 min), and the separated serum was placed at −80°C refrigerators for cryopreservation. The samples were stored for a maximum of 1 month before analysis.
Urinary iodine concentration
UIC was analysed by inductively coupled plasma MS (ICP-MS; iCAP Q, Thermo Fisher Scientific). ICP-MS was operated with argon (> 99·999 %, high purity) in kinetic energy discrimination for the determination of elemental iodine. The flow rate of argon gas was set as follows: auxiliary: 0·8 l/min; nebuliser: 1·11 l/min; and cooling gas flow rate: 14·00 l/min. The isotopes used for the measurements were 127I and 130Te. The standard working solution of urine iodine is prepared by diluting the potassium iodate solution with urine iodine diluent. The concentration gradient is as follows: 0 μg/l, 5 μg/l, 10 μg/l, 20 μg/l, 30 μg/l, 50 μg/l, 100 μg/l, 150 μg/l, 200 μg/l, 300 μg/l, 400 μg/l, 500 μg/l, 750 μg/l and 1000 μg/l. Urine samples were diluted 21-fold with 0·25 % tetramethylammonium hydroxide, 0·02 % Triton X-100 and 100 mg/l Te before analysis using ICP-MS. The total inter-assay and intra-assay CV% for UIC measurements were 1·4–3·2 and 0·6–1·8 %, respectively.
Serum iodine concentration
SIC was analysed by ICP-MS with the use of Te for mass bias correction. Serum samples were diluted with 1·5 % isopropanol and 7 mmol of hydrous ammonium before analysis by the ICP-MS. The calibration curve was linear from 1 to 1000 μg/l. Standard freeze-dried human serum material (ref. 201413, Seronorm) was tested with every batch of serum samples as quality control. The mean iodine concentration of the standard reference material was 71·7 ± 2·2 μg/l, which is consistent with the certificated value of 71·8 μg/l. Intra-assay and inter-assay CV were 1·3 % (n 12) and 3·2 % (n 20), respectively.
Placenta collection and iodine detection
The placenta tissue was removed within 15 min after delivery of the placenta. As shown in Fig. 2, a piece of tissue of 2 cm2 is taken from the centre, the edge and the middle area (or annular area) of the line connecting the two through three points. Macroscopic necrosis, infarcts and calcifications were avoided while collecting specimens. The removed tissue was rinsed repeatedly with sterilised saline, and then the water was absorbed with sterilised gauze to remove the blood in the placenta tissue.

Fig. 2. Schematic diagram of placenta sampling.
The iodine concentration of the placenta was detected and analysed by ICP-MS using Te for mass bias correction. The samples were digested overnight with tetramethylammonium hydroxide before measurement and baked at 90°C for 3–4 h the next day and then diluted with Te and distilled water. Tissue taken from all three regions of the maternal and fetal sides was measured, and the placenta iodine concentration (PIC) was expressed as an average. Placental iodine storage was calculated by multiplying the mean iodine concentration of the six sites (three regions of the maternal and fetal sides) by the mass of the placenta.
Serum thyroid function tests
Serum-free triiodothyronine (FT3), free thyroxine (FT4), thyroid-stimulating hormone (TSH), thyroglobulin antibody (TgAb) and serum thyroid peroxidase antibody (TPOAb) were measured using an automatic Immulite analyser with a chemiluminescent kit (Sophonix). Before testing the sample, each kit was controlled with a calibration solution and quality control product. Hundred millilitres of calibration solution and quality control product were added to each reagent. Samples were tested only after successful calibration and quality control, and each item requires 100 μl of serum.
Placenta thyroid hormone test detection
The placenta tissue was washed away and then homogenised in 1 × PBS (300 mg tissue/300 μl PBS). After performing three freeze–thaw cycles to break the cell membranes, the homogenates were centrifuged at 5000 g for 5 min at 4°C. The supernatant was collected, and the concentrations of TSH, FT3 and FT4 were measured using an ELISA Kit (Jianglaibio) according to the manufacturer’s instructions. Total protein content was detected by bicinchoninic acid, and the concentrations of FT3, FT4 and TSH of the placenta maternal side and fetal side were detected by ELISA and were calibrated by total protein content, that is, the ELISA result was divided by the total protein concentration.
Western blotting analysis
Western blotting was expected to analyse protein expressions in the placenta. Membrane proteins of the placenta were obtained with the Membrane and Cytosol Protein Extraction Kit (Beyotime). Phenylmethylsulfonyl fluoride (Beyotime) was prepared separately and added to the extraction reagent 2–3 min before the reagent was added to the sample. Protein concentrations were measured by a bicinchoninic acid Protein Assay kit (Beyotime). Protein samples were fractionated through sodium dodecyl sulfate – polyacrylamide gel electrophoresis and transferred onto polyvinylidene difluoride (PVDF) membranes (PVDF; Millipore). The PVDF membranes were blocked with 5 % bovine albumin (Sigma) in 1 × TRIS-buffered saline Tween for 1 h at room temperature. Then the membranes were incubated with primary antibody of mouse anti-NIS (1:500; Santa Cruz), rabbit anti-Pendrin (1:500; Santa Cruz) and rabbit anti-GAPDH (Loading Control, 1:5000; Bioss) overnight at 4°C. After washing with tris buffered saline with tween, membranes were incubated with relational horseradish peroxidase (HRP)-conjugated secondary antibodies for 1 h at room temperature. Then, the proteins were detected by Sparkjade ECL super (Shandong Sparkjade) and observed using a ChemiDocTM XRS + Imaging System (Bio-Rad). The protein levels were quantified by densitometry using NIH ImageJ 1.61 Software (National Institutes of Health).
Immunohistochemistry
The placenta samples were fixed with 4 % paraformaldehyde immediately and then embedded in paraffin when they were removed. Sections were dewaxed and rehydrated, and the next steps were performed with rabbit-specific HRP/DAB (ABC) IHC Detection Kit ab64261 (Abcam). The placenta was incubated with primary antibody of rabbit anti-NIS (1:100; Proteintech) and rabbit anti-SLC26A4 (1:100; Santa Cruz), overnight at 4°C. Then the biotinylated secondary antibody was bound to the primary antibody, and the HRP-labelled streptavidin was bound to the secondary antibody. The HRP produced a brown coloured substance at the site of primary antibody binding by reacting with DAB. The images were obtained with an inverted microscope (IX81; Olympus).
Statistical analysis
The statistical software package SPSS Statistics version 20.0 and GraphPad Prism v7 (GraphPad Software Inc.) were used for statistical analysis. The Kolmogorov–Smirnov test was used to analyse the normality of continuous variable data. The variables conforming to a normal distribution were expressed as means and standard deviation. Comparisons between different groups were performed by a one-way ANOVA, followed by a least significant difference (LSD) test for multiple comparisons. The variables without normal distributions were expressed as Mean (P25, P75), and comparisons between groups were performed by a Kruskal–Wallis test. Pearson’s correlation coefficient was used to determine the correlation between UIC or SIC with the placenta iodine storage (PIS). Spearman’s correlation coefficient was used to determine the correlation between serum TH and placenta TH. P < 0·05 was considered statistically significant.
Results
Basic information about pregnant women
A total of sixty pregnant women were surveyed in this study. The baseline characteristics of the study population are shown in Table 1. The age, weight, BMI and gestational week of subjects were 28 (25·0, 32·0) years old, 74·4 ± 11·6 kg, 28·6 ± 4·2 kg/m2 and 39·6 ± 1·2 week, respectively. The mean ± sd of UIC and SIC were 227·6 ± 146·2 μg/l and 63·1 ± 22·1 μg/l, respectively.
Table 1. Basic information of pregnant women
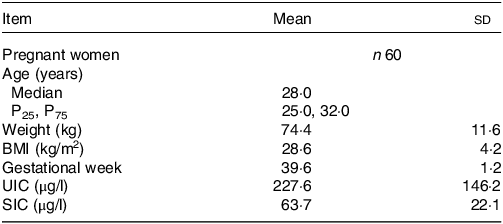
UIC, urinary iodine concentration; SIC, serum iodine concentration.
Normal data were described as mean ± sd; non-normal data were described as Median (P25, P75).
The placental iodine level of pregnant women with different iodine nutritional status
The PIS showed a positive correlation trend with the UIC (R = 0·58, P < 0·001, Fig. 3(a)) and SIC (R = 0·55, P < 0·001, Fig. 3(b)). According to the results of UIC and SIC, pregnant women were divided into the II, AI and ARI group (n 5/group). The levels of the PIC and the PIS in II, AI and ARI groups were shown in Fig. 3(c) and Fig. 3(d). PIS was significantly higher in the ARI group than in the AI group (P = 0·017).
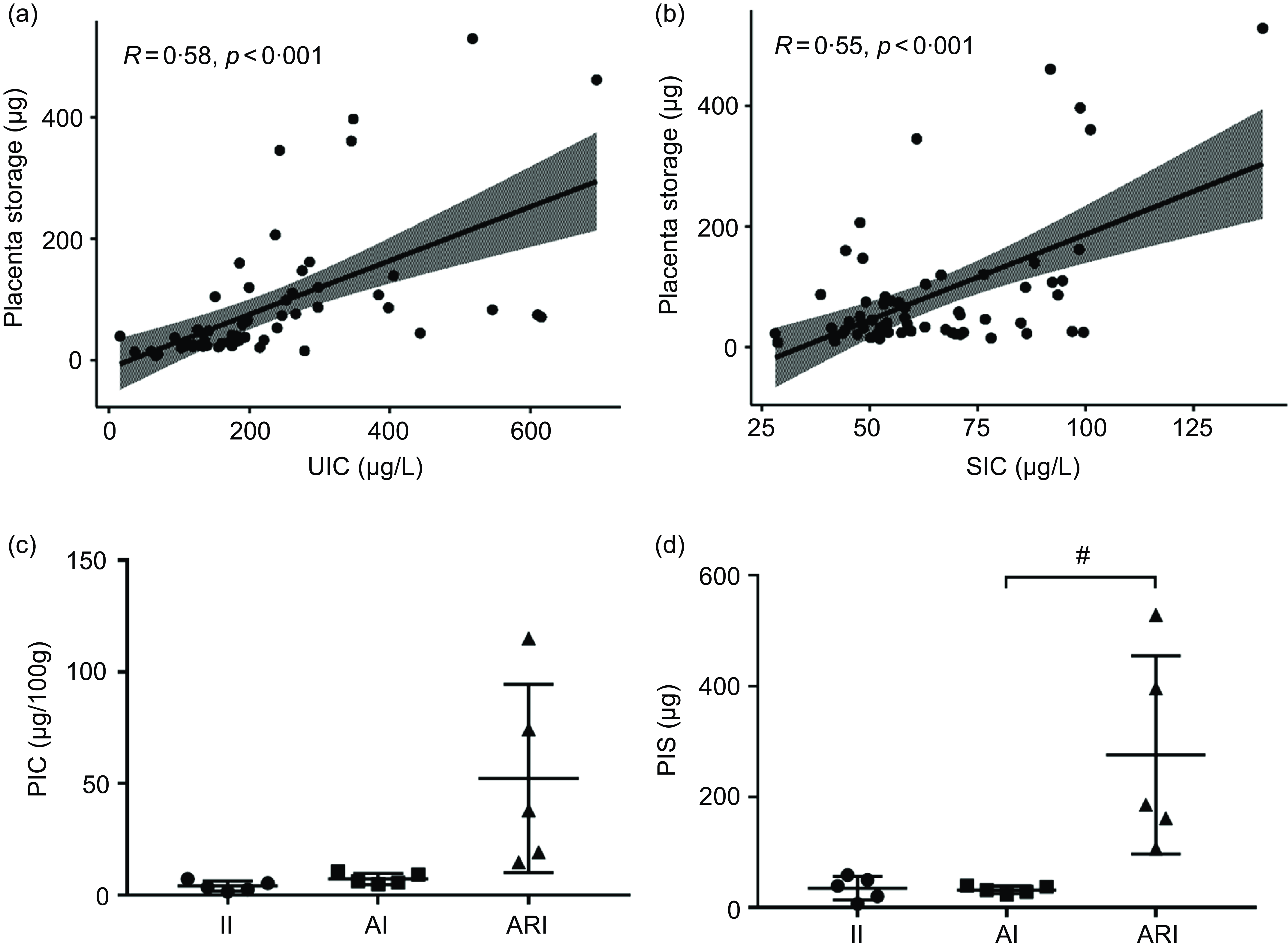
Fig. 3. Correlation of the UIC (a) and SIC (b) with the placenta storage. The levels of PIC (c) and PIS (d) in II, AI and ARI groups. II, insufficient intake; AI, adequate intake; ARI, above requirements intake. UIC, urinary iodine concentration; SIC, serum total iodine concentration; PIC, placental iodine concentration; PIS, placental iodine storage. (Data are expressed as mean ± sd, n 5 per group, # P < 0·05 compared with the AI group.).
Expression of the placenta transporter in pregnant women with different iodine nutritional status
Iodine transporters in the placenta tissues from both maternal and fetal sides were determined by immunocytochemistry. NIS and Pendrin were expressed in both the maternal and fetal sides of the placenta. NIS was highly expressed at the apical side of the syncytiotrophoblast layers of the placenta (Fig. 4(a)). Pendrin was detected in the syncytiotrophoblast and cytotrophoblast layers of the placenta (Fig. 4(b)). The general protein expressions of NIS and Pendrin are shown in Fig. 4(c). On the maternal side (m) of the placenta, the expression of NIS in the mARI group was significantly higher than that in the mAI group. On the fetal side (f) of the placenta, the expression of NIS in fII group was significantly higher than that in the fAI group. In the II group, the expression of NIS on the fetal side was significantly higher than that on the maternal side (P < 0·05, Fig. 4(d)). The Pendrin expression level was significantly higher in the mII group than in the mAI group (P < 0·05, Fig. 4(e)).

Fig. 4. Localisation of NIS (a) and Pendrin (b) in the placenta. Scale bars: 50 μm (c) representative western blot results of NIS and Pendrin. Bar graphs show the semiquantitative levels of NIS (d) and Pendrin (e) determined by band density analysis. (Maternal side, m; fetal side, f; insufficient intake, II; adequate intake, AI; above requirements intake, ARI. Values were shown as mean ± sd. n 5 per group. Comparisons among different groups were performed by one-way ANOVA and followed by LSD test for multiple comparisons. # P < 0·05 compared with the AI group.). NIS, sodium/iodide symporter.
Consistency analysis between thyroid hormone in serum and placenta maternal and fetal sides
Serum and placenta TSH, FT3 and FT4 concentrations were shown in Table 2. Serum TSH, FT3 and FT4 were detected by Chemiluminescence, and placenta TSH, FT3 and FT4 were detected by ELISA. In this study, we also analysed the association between serum TH and placenta TH. As exhibited in Fig. 5(a), there is a positive correlation between the FT3 of the placenta maternal side (mFT3) and FT3 of the fetal maternal side (fFT3) (r = 0·44, P = 0·0067). TSH of the placenta maternal side (mTSH) was positively correlated with TSH of the placenta fetal side (fTSH) (r = 0·75, P < 0·001, Fig. 5(b)). However, there was no correlation between serum and placenta TH.
Table 2. TH concentrations in serum, placenta maternal and placenta fetal measurements

TH, thyroid hormones; TSH, thyroid-stimulating hormone; FT3, free triiodothyronine; FT4, free tetraiodothyronine.
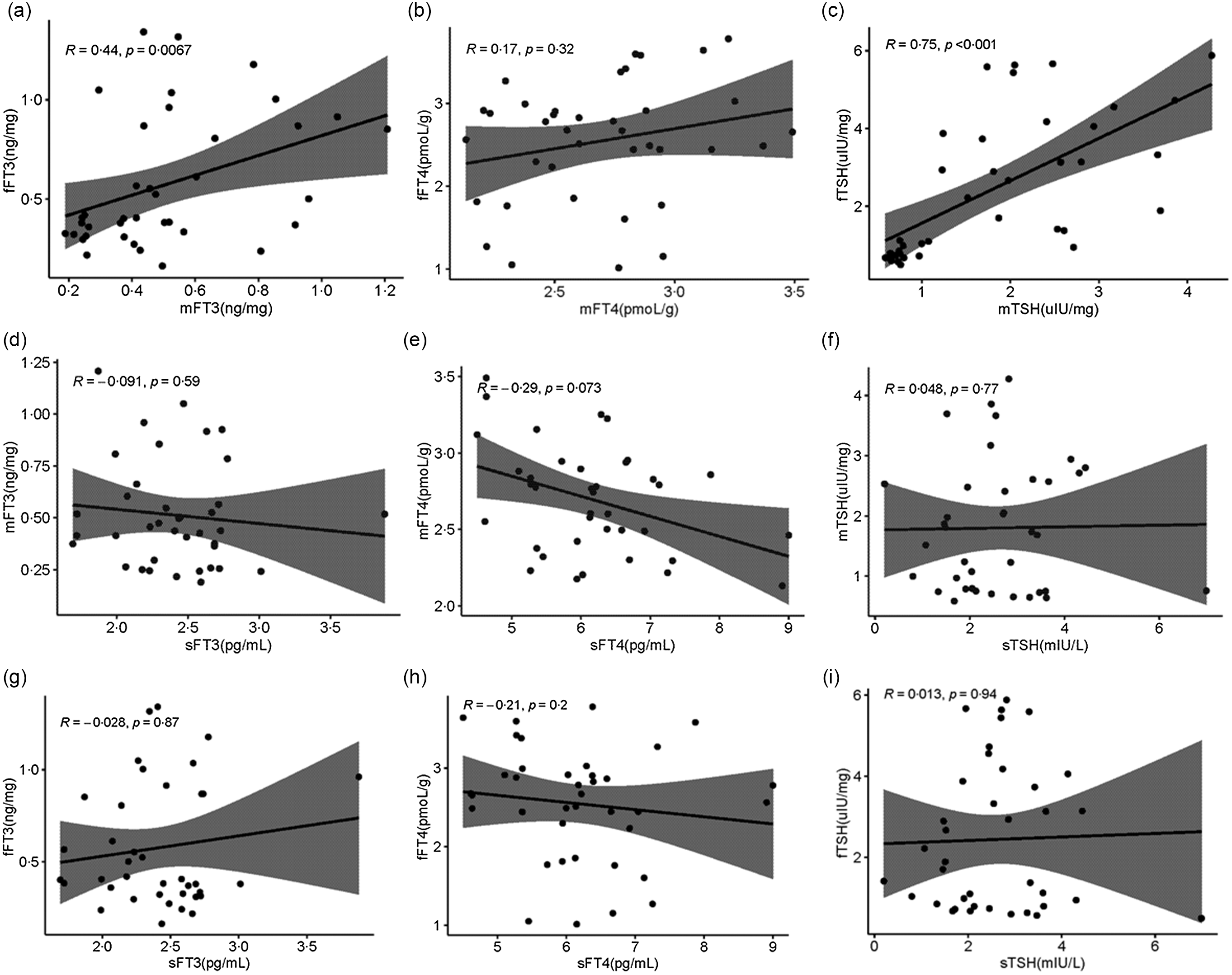
Fig. 5. Distribution of TH in serum, placenta maternal side and placenta fetal side. Correlation of the mFT3 with the fFT3 (a), mFT4 with the fFT4 (b), mTSH with the fTSH (c), sFT3 with the mFT3 (d), sFT4 with the mFT4 (e), sTSH with the mTSH (f), sFT3 with the fFT3 (g), sFT4 with the fFT4 (h), and sTSH with the fTSH (i). mFT3, free triiodothyronine of placenta maternal surface, fFT3, free triiodothyronine of placenta fetal side; mFT4, free tetraiodothyronine of placenta maternal side; fFT4, free tetraiodothyronine of placenta fetal side; mTSH, thyroid-stimulating hormone of placenta maternal side; fTSH, thyroid-stimulating hormone of placenta fetal side; sFT3, free triiodothyronine of serum; sFT4, free tetraiodothyronine of serum; sTSH, thyroid-stimulating hormone of serum.
Discussion
Several recent studies have shown that the placenta could store iodine(Reference Peng, Li and Xie14). Our study first demonstrated a significant difference in levels of the placenta iodine between pregnant women with different iodine nutritional statuses. When the iodine intake was insufficient, the expression of NIS on the fetal side of the placenta increased and the expression of Pendrin on the maternal side of the placenta increased; when the iodine intake was above requirements (but is not excessive), the expression of NIS on the maternal side of the placenta increased. We also tested serum and placenta TH and found no correlation between serum and placenta TH.
The placenta has a role not only in uptake but also in storing iodine as a possible means of protecting the fetus from inadequacies in maternal dietary iodine intake(Reference Burns, O’Herlihy and Smyth10). Iodine may play a role in redox balance, and iodine deprivation induces a significant increase of reactive oxygen species. Research shows that iodine is important for keeping the proliferation/differentiation balance in the placenta(Reference Olivo-Vidal, Rodríguez and Arroyo-Helguera15,Reference la Peña Sol, Isela and Zendy16) . NIS is expressed in various placental and ovular components, and its expression is to remain constant during the three trimesters of pregnancy(Reference Di Cosmo, Fanelli and Tonacchera17). It has been shown that the placental NIS expression is regulated by human chorionic gonadotropin hormone(Reference Arturi, Lacroix and Presta18). In an in vitro model, the human placental villus cancer cells choriocarcinoma cell line human chorionic gonadotropin up-regulated NIS expression at both the mRNA and protein levels(Reference Arturi, Lacroix and Presta18). Although many studies have examined the dietary iodine status of mothers and fetuses/newborns, there are few data on the role of the placenta in iodine transport and storage(Reference Gulaboglu, Borekci and Halici19,Reference Glinoer20) . The delivery of iodine to the fetus depends not only on an adequate supply of iodine in the maternal diet but also on the proper functioning of the placenta transport system. The iodide transporters, NIS and Pendrin, have recently been identified in both placental cytotrophoblasts and syncytiotrophoblasts(Reference Bidart, Lacroix and Evain-Brion9,Reference Mitchell, Manley and Morris21) . In iodine-deficient rats, compensatory NIS protein regulation of placental iodine intake reduction occurred mainly during translation and post-translational glycosylation modification. Pendrin may be more important than NIS in mediating placental iodide transport(Reference Sun, Han and Qian8). Our study also confirmed that the expression of Pendrin on the maternal side of the placenta was significantly increased when iodine was deficient in the human placenta. We also found that the expression of NIS on the fetal side of the placenta in the II group was significantly higher than that in the AI group, and the expression of NIS on the fetal side of the II group was also higher than that in the maternal side of the placenta. A similar iodine concentration (PIC and PIS) appeared in the group II and AI group. We speculate that the reason for this phenomenon is that when iodine is deficient, to prevent fetal iodine deficiency, the expression of the NIS and Pendrin increase compensatorily, prompting more iodine in the mother’s body which is transported to the placenta. However, when iodine intake was above requirements, the expression of NIS on the maternal side of the placenta in the ARI group was significantly higher than that in the AI group. Huika et al. (Reference Li, Richard and McKinnon22) performed in vitro experiments on human choriocarcinoma BeWo cells as a human trophoblast cell model and concluded that incubation with iodide inhibited the expression of NIS mRNA and protein in BeWo cells, but it seemed to not affect Pendrin. When suggesting iodine excess, the expression of NIS in the placenta may be reduced to protect the fetus from the effects of iodine excess(Reference Li, Richard and McKinnon22). However, a more direct human study on the regulation of iodine uptake by the placenta iodine transporter under conditions of iodine excess has not been reported. Current studies in the rat thyroid generally agree that NIS expression is significantly reduced when iodine intake is excessive(Reference Serrano-Nascimento, Salgueiro and Pantaleão23,Reference Liang, Feng and Lin24) . In this study, the average UIC and SIC of the ARI group with high iodine content were 386·60 μg/l and 104·76 μg/l, respectively. The WHO defined iodine excess as UIC greater than 500 μg/l(25). Decreased NIS expression occurred only at multiples to tens of multiples above appropriate iodine in rat and mouse studies(Reference Chen, Lin and Yang26,Reference Hussein Ael, Abbas and El Wakil27) .
Since there is no functioning fetal thyroid tissue during the first 13–15 weeks of pregnancy, iodine is not required by the fetus. TH-related psychoneurological development in the first trimester is entirely dependent on maternal hormone production(Reference Delange28). Exchange of molecules between maternal and fetal circulations occur by transport through the plasma membrane and cytoplasm of the trophoblast cells(Reference Jauniaux, Jurkovic and Campbell29). Currently, only one study has focused on differences in the concentrations of triiodothyronine (T3), thyroxine (T4) and trans-T3 on the maternal and fetal sides of the human placenta(Reference Peng, Li and Xie14). The levels of F3 and T4 on the fetal side were significantly lower than those on the maternal side, indicating that the fetal side of the placenta made a fundamental contribution to the limitation of deiodination of iodothyronines(Reference Peng, Li and Xie14). However, the results of this study showed that the concentrations of FT3 and FT4 on the fetal side were slightly lower than those on the maternal side, but the difference was not statistically significant. We think it may be because we are detecting FT3 and FT4 instead of T3 and T4, and the proportions of T3 and FT3, T4 and FT4 in the placenta are inconsistent.
For the first time, we focused on the association of TSH, FT3 and FT4 in serum and placenta. There was a positive correlation between mFT3 and fFT3 (r = 0·44, P = 0·0067). mTSH was positively correlated with fTSH (r = 0·75, P < 0·001, Fig. 5(b)). However, there was no correlation between serum and placenta FT3, FT4, and TSH. We speculate that the transport of TH from serum to placenta mainly relies on active transport by hormone transporters, while FT3 and TSH may be passive transporters in the fetal and maternal sides of the placenta. Seven TH transporters have been reported to be expressed in the placenta. These include monocarboxylate transporters 8 and 10 (MCT8, MCT10), l-amino acid transporters 1 and 2 (LAT1, LAT2), organic anion transporting polypeptides 1A2 and 4A1 (OATP1A2, OATP4A1), and TH-binding protein transthyretin (TTR)(Reference James, Franklyn and Kilby30–Reference Landers, McKinnon and Li32). The mechanisms that mediate TH transport from maternal to fetal circulation remain largely unknown. We will further explore this in future studies.
Acknowledgements
None.
This work was funded by the National Natural Science Foundation of China (NSFC grant no. 81920108031 and NSFC Grant nos. 82230113).
W. Z., M. F. and W. G. contributed to the study’s conception and design. M. F., H. Z., Y. G. and Z. R. performed the experiments. M. F., Y. G. and H. Z performed the data analysis. M. F. and Y. G wrote the first draft of the manuscript. H. Z. helped to check the manuscript. All authors commented on previous versions of the manuscript. All authors read and approved the final manuscript.
The authors declare no conflicts of interest.
Supplementary material
For supplementary material/s referred to in this article, please visit https://doi.org/10.1017/S0007114524000084